Dark Matter: Giver of Life
Sometimes, it’s the most unexpected ingredients that give rise to the greatest results in the end.
“The privilege of a lifetime is being who you are.” –Joseph Campbell
When you look up past the stars of our Milky Way and out at the galaxies beyond, it might surprise you to learn that most of what we see isn’t most of what’s actually there. Sure, in our Solar System, 99.8% of the mass is in our Sun, and astronomy has taught us a tremendous amount about how stars work. So you might think that if you measure all the starlight — of all different types and wavelengths — coming from each individual galaxy we observe, we can figure out how much mass is in there.
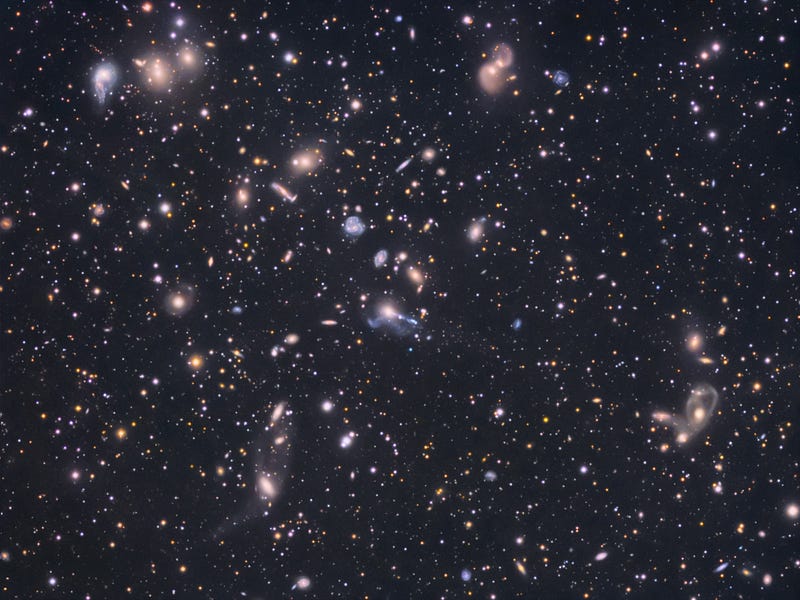
On the other hand, we know how the laws of gravitation work, and how the motions of gravitationally bound objects depend wholly on the total mass of the system and how that mass is distributed. So we can look both at
- individual galaxies and how the stars within them orbit, and also
- how entire galaxies move, individually, within giant galactic clusters.
When we make all of these measurements, we find a shocking fact: the measurement of mass from light and the measurement of mass from gravitation are off from one another by a factor of 50.
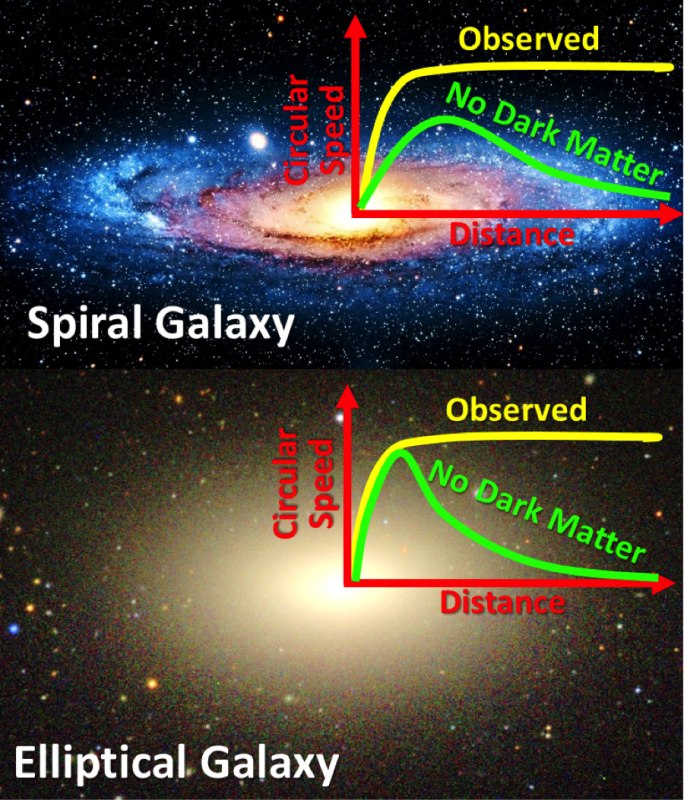
Now, we’ve discovered lots of other types of matter in the Universe besides stars, including:
- stellar remnants like white dwarfs, neutron stars and black holes,
- asteroids, planets and other objects with masses too low (like brown dwarfs) to become stars,
- neutral gas both within galaxies and in the space between them,
- light-blocking dust and nebulous regions,
- and ionized plasma, found mostly in the intergalactic medium.
All of these forms of normal matter — or matter originally made of the same things we are: protons, neutrons and electrons — do in fact contribute to what’s there, with gas and plasma in particular each contributing more than the sum total of all the stars in the Universe. But even adding all these components together only gets us up to about 15-to-17% of the total amount of matter we need to explain gravitation. For the rest of the motions that we see, we need a new form of matter that isn’t just different from protons, neutrons and electrons, but that doesn’t match up with any of the known particles in the Standard Model. We need some type of dark matter.
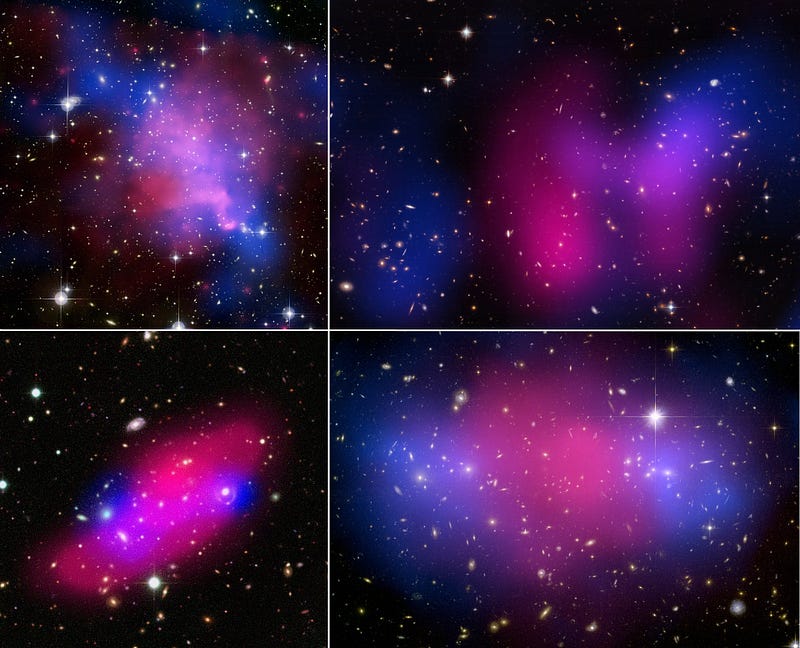
A minority group of scientists favor not adding some unseen source of mass, but to rather modify the laws of gravitation instead. These models all have difficulties, including the inability to reproduce the full suite of observations, including individual galaxies moving within clusters, the cosmic microwave background, galaxy cluster collisions (above) the grand cosmic web or the patterns observed in the large-scale structure of the Universe. But there’s an important piece of evidence that points to the existence of dark matter that you might not expect: our very existence.
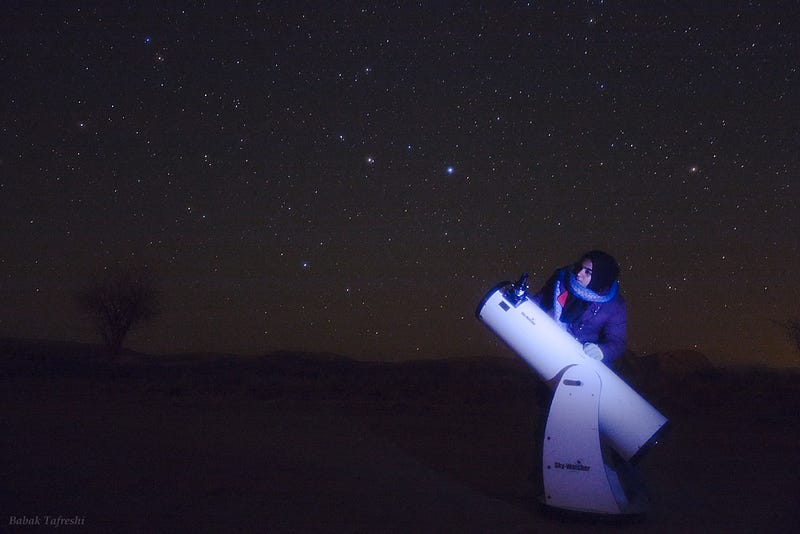
It might surprise you to learn that we don’t just need dark matter to explain astrophysical phenomena like galactic rotation, cluster motions and collisions, but to explain the origin of life itself!
To understand why, all you need to remember is that the Universe began from a hot, dense state — the hot Big Bang — where everything started off as a mostly uniform sea of individual, free, high-energy particles. As the Universe expands and cools, we can form protons, neutrons, and the lightest nuclei (hydrogen, deuterium, helium and a trace amount of lithium), but nothing else. It isn’t until tens or even hundreds of millions of years later that matter will collapse into dense enough regions to form stars and what will eventually become galaxies.
All of this will happen just fine, albeit differently in detail, whether there were plenty of dark matter or none at all. But in order to make the elements necessary for life in great abundance — elements like carbon, oxygen, nitrogen, phosphorous and sulphur — they need to be forged in the cores of the most massive stars in the Universe. They do us no good in there, though; in order to enable the creation of rocky planets, organic molecules and (eventually) life, they need to eject those heavier atoms back into the interstellar medium, where they can be recycled into future generations of stars. To do that, we need a supernova explosion.
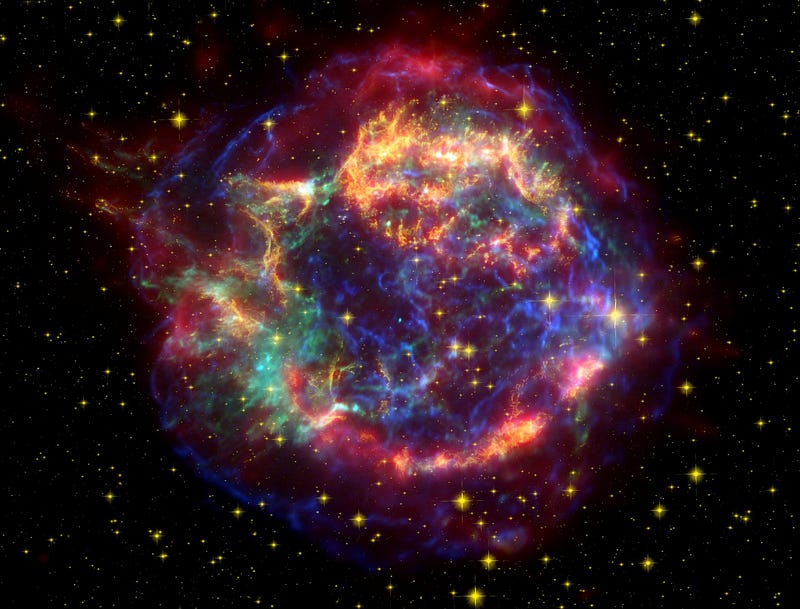
But we’ve observed these explosions in great detail, and in particular, we know how quickly this material gets ejected from the stars in their death throes: on the order of a thousand kilometers per second. (The Cas A supernova remnant, our galaxy’s most recent, verified one, has ejecta leaving it between a whopping 5,000 and 14,500 km/s!) While this may not sound like that big a number, especially compared to the speed of light, remember that our own star orbits the Milky Way at only some 220 km/s. In fact, if the Sun were to move even three times as fast as that, we’d find ourselves — today — escaping well beyond our galaxy’s gravitational pull.
A supernova remnant might see the fastest of its ejecta leave the luminous, star-based part of the galaxy, but combined with the intense gravitational pull of a diffuse, extended halo of dark matter, we’ll keep most of that mass inside our own galaxy. Over time, it will fall back towards the normal-matter-rich regions, form neutral, molecular clouds, and participate in subsequent generations of stars, planets, and more interesting, organic molecular combinations.
But without the additional gravitation of a massive dark matter halo surrounding a galaxy, the overwhelming amount of material ejected from a supernova would escape from galaxies forever. It would wind up floating freely in the intergalactic medium, never to become incorporated into future generations of star systems. In a Universe without dark matter, we’d still have stars and galaxies, but the only planets would be gas giant worlds, with no rocky ones, no liquid water, and insufficient ingredients for life as we know it. Without the copious amounts of heavy elements provided by generations of massive stars, molecule-based life like us would never have come to be.
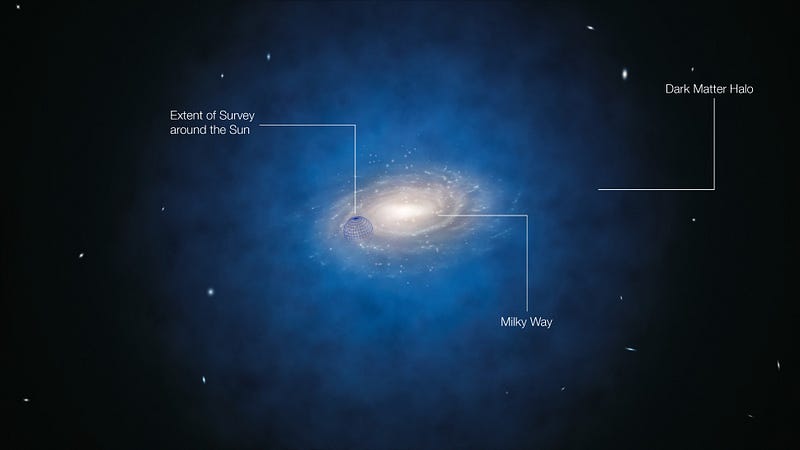
It’s only the presence of these massive dark matter halos, surrounding our galaxies, that allow the carbon-based life that took hold on Earth — or a planet like Earth, for that matter — to even be a possibility within our Universe. As we’ve come to understand what makes up our Universe and how it came to be the way it is, we’re left with one inescapable conclusion: dark matter is absolutely necessary for the origin of life.
Without it, the chemistry that underlies it all — the heavy, complex elements, the ingredients necessary for biology in the first place — could never have occurred.
Leave your comments at the Starts With A Bang forum on Scienceblogs!