Dark matter in galaxies: proven!
Either there’s an unseen source of mass, or the laws of gravity are wrong. But only one can explain what we see.
“The discrepancy between what was expected and what has been observed has grown over the years, and we’re straining harder and harder to fill the gap.” –Jeremiah Ostriker
Take a look out at the galaxies of the Universe, and you’ll surely notice one thing: they come in two main classes, grand spirals and giant ellipticals.
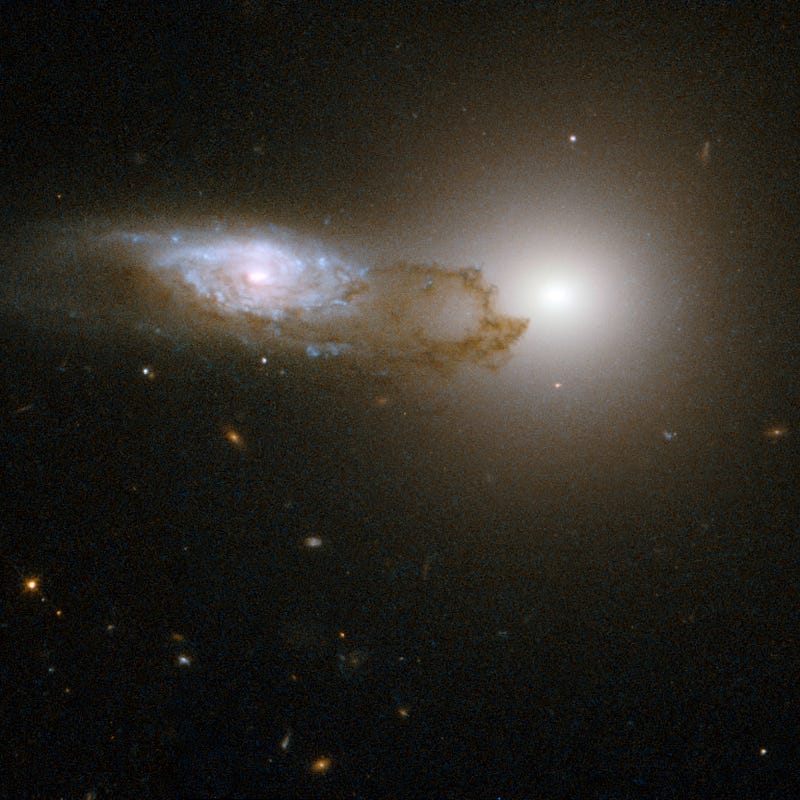
In all cases, these galaxies are made up of huge numbers of stars: hundreds of billions in the case of our Milky Way, but often many trillions in the largest elliptical galaxies.
Since we know how stars work, how their brightness, color, spectra and other intrinsic properties are correlated, all we need to do is measure all the light coming from one of these galaxies, and we know how much of their mass is in the form of stars.
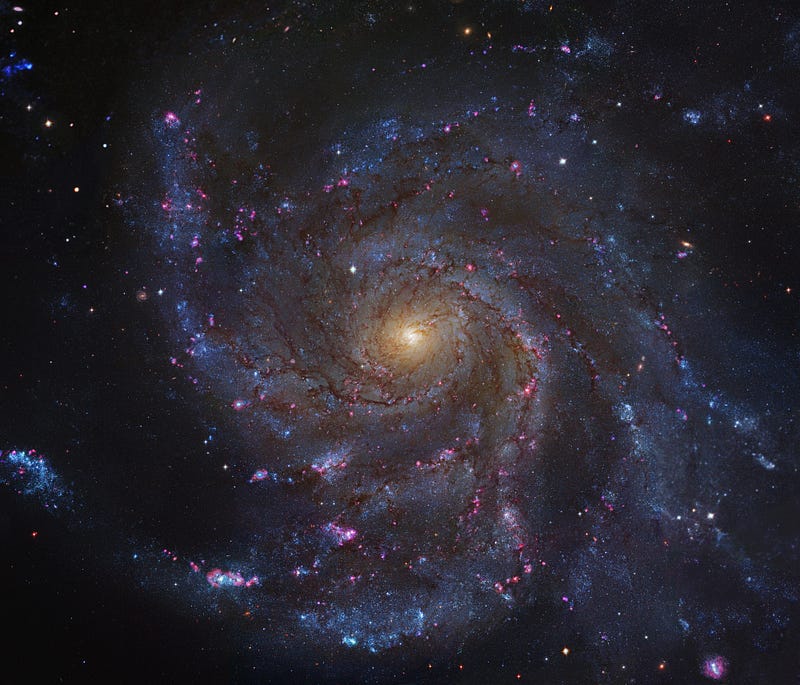
If a galaxy is oriented face-on to us — like the Pinwheel galaxy is, above — we can’t quite measure how quickly the stars are moving within it.
This would be an interesting measurement to make, you realize, because stars moving within a galaxy obey the laws of gravity, which are incredibly well-known. So if you make a measurement of how quickly the stars within it are moving, you can infer how much mass — and where its located — are inside.
Thankfully, most galaxies aren’t oriented face-on to us at all, but rather at an angle, so that we can measure how quickly the rotational speeds of the stars inside are.
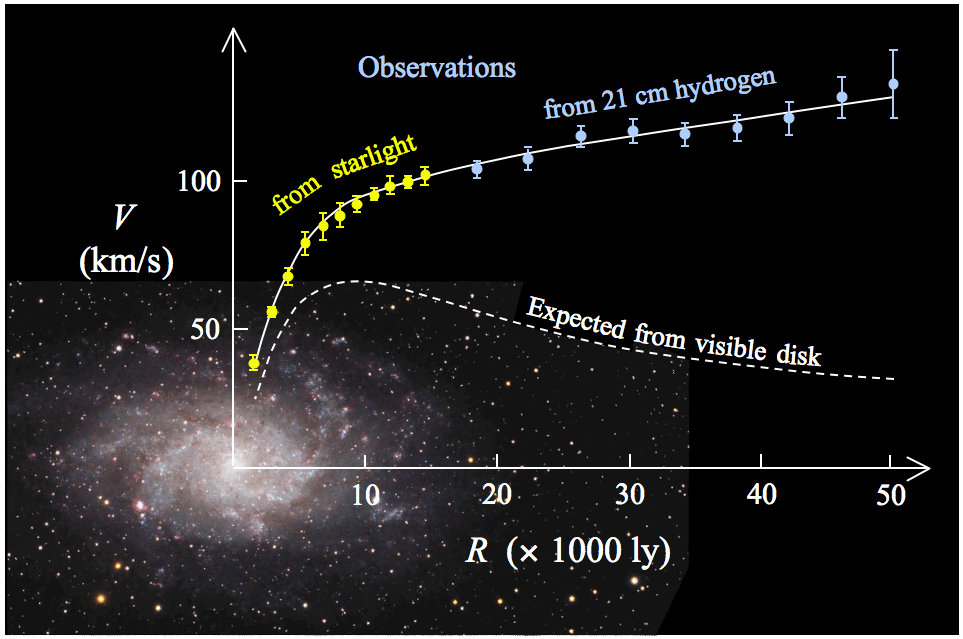
(In the case of ellipticals, we can use the velocity dispersions of the stars at various radii from the galactic center, which is also a quality measurement.)
What we notice when we make these measurements, quite shockingly, is that while the interiors of the galaxies are dominated by stars, there must be some additional type of mass present to account for the motions that we see. Not only that, but there must be appreciably more of it as we move farther and farther away from the galaxy’s center.
There were two (quite reasonable) potential solutions to this problem:
- The laws of gravity are problematic, and need to be modified on scales larger than the Solar System.
- Our understanding of matter is incomplete, and there must be a new type of matter present to account for what we observe.
The latter of these two possibilities is the idea of dark matter.
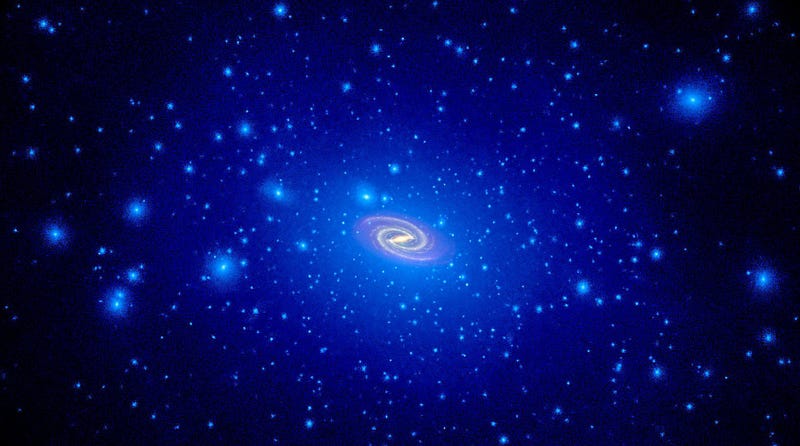
You might think, of course, that this “dark matter” is just normal stuff — protons, neutrons and electrons — that doesn’t emit light. I couldn’t fault you for that: we know of plenty of matter that does exactly this. Planets, you and I, dust, gas, and even ionized plasmas are all “normal matter” that doesn’t emit any visible light of its own.
And yet, if we look in all the different wavelengths of light that we know of, the wavelengths that are sensitive to these types of matter, as well as all the other signals we know of (such as microlensing, absorption lines, signatures of black holes, etc.), we find that there isn’t close to enough of it.
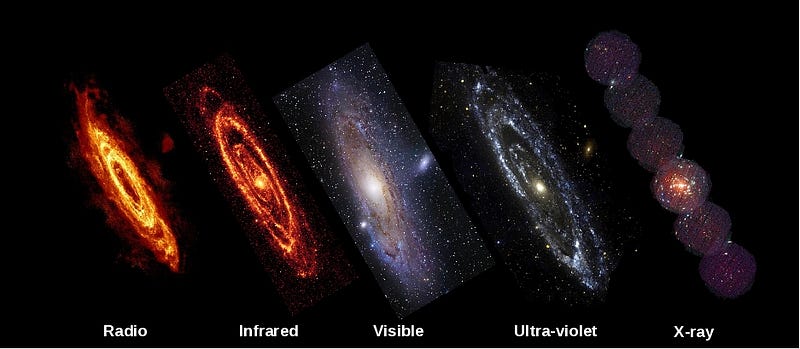
But if we look instead at gravitational lensing, or how much light gets bent, magnified and distorted by an intervening foreground galaxy, we can infer the total amount of mass present within the galaxy.
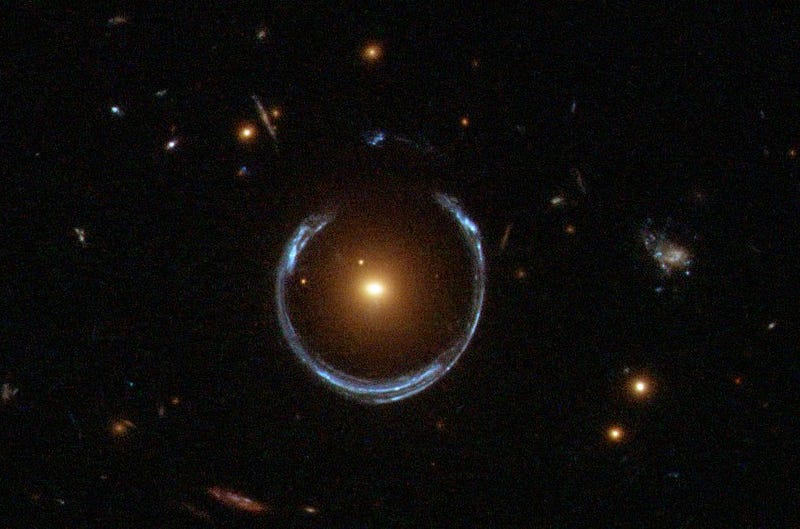
Based on what we can see, that same mismatch is always there: there’s significantly more total mass inside each and every galaxy that we measure than all the normal matter inside can account for.
But we could, in principle, just have the law of gravity wrong just as easily. What we’d want, ideally, is a way to do an experiment to test whether there’d be some way to separate the normal matter from the dark matter. This might sound impossible, but every once in a while, the Universe does us a favor, and two huge objects collide with one another at tremendously high speeds.
Imagine that there’s dark matter (in blue) and normal matter (in red) in both of these objects. When they collide, the normal matter — just like your hands “smack” together if you collide them — will interact, heating up, dissipating energy and slowing down. But the dark matter doesn’t interact (except gravitationally), so it simply passes right through to the other side.
The heated-up gas will emit X-rays, and the location of the X-rays will reveal where the normal matter (that isn’t in the form of stars) is located.
It’s a lot like imagining we have two guns pointed at one another.
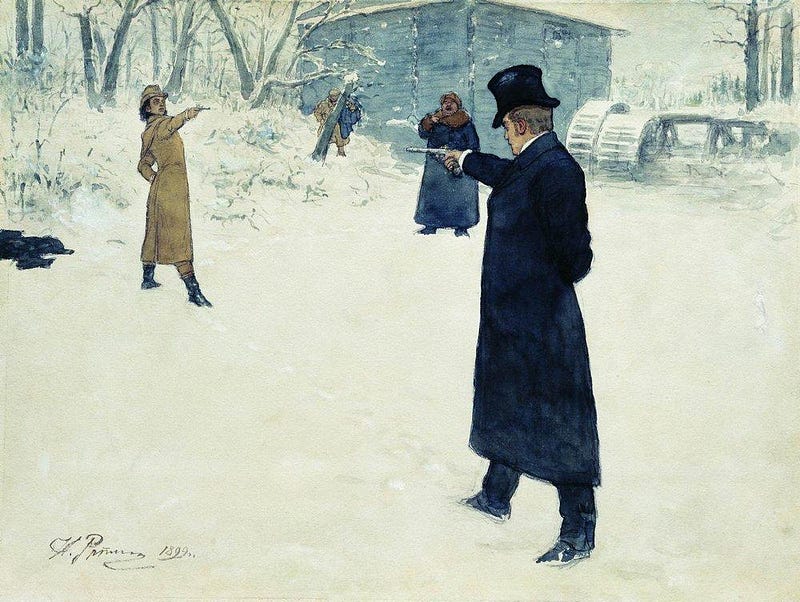
But instead of lethal bullets, each one is filled with some combination of:
- bird shot,
- foam, and
- some new type of material that can never collide,
all fired at one another. The pellets of bird shot, in pretty much all cases, will all miss one another. On rare occasion, you might get a collision, but that’s it. The foam, on the other hand, will always stick together if the shot is on-target. And the new material will always pass right through, whether the shot’s on-target or not.
How can you tell whether this new type of material is really there or not?
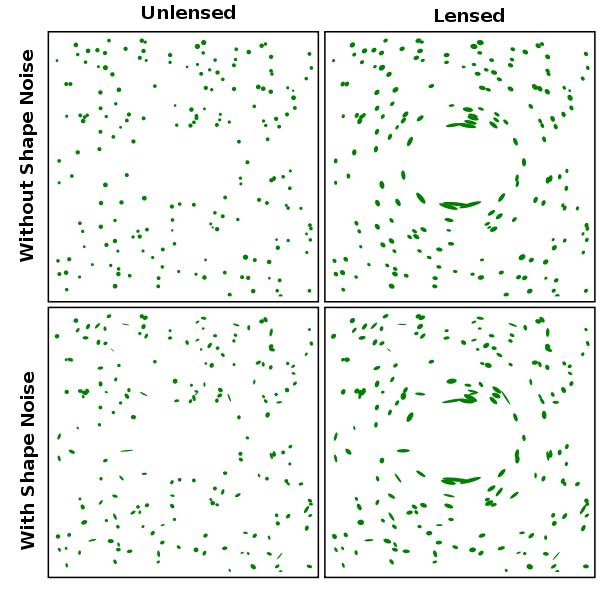
You use the phenomenon of gravitational lensing! While you might not have a perfect alignment or a super-dense clump to get those crazy lensed arcs or that insane magnification, you can still get weak gravitational lensing, which distorts the light from background sources (like galaxies) into particular elliptical patterns.
This tells you both the total mass inside as well as where it’s located, and has been successfully used to map the mass of various galaxies and clusters in the past.
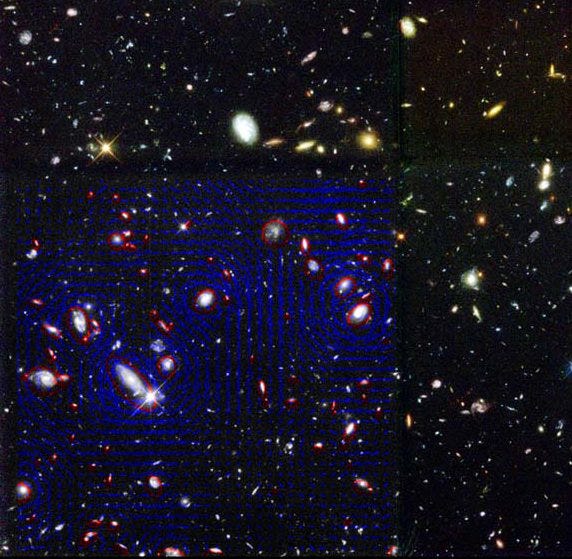
So that’s how we’d do it.
Well, we’ve actually discovered a significant number of giant structures — galaxy clusters — that are in the process of relatively high-speed collisions. Some have just undergone it, while others are in later phases of the collision, settling down into a more equilibrium state. In all cases, they’ve got images of the galaxies in the optical (the bird shot), a picture of the X-rays in pink (the foam), and a reconstruction of where the mass is (the non-colliding stuff) in blue.
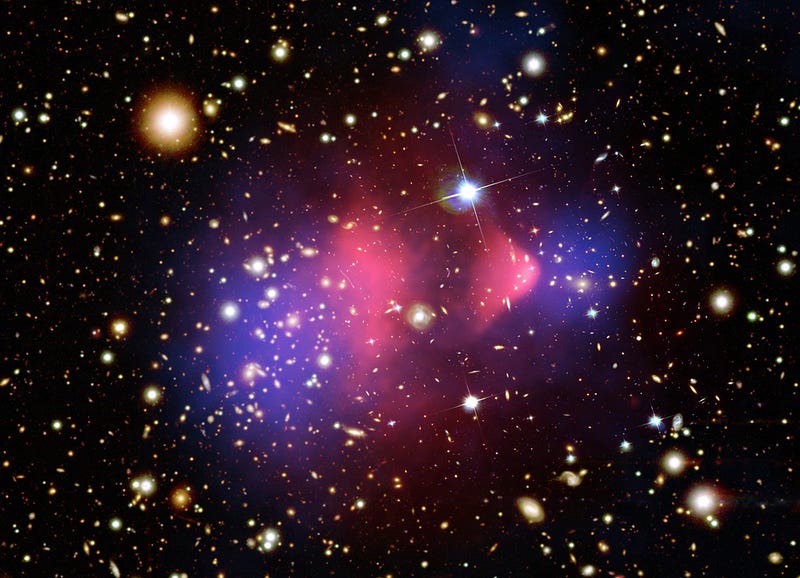
Lensing Map: NASA/STScI; ESO WFI; Magellan/U.Arizona/ D.Clowe et al.;
Optical: NASA/STScI; Magellan/U.Arizona/D.Clowe et al.
The first one discovered was the Bullet Cluster, which was back in 2006, which shows a clear separation of dark matter from the X-rays.
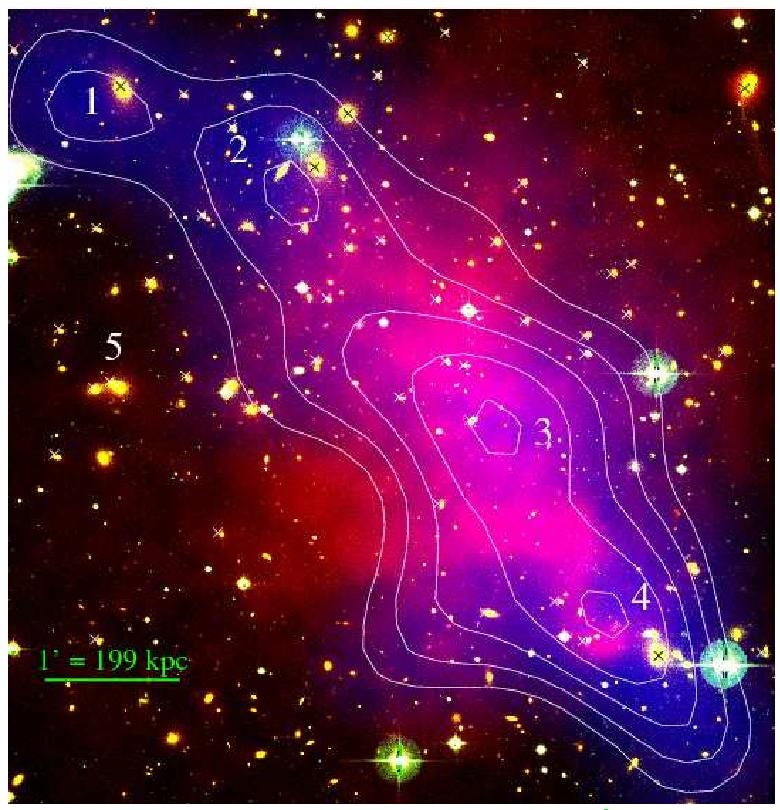
There’s the Trainwreck Cluster, Abell 520, which is in a much later phase.
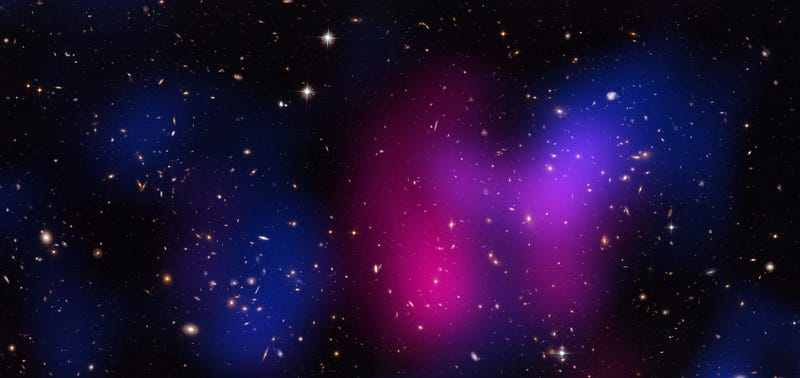
There’s the Musket Ball Cluster, a very high-speed collision that also shows a huge separation from X-rays and the matter.
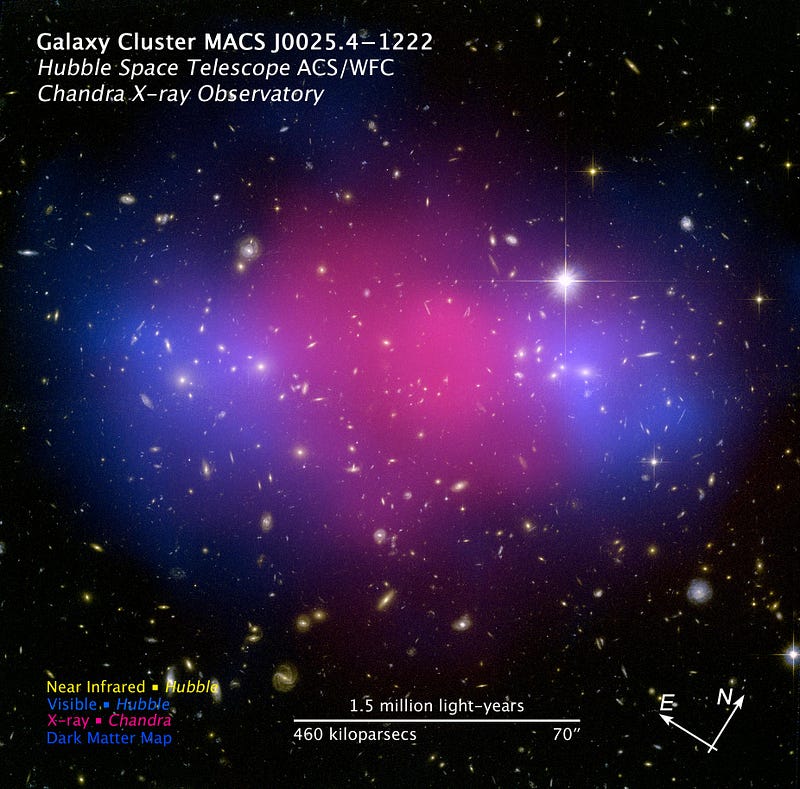
And there are two more recent interesting colliding clusters, that haven’t been given clever names, MACS J0025.4–1222 (above) and MACSJ0717 (below).
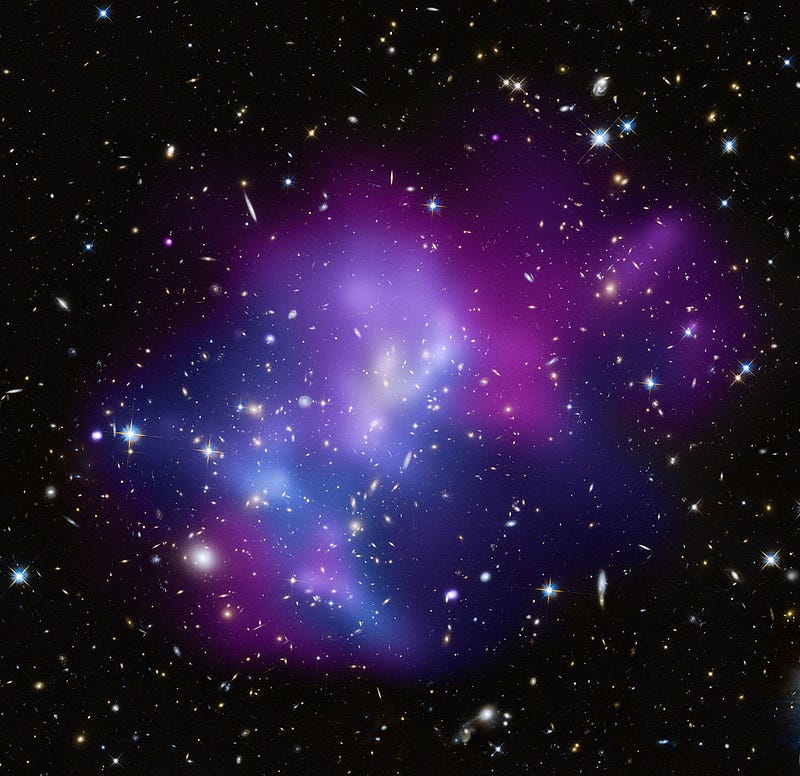
But these are huge collections of matter! Wouldn’t it be nice and clean if we could just have a single galaxy collide with another?
That might be too much to ask for, since the lensing signal would be almost imperceptible. But the Universe was kind enough to give us two very, very small galaxy groups — no bigger than our local group, which consists of our galaxy, Andromeda, and then maybe 40-to-50 tiny-pieces-of-crap galaxies (with less mass than Andromeda if you combined them all) — that slammed into one another at an incredibly high speed. The entire system, as you might expect, was dominated by just a handful of galaxies.
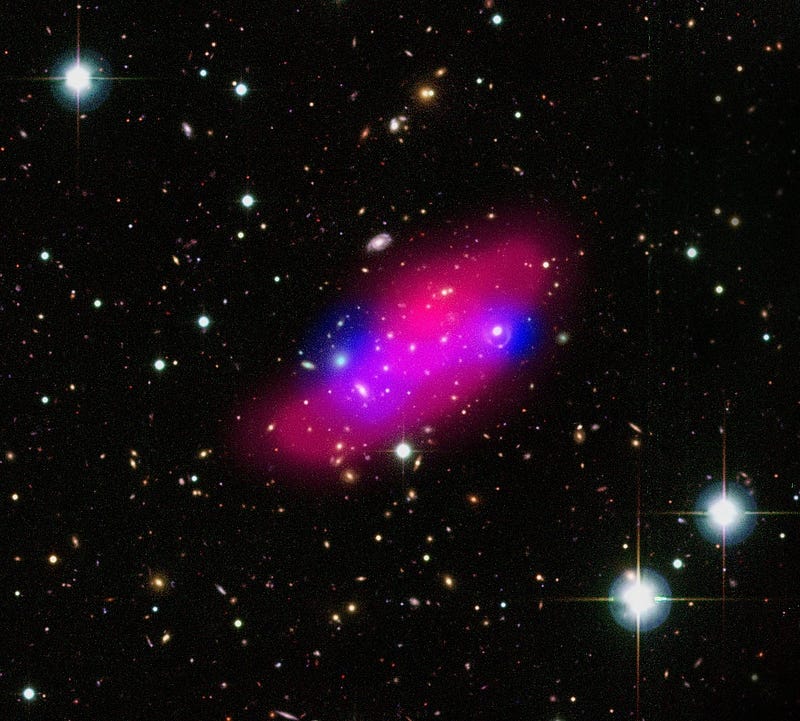
But that’s not where the majority of the normal matter — as evidenced by X-rays — was located at all! Say hello to the Bullet Group, SL2S J08544–0121. It was discovered, imaged and mass-reconstructed only a few months ago, which for the first time shows a huge discrepancy between where the normal matter and the mass is located in a structure this small!
We can zoom in, and highlight exactly where the individual galaxies are inside. Have a look at the blue and purple regions (where the mass by itself and the mass-and-gas overlap), and see how they compare to the red-and-purple regions.
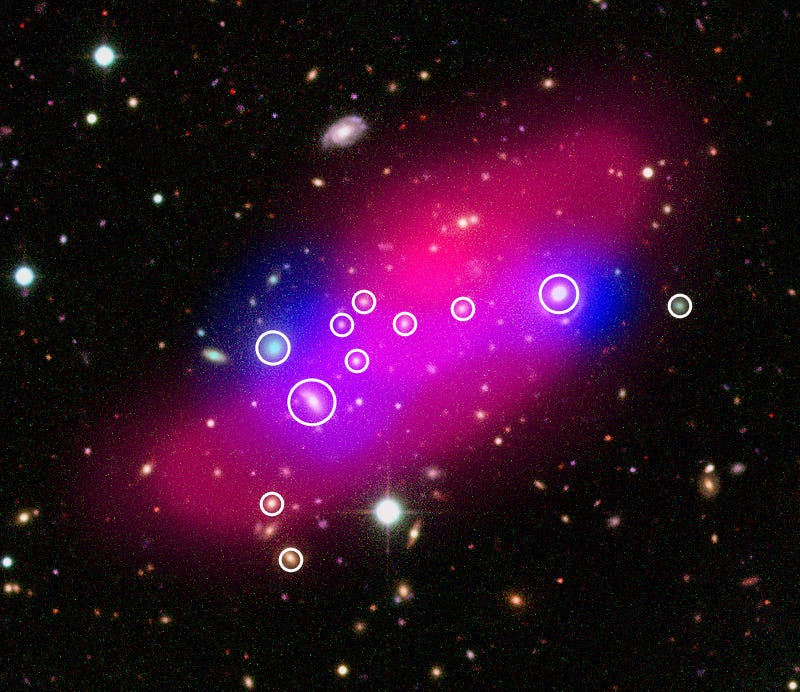
You can even see — in red — the background galaxies, from whose shape the gravitational lens mass was reconstructed! There’s simply no way to account for these observations by modifying gravity alone; you need dark matter no matter what you do to gravitation.
So not only do we have evidence for dark matter on scales of huge galaxy clusters, but now, for the first time, on the scales of individual galaxies within a very small group. All we can do, as good scientists, is follow the Universe to wherever the story it tells us about itself takes us.
Leave your comments at the Starts With A Bang forum on Scienceblogs!