Earth Is Drifting Away From The Sun, And So Are All The Planets
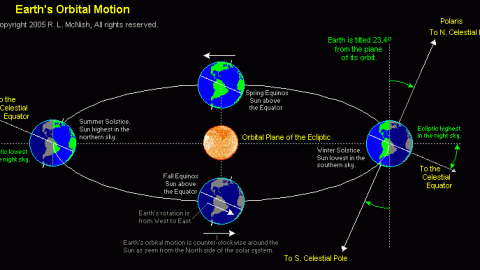
The reason why is simple, and should apply to every solar system like our own.
On January 3rd, 2019, Earth reached the point in its orbit where it’s at its closest approach to the Sun: perihelion. Every object orbiting a single mass (like our Sun) makes an ellipse, containing a point of closest approach that’s unique to that particular orbit, known as periapsis. For the past 4.5 billion years, Earth has orbited the Sun in an ellipse, just like all the other planets orbiting their stars in all the other mature solar systems throughout the galaxy and Universe.
But there’s something you may not expect or appreciate that nevertheless occurs: Earth’s orbital path doesn’t remain the same over time, but spirals outward. This year, 2019, our perihelion was 1.5 centimeters farther away than it was last year, which was more distant than the year before, etc. It’s not just Earth, either; every planet drifts away from its parent star. Here’s the science of why.
The force responsible for the orbits of every planet around every solar system in the Universe is the same: the universal law of gravitation. Whether you look at it in terms of Newton, where every mass attracts every other mass in the Universe, or in terms of Einstein, where mass-and-energy curves the fabric of spacetime through which other masses travel, the largest mass dominates the orbit of everything it influences.
If the central mass were unchanging, and were the only factor at play, the force of gravity would remain constant over time. Every orbit would continue in a perfect, closed ellipse forever, and would never change.
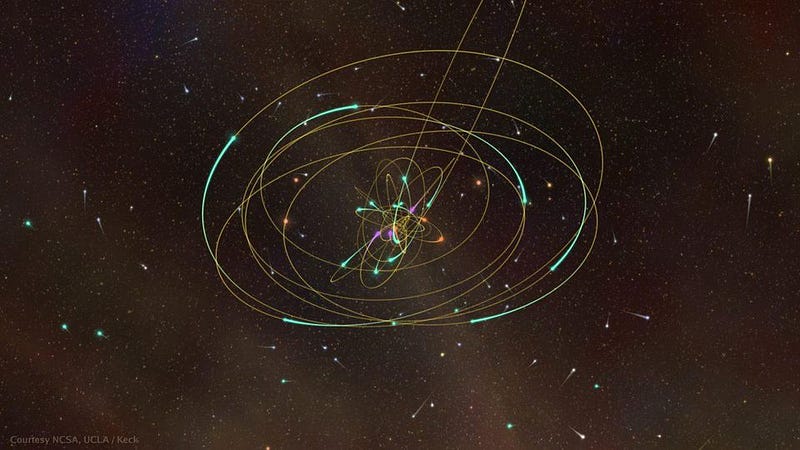
Of course, that’s not what happens. There are other masses present in every solar system: planets, moons, asteroids, centaurs, Kuiper belt objects, satellites and more. These masses serve to perturb the orbits, causing them to precess. This means that the point of closest approach — periapsis in general or perihelion for an orbit with respect to our Sun — rotates over time.
Orbital mechanics, in a variety of fashions, affect the precession of equinoxes. The Earth, for example, had its perihelion and the December solstice aligned just 800 years ago, but they are slowly drifting apart. With a period of 21,000 years, our perihelion precesses in such a way that it alters not only the point of closest approach in our orbit, but the location of our pole stars.
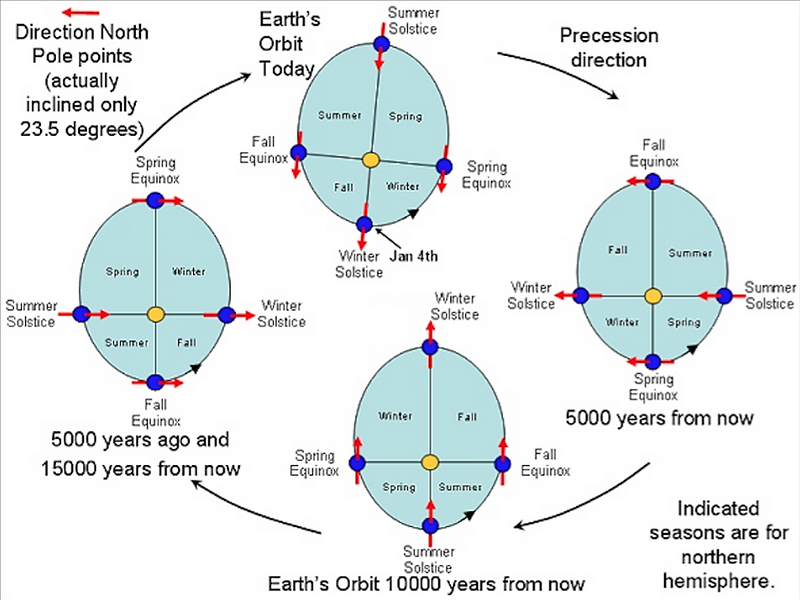
There are other factors that alter our orbit as well, including:
- the additional curvature of spacetime due to General Relativity, which causes planets near to a large mass to undergo additional precession,
- the presence of matter particles in the plane of the Solar System, which causes drag on the planets and creates an inspiraling phenomenon,
- and the creation of gravitational waves, which is what happens when any mass (like a planet) passes through a region where spacetime curvature is changing (such as near a star), also causing an inspiral.
These latter two effects, however, are only important under extreme conditions, such as very close to a large, compact mass, or in the early stages of a solar system’s formation, when protoplanetary disks are present and still massive.

Today, Earth (and all of the planets) are so far from the Sun and surrounded by such a sparse amount of matter that an inspiral timescale is trillions-to-quadrillions of times longer than the present age of the Universe. Since the protoplanetary disk evaporated away entirely some 4.5 billion years ago, there is almost nothing left to dissipate our angular momentum. The largest effect contributing to our inspiral is the emission of the solar wind, i.e., particles from the Sun, which smack into our planet and stick, causing us to lose a little bit of angular momentum.
Overall, the Earth isn’t even spiraling in toward the Sun; it’s spiraling outward, away from it. So are all the planets of the Solar System. With every year that goes by, we find ourselves just slightly — 1.5 centimeters, or 0.00000000001% the Earth-Sun distance — farther away from the Sun than the year before.
The reason why is due to the Sun itself.
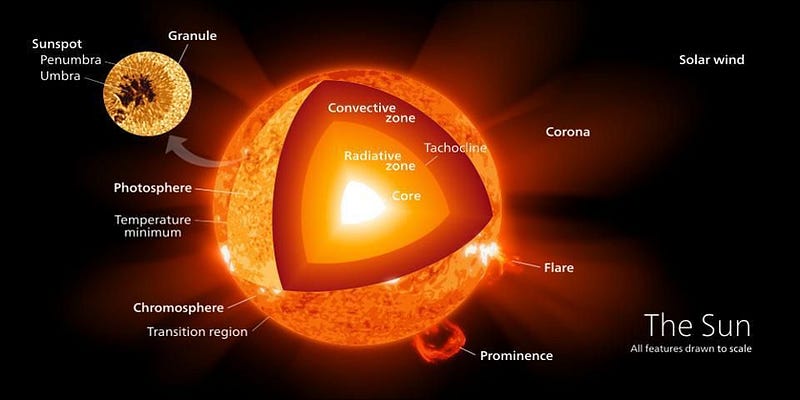
Deep inside the Sun, the process of nuclear fusion occurs. Every second, the Sun emits some 3.846 × 10²⁶ joules of energy, which are released via the conversion of mass into energy in the core. Einstein’s E = mc² is the root cause, nuclear fusion is the process, and the continuous emission of energy from the Sun is the result. This energy is the underlying process that powers practically every biologically interesting process occurring on Earth.
But what’s underappreciated is that, over time, the conversion of matter into energy results in the Sun losing a considerable amount of mass. Over the 4.5 billion year history of the Solar System, our Sun, due to the process of nuclear fusion, has lost approximately 0.03% of its original mass: comparable to the mass of Saturn.

On a year-by-year basis, the Sun loses some 4.7 million tons of matter, which lessens the gravitational pull on every object in our Solar System. It’s this gravitational pull that causes our orbits to behave as we know them to behave.
If the pull had remained unchanged, there would be a very, very slow inward spiral due to the effects of friction, collisions, and gravitational radiation. But with the changes we actually experience, the Earth, like all planets, is compelled to slowly drift away and spiral outwards from the Sun. Although the effect is small, this change of 1.5 centimeters per year is easily calculable and is unambiguous.
What we haven’t been able to do is measure that change in distance directly, however. We know it must occur; we know what its magnitude must be; we know that we’re spiraling away from the Sun; we know that this is happening to all the planets.
But what we’d love to do is measure it, directly, as yet another test of the laws of physics as we know them. That’s how physics advances:
- by predicting what we expect to observe based on all the knowledge we’ve accumulated and our best theories,
- by running an experiment/making an observation that measures the results of such a test to the required precision,
- and to compare what we see with what we expect.
When things line up, our theories are confirmed; when they don’t, it’s an indication that we might be on the cusp of a scientific revolution.
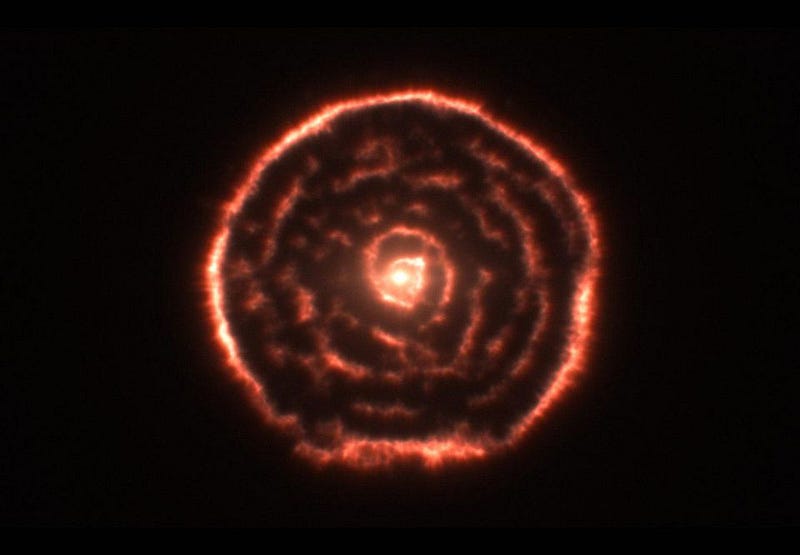
In the case of the Solar System, though, it would be a shock if the Earth and all the planets weren’t spiraling away from the Sun. The story of why we must be spiraling away from the Sun is so simple and compelling that it’s impossible to ignore.
The Sun outputs energy, which we observe, which allows us to calculate the rate of mass loss via Einstein’s E = mc².
The mass of the Sun, along with the orbital parameters of our planets, determines the path and shape of how they revolve around the Sun.
If we change that mass, the orbits change by an easily-calculable amount, even using straightforward Newtonian physics.
And when we do those calculations, we find that the Earth migrates away from the Sun at ~1.5 centimeters per year.
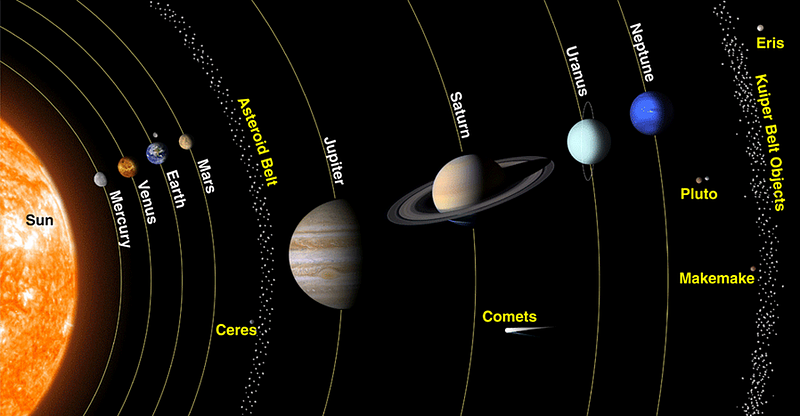
The Sun’s mass loss, by burning its nuclear fuel, ensures that every mass orbiting in our Solar System is slowly spiraling outward as time goes on. Some 4.5 billion years ago, our planet was around 50,000 kilometers closer to the Sun than it is today, and will grow more distant more rapidly as the Sun continues to evolve.
With each and every orbit that passes, the planets become progressively less tightly-bound to our Sun. The rate at which the Sun burns through its fuel is increasing, accelerating the rate at which all the planets spiral outwards. While this should never unbind any of the planets we have today, the slow, steady, outward migration of every world is inevitable.
We’re closer to the Sun, this year, than we’ll ever be again. This is true for every planet around every established star in the Universe, too, giving us one more reason to appreciate all that we have today.
Ethan Siegel is the author of Beyond the Galaxy and Treknology. You can pre-order his third book, currently in development: the Encyclopaedia Cosmologica.