Does dark matter exist? Or is gravity wrong?
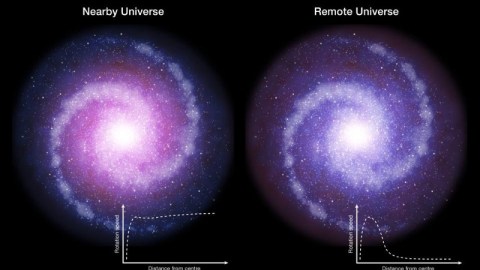
The answer lies billions of years in the past.
“Dark matter or invisible element?
You decide.” –Toba Beta
When we look out at the luminous matter in the Universe — stars, galaxies, clusters of galaxies and the hot gas in and between them — it tells a couple of different stories. One is the story of how normal matter (based on atomic nuclei and electrons) comes together to emit, absorb and otherwise interact with light: an indispensable part of how we see the Universe. But another story is that of gravitation. By observing how this matter moves relative to its surrounding environment, we can learn an awful lot about the gravitational interaction in the Universe. One of the greatest surprises that the 20th century had in store for astronomers was that if you look at the gravitational effects of these large structures, normal matter alone isn’t enough to account for it.
If you measure the individual velocities of galaxies within a large galaxy cluster, such as the Coma Cluster (above), you can infer how much mass must be present in order to keep the cluster from flying apart. That number is not only about 50 times greater than the amount of stars that are present, but approximately a factor of six larger than all the stars, planets, gas, dust, plasma, and all other forms of normal matter combined. There would seem to be but two simple options that exist as solutions to this: either there’s a new, unseen form of mass that’s present, dark matter, or the laws of gravity on the largest scales depart from the predictions of Einstein’s General Relativity, some form of modified gravity.
A very similar effect shows up when we look at individual galaxies. If you look at the speeds of the stars rotating close to a galaxy’s center, you find that they’re consistent with orbiting at speeds given by the normal matter in the galaxy’s core. But as you move farther out, the speeds of the more distant stars doesn’t drop off the way you’d expect if normal matter were responsible for the galaxy’s gravity. In our Solar System, Mercury orbits more quickly than Neptune because the Sun dominates our gravitational field; in a galaxy, you expect that mass will follow where the stars, gas, dust, plasma, and the rest of the normal matter is. But it doesn’t.
Again, the same two explanations could, in principle, explain the discrepancy. If the Universe is filled with dark matter, a form of matter that only interacts gravitationally, but that is invisible to both light and normal matter, that additional mass would have fallen into a massive, diffuse halo surrounding each galaxy by this point. If instead, the Universe obeys a different gravitational law than General Relativity, that modified law of gravity should be affect galaxies the same way — based on accelerations below a certain scale — regardless of the size of the galaxy in question.
Although there are attempts to directly detect dark matter, and there are similarly attempts to look for different effects on smaller astrophysical scales that would show a departure from Einstein’s General Relativity, both of these efforts have come up empty. However, there’s a brilliant way from a purely astrophysical perspective to distinguish between these two ideas: look at the rotation curves of galaxies from billions of years ago.
If the laws of gravity truly do depart from Einstein’s relativity, then they should exhibit that departure in a consistent way at all times throughout our cosmic history. A galaxy today should obey the same underlying laws as a galaxy three, five or ten billion years ago. On the other hand, a Universe with dark matter in it should exhibit two different evolutionary effects:
- Powerful bursts of star formation should impart large amounts of energy to the normal (but not the dark) matter, expelling some of the normal matter (but keeping all of the dark matter), especially in smaller, lower-mass galaxies.
- Younger galaxies should have had less dark matter fall into them, and should exhibit lower densities of dark matter if we can observe their rotations at earlier times.
The first of these effects has been noted for many years: dwarf galaxies are dominated by dark matter to an even greater degree than large, spiral galaxies. Unfortunately, that effect alone isn’t enough to discriminate between dark matter and modified gravity, since the same acceleration law (known as MOND) describes those systems as well. But technology and techniques are finally progressing to the point where rotation curves of distant, young galaxies can begin to be measured. For the younger galaxies, we’d expect that there would be less dark matter in the luminous parts of these galaxies, meaning that we’d expect the stars closer to the galactic outskirts to rotate more slowly than their modern counterparts.
In a new paper published in Nature, lead author Reinhard Genzel claims to have discovered exactly that. By examining six independent, bright galaxies, Genzel claims to have discovered exactly this effect: more distant galaxies rotate more slowly at their outskirts than in their center. It would seem that dark matter has scored a major victory!
And it has, but not for the reason that Genzel claims. You see, if you look at the six individual galaxies that Genzel claims as evidence, they don’t show a substantial effect to support this idea. The rotation curves are completely consistent with being flat, and more importantly, correlate with surface brightness just as local galaxies do, as MOND-advocate Stacy McGaugh points out.
However, the same group has used the same technique to study many more than just six galaxies; they’ve studied a total of 101! When they use a technique called “stacking” — where they calibrate each galaxy to one another to examine their overall, average properties — they find that there is, in fact, a precipitous drop-off in rotational velocity as you move away from the center of these galaxies.
This is, remarkably, a strong piece of evidence that points to dark matter and not to modified gravity! As Philipp Lang and his coauthors write in a paper just submitted to the Astrophysical Journal:
Our stacked rotation curve exhibits a decrease in rotation velocity beyond the turn-over radius down to ∼ 62% of the maximum normalized velocity Vmax, confirming the drop […] as a representative feature for our sample of high-z disk galaxies. The drop seen in our stacked rotation curve strikingly deviates from the average rotation curves of local spirals at the same mass at > 3σ significance level.
As you can see from their attempts to fit various dark matter (and no dark matter) models to this data, there’s still very good evidence for dark matter, it’s merely at a different stage of galactic evolution.
If this result holds up with more and better data, this may provide a window into galactic evolution that finally allows us to discriminate between dark matter and modified gravity in a clear and robust way. These types of observations, to measure the rotation curves of galaxies many of billions of light years away, will be a prime science goal for new telescopes in the 2020s like GMT, E-ELT and WFIRST. Both sides will continue to argue for their interpretation of the data, but in the end, it will be the full suite of data that reveals how nature truly behaves. Will Einstein be superseded? Or will we all wind up joining the dark side? By time another decade goes by, the answer may finally be known.
This post first appeared at Forbes, and is brought to you ad-free by our Patreon supporters. Comment on our forum, & buy our first book: Beyond The Galaxy!