Did LIGO Just Discover Two Fundamentally Different Types Of Neutron Star Mergers?

LIGO just announced the second neutron star-neutron star merger ever seen in gravitational waves. It doesn’t match the first.
On August 17, 2017, an event occurred that forever changed how we viewed our Universe: two neutron stars were caught merging together in a galaxy just 130 million light-years away. Its gravitational waves arrived in the LIGO and Virgo gravitational wave detectors over a timespan of just a few seconds, followed by a spectacular burst of high-energy radiation seen by NASA’s Fermi satellite.
Over the following weeks and months, follow-up observations across the electromagnetic spectrum revealed that two neutron stars had merged, leading eventually to a black hole after creating and ejecting an extraordinary amount of heavy elements. At last, we understood the cosmic origin of gold, mercury, tungsten and more. Two years later, on April 25, 2019, gravitational wave detectors saw their second neutron star-neutron star merger, but no electromagnetic signals were seen at all. The reason, excitingly enough, might be that there are two fundamentally different classes of binary neutron star mergers. Here’s how.
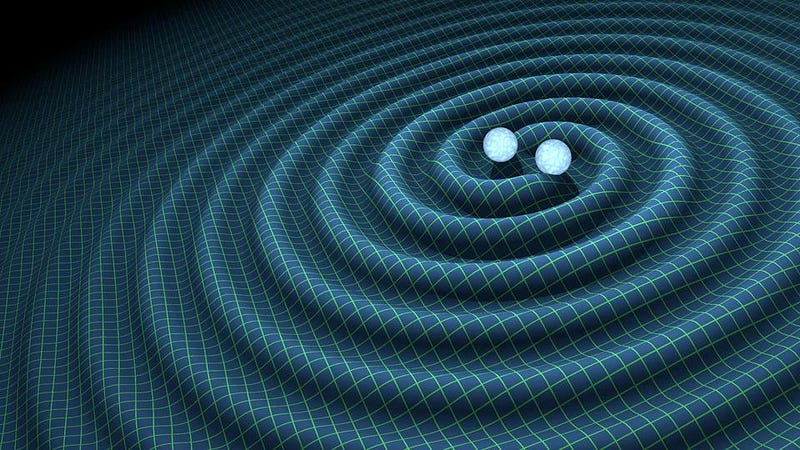
On April 1, 2019, the LIGO and Virgo gravitational wave observatories began operating for their third data-taking run, after discovering a total of 13 cumulative events in approximately 400 days of observing in all prior runs. The detectors had all been upgraded since that time, and as of early January, approximately 43 additional events have been seen in just 250 days of observing time, a clear indicator of how LIGO and Virgo have become more sensitive: to wider mass ranges and events at greater distances.
The first neutron star-neutron star merger had a slew of fascinating properties, but one thing that stands out is how remarkably close it was: just 130 million light-years away, close to the limits of what the LIGO detectors could see prior to the upgrade. The second such neutron star-neutron star merger event, seen less than 4 weeks after the start of the third data-taking run, was approximately four times as distant, occurring an estimated 518 million light-years away. The signal was too distant and faint to be seen by the Virgo detector, and just by chance only one of the LIGO detectors was operational at the time.
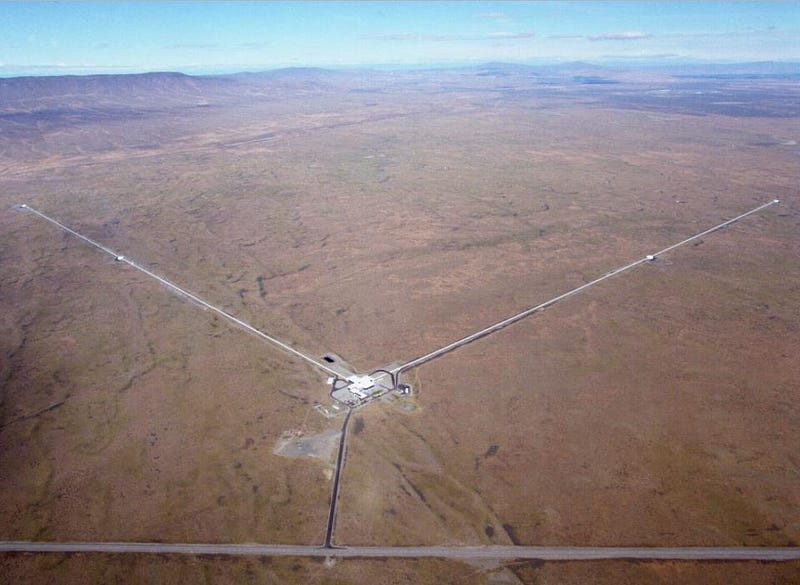
Still, the April 25, 2019 signal that showed up in the LIGO Livingston detector — the one that was online at the time — was extremely strong, achieving a detection signal-to-noise significance of 12.9, where 5 is the “gold standard” for a robust detection. The form of the signal was incredibly analogous to what was seen back on August 17, 2019 in both LIGO detectors, but had an inherently greater amplitude, indicating a higher set of masses for both neutron stars, as well as a higher combined mass.
Whereas the first binary neutron star merger had a combined mass of approximately 2.7-to-2.8 solar masses, the second one was significantly heavier, with a combined mass of 3.4 solar masses. The 2017 event, where two neutron stars merged together, appeared to show evidence of initially forming a single, rapidly rotating neutron star for a few hundred milliseconds, before the entire system collapsed to a black hole. However, the 2019 event was well above the mass limit where neutron stars are theoretically allowed. At a combined 3.4 solar masses, this neutron star merger should have formed a black hole directly.
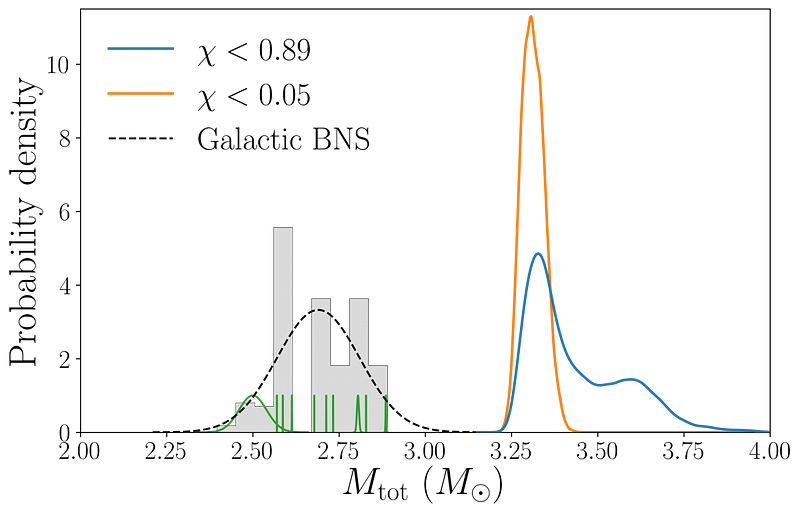
Does this mean that there are fundamental differences between the types of neutron star mergers that occur with low combined masses, where it’s possible to form a neutron star in the immediate aftermath, and the heavier neutron star mergers that lead directly to black holes? It’s an intriguing idea, and one that appears to be supported by the fact that neither the official NASA Fermi collaboration nor the ESA INTEGRAL collaboration saw a gamma ray signal: the type of signal that should have arrived within seconds of the merger appearing in our gravitational wave detectors.
The lack of such a signal appears, on its surface, to suggest something absolutely remarkable. Perhaps lower-mass neutron star mergers produce gamma rays, ejecta, the Universe’s heaviest elements, and a multi-wavelength, long-lasting afterglow. And perhaps, above a certain mass threshold, higher-mass neutron star mergers simply interact and go directly to a black hole, swallowing up all of the matter associated with both stars, producing no heavy elements and emitting no further observable signal at all.
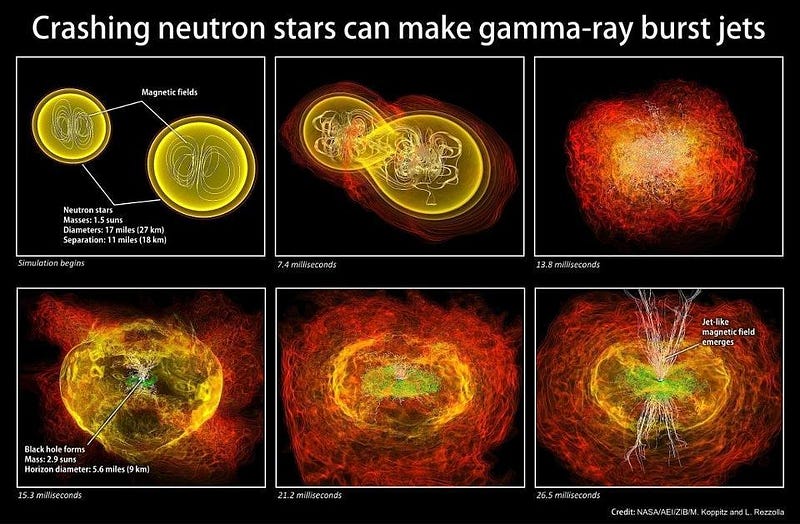
This is an eminent possibility from a theoretical perspective. If two neutron stars merge together and don’t immediately create an event horizon, an enormous, runaway fusion reaction will begin to occur. The high-energy processes at play will produce a fast gamma ray signal, while approximately 5% of the total mass of the neutron stars will get ejected back into the interstellar medium, enriching its host galaxy and providing the origin of the heaviest elements of all, along with a long-lasting afterglow. Even if that resultant neutron star quickly collapses down to a black hole, which rapidly spinning neutron stars above about 2.5 solar masses are expected to do, the critical signals, light, and material have already escaped.
However, if they do immediately create an event horizon, the material that takes part in the neutron star-neutron star merger could all get swallowed by the expanding event horizon. Without any internal pressure coming from the merger’s core, there’s nothing holding the material outside the horizon up, and it could all collapse before any signal departed at all.
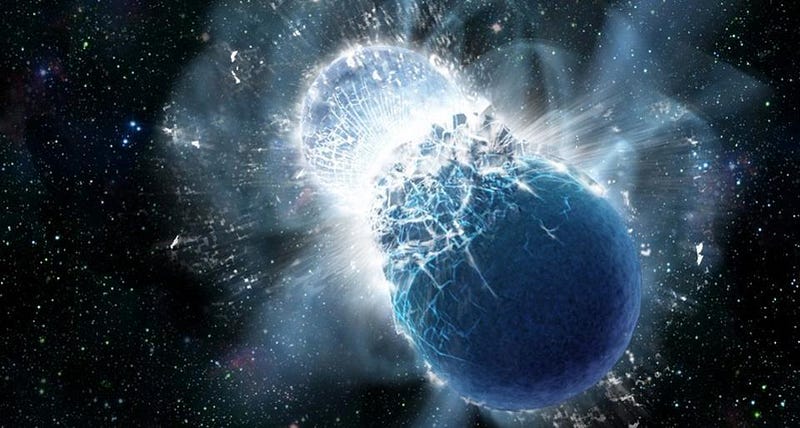
The scenario that two fundamentally different types of neutron star-neutron star mergers exist — separated by a direct-to-black-hole mass threshold — is a viable, intriguing possibility.
However, it’s not a foregone conclusion at all.
If you were to take the same gamma ray signal that was emitted by the 2017 neutron star-neutron star merger and to place it at the distance of this latest neutron star-neutron star merger, it would be approximately a factor of 16 weaker by the time it arrived on Earth, as signals get fainter with the square of the distance: something 4 times as distant appears just 1/16th as bright. The gamma-ray signal seen by NASA’s Fermi in 2017 was faint and weak, so much so that if it were reduced to 1/16th of what it actually was, it would have been a completely unobservable signature.
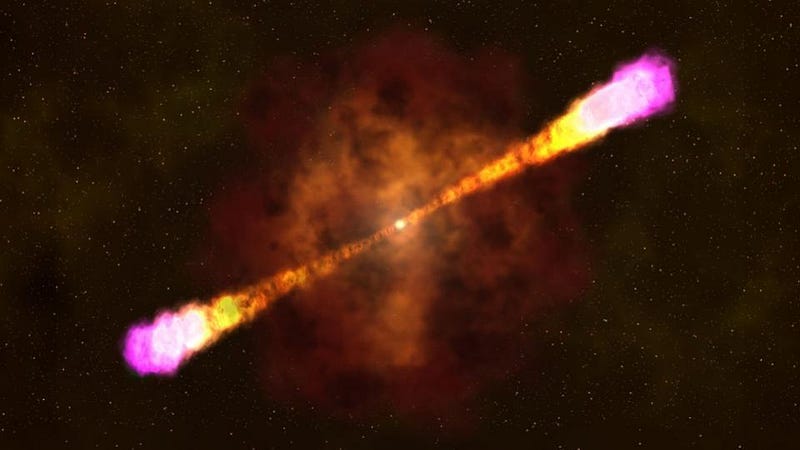
However, we’ve seen short-period gamma ray bursts — at least some of which are caused by merging neutron stars — out to much greater distances than either of these observed neutron star-neutron star mergers. The reason that the first observed merger was so weak may have been due to the orientation of the merger relative to our line-of-sight, which can change the observed brightness by approximately a factor of 100 between the most favorable and least favorable configurations. The second merger could also have similarly had an unfavorable configuration, creating a burst that was simply below our detection threshold.
Both the NASA Fermi and ESA INTEGRAL instrument teams, which are the two space-based gamma ray observatories that ought to be sensitive to the type of signal that would be produced in merging neutron star events, reported no statistically significant signal in their data. They saw no signs of any transient signals that could be associated in both space and time with the gravitational wave signal seen by LIGO Livingston.
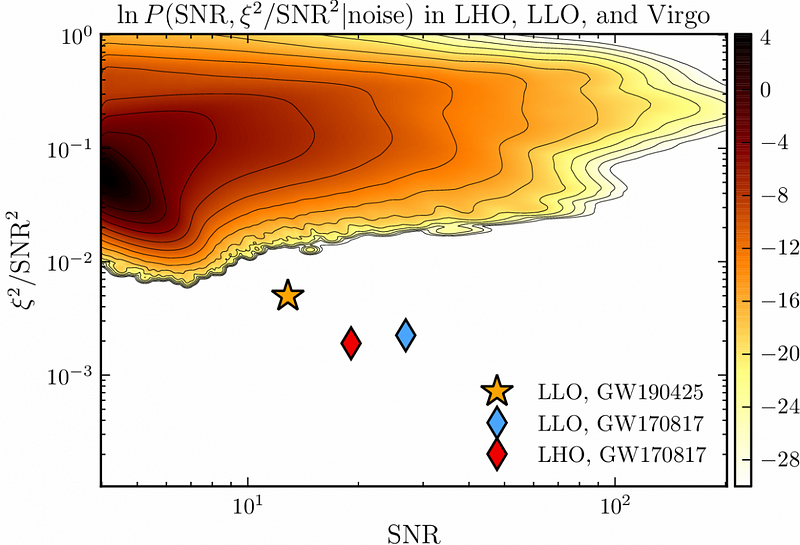
However, an independent team did their own analysis using the ESA INTEGRAL data from that time, and claims to find weak evidence for a signal in the data after all: one that could possibly be correlated with the gravitational wave event. Their claim has been met by skepticism from the scientific community, as:
- they see two bursts separated by about 5 seconds, rather than the expected (and previously recorded) single burst,
- each burst, independently, is not statistically significant on its own,
- and, as outsiders who are not a part of the ESA INTEGRAL team, they do not have the same experience as INTEGRAL team members in analyzing, calibrating, and interpreting the data.
There are many infamous instances of collaboration outsiders drawing incorrect conclusions from a collaboration’s data because of analysis, calibration, and interpretation mistakes, and few have been convinced by this team’s arguments so far.
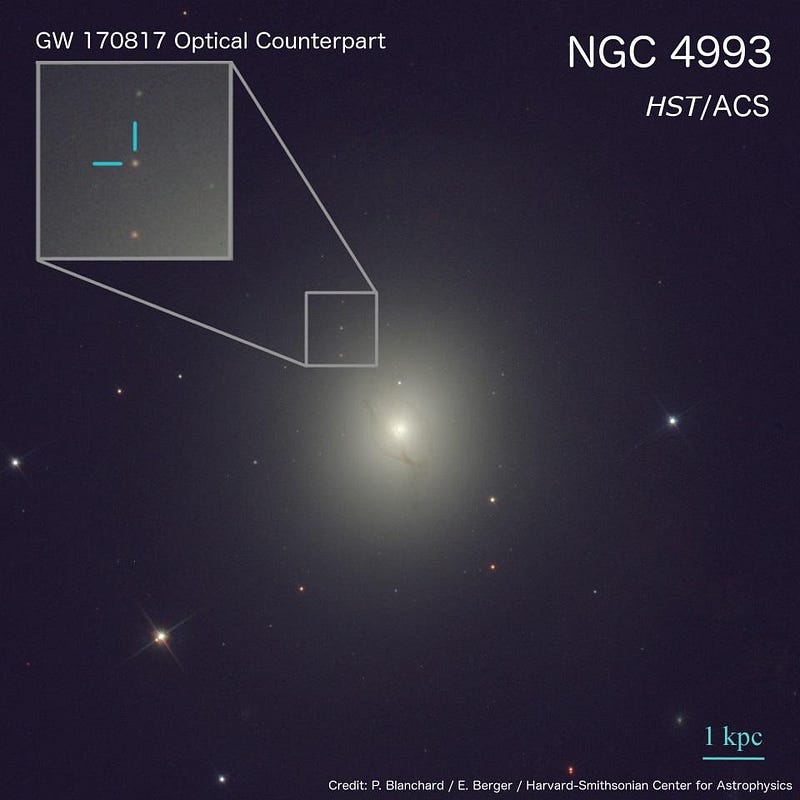
One of the difficulties in knowing what the true nature of this latest neutron star-neutron star merger was articulated by Dr. Katerina Chatziioannou at the most recent meeting of the American Astronomical Society. Because this event was only detected by the LIGO Livingston detector, with complementary data but no robust signal from the Virgo detector, achieving a good sky localization is impossible.
The first neutron star-neutron star merger from 2017 had data from all three detectors, including a robust detection from both LIGO Hanford and LIGO Livingston, and the gravitational wave signal was restricted to an area of just 28 square degrees: 0.07% of the entire sky. Owing mostly to the lack of LIGO Hanford data, the second neutron star-neutron star merger could have occurred anywhere over an area of 8,284 square degrees, or about 20.7% of the sky. Without knowing where to point our telescopes, follow-up observations attempting to find an electromagnetic counterpart are all but guaranteed to be fruitless.
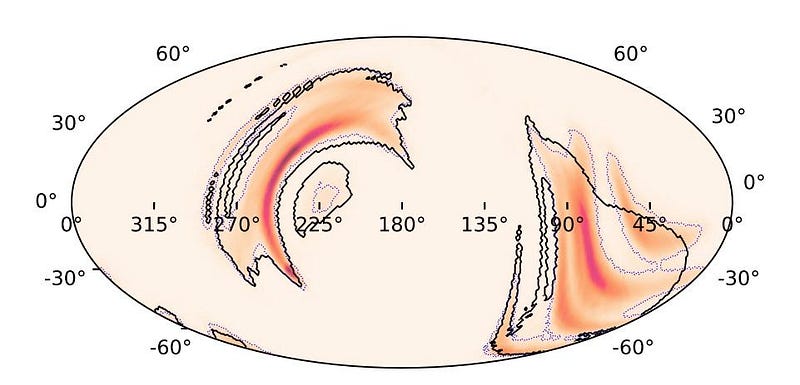
The first neutron star-neutron star merger ever directly observed was seen in both gravitational waves and in various forms of light, giving us a window into the nature of short gamma ray bursts, kilonovae, and the origin of the heaviest elements of all. The second one, however, had no robustly confirmed electromagnetic counterpart at all. The only major physical differences were the combined mass (2.74 vs. 3.4 solar masses), the initial object formed (neutron star vs. black hole), and the distance to the event (130 vs. 518 million light-years).
It’s possible that there really was an electromagnetic counterpart, and we simply weren’t able to see it. However, it’s also possible that binary neutron star mergers that directly lead to a black hole don’t produce electromagnetic signatures or enriched, heavy elements at all. It’s possible that this binary neutron star system, the most massive one ever discovered to date, represents a fundamentally different class of objects than have ever been seen before. This incredible idea should get put to the test over the next few years, as gravitational wave detectors continue to find more and more of these mergers. If there are two different classes of neutron star mergers, LIGO and Virgo will lead us to that conclusion, but we have to wait for the scientific data to know for sure.
Ethan Siegel is the author of Beyond the Galaxy and Treknology. You can pre-order his third book, currently in development: the Encyclopaedia Cosmologica.