Dark energy and the myth of the disappearing Universe
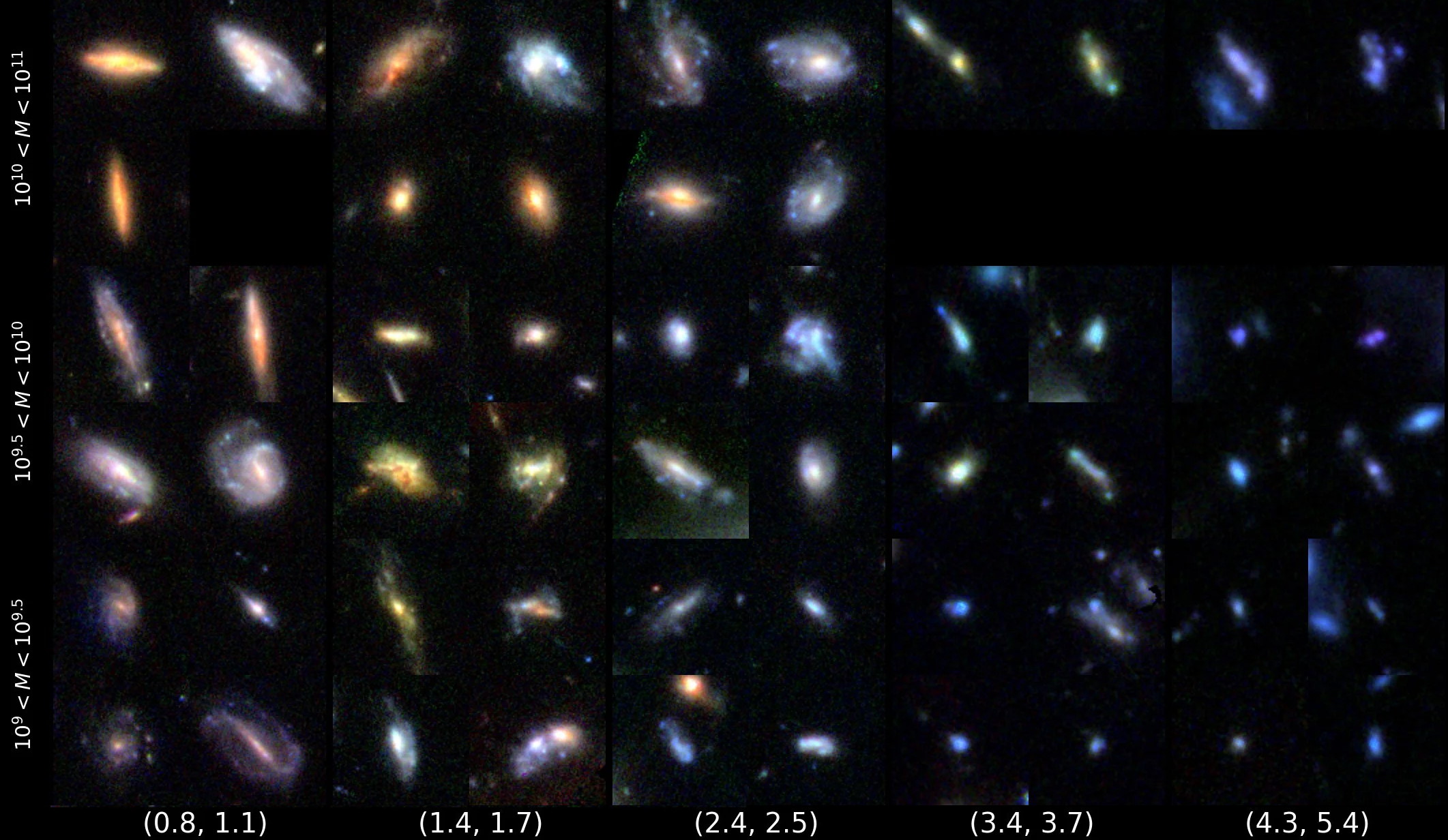
- Our Universe isn’t just expanding, but the expansion itself is accelerating owing to the presence of a form of energy that doesn’t dilute as the Universe expands: dark energy.
- Any individual object, like a galaxy, appears to recede faster and faster from any other as time goes on, driving unbound objects apart at ever increasing speeds.
- While these galaxies will become unreachable, the notion that they’ll disappear from view is misleading, as more and more of the Universe becomes visible as time goes on. Here’s the counterintuitive science of why.
Since the dawn of humanity, we’ve wondered just what our Universe is. What is it made of? How is it structured? Where did it come from? How did it get to be the way it is today? And what will be its ultimate fate? After millennia of wondering and philosophizing, the past ~200 years of scientific investigation have finally brought the answers to us. The Universe is made not just of atoms, neutrinos, and photons, but also of two mysterious substances: dark matter and dark energy. We emerged from an early, hot, dense state; we gravitated and cooled; now the Universe is cold, low in matter density, and we have seen what our ultimate fate will be.
Owing to the presence of dark energy — the most dominant but least understood component of the Universe — we now know what’s in store for us in the far future. Objects that are gravitationally bound together, like planets, stars, stellar systems, galaxies, and galaxy clusters, will remain so. But larger-scale objects will be driven apart by the expanding Universe, receding from one another at ever-increasing speeds. We colloquially call this “the disappearing Universe,” but that’s simply a myth. Nothing is disappearing from view, although things are disappearing from our reach. Here’s why that difference is so important.
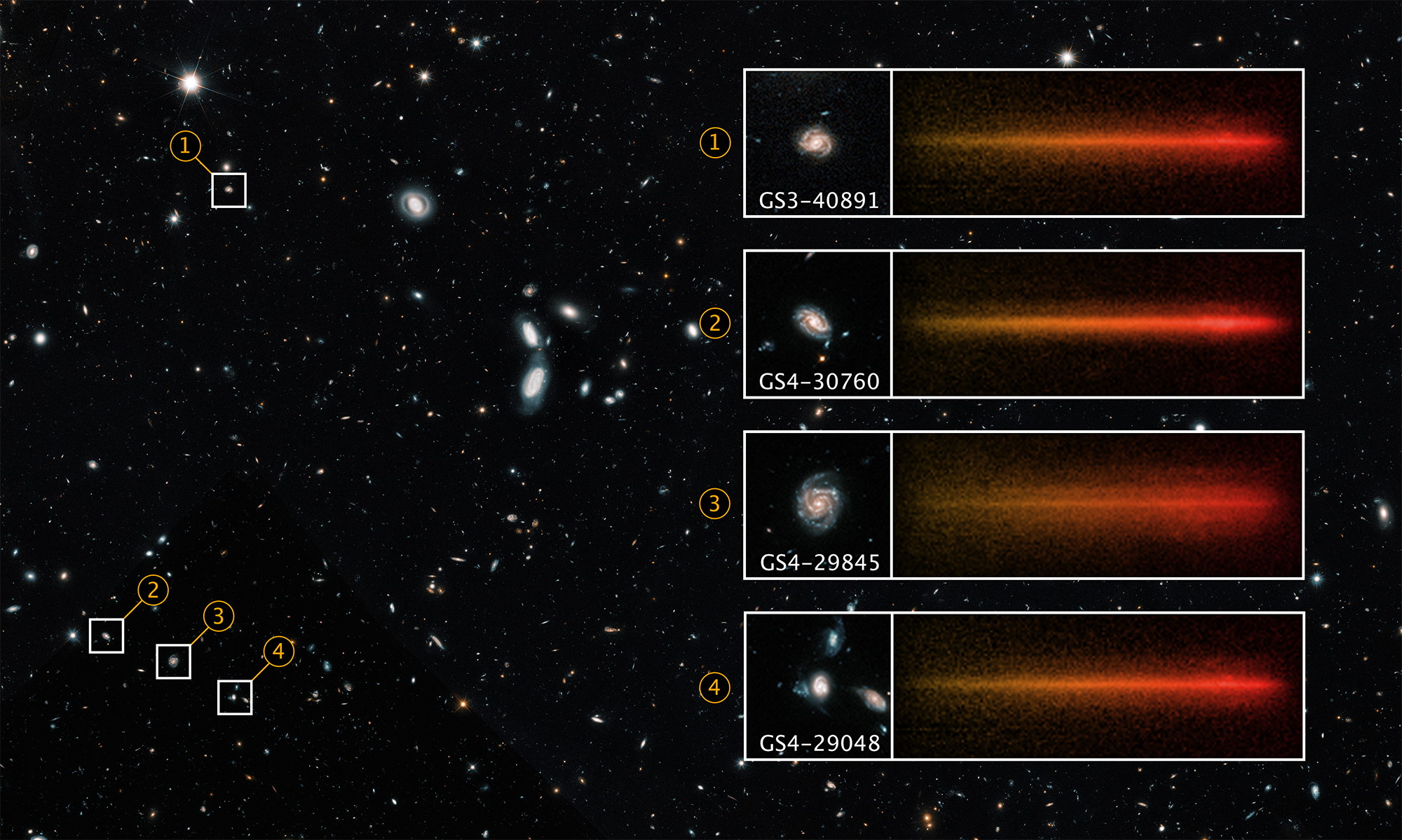
If you want to know how the Universe is going to behave into the far future, there are only three things you need to understand and/or measure in order to make an accurate set of predictions.
- You must know the laws that govern the Universe on the largest scales: in this case, General Relativity, our theory of gravity, is presumed to work extraordinarily well, having passed every cosmic test thrown its way.
- You must be able to measure how quickly the Universe is expanding today: the expansion rate as we perceive it to be right at this moment in time. Although two different methods yield results that differ by about ~9% from one another, this difference, as well as the uncertainty we have about the “true value,” is still relatively small.
- And you must be able to measure how the expansion rate has changed and evolved over time, or in other words, how the Universe was expanding at various points over its cosmic history. Recent measurements (in the last ~30 years) of distant supernovae and the large-scale structure of the Universe have enabled us to make this measurement to outstanding precision as of 2023.
Put these three pieces of knowledge together, and one of the lessons we can derive from them is what the ultimate fate of the Universe will be.
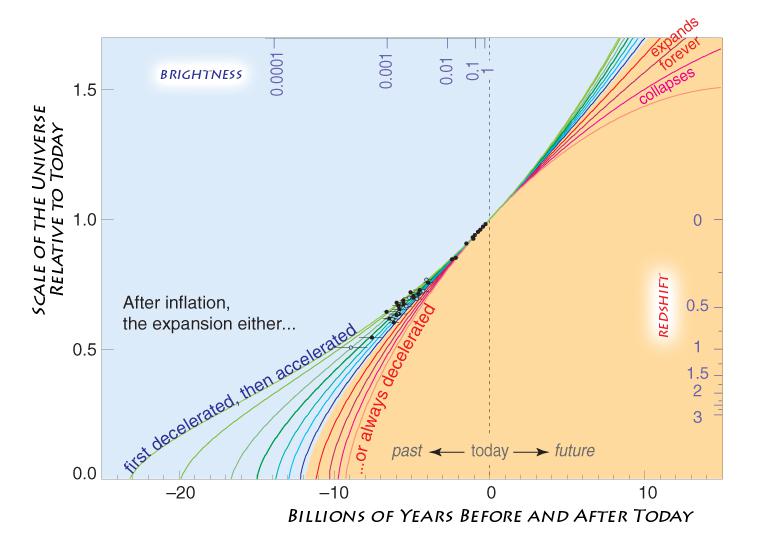
It all comes down to the simple fact that the Universe expands at a rate that depends on all the different forms of energy in it, combined. As the Universe expands (and its volume increases), the energy densities of all those various forms of energy change in predictable, understandable, and measurable ways. If we know the laws of physics and can measure how the Universe expands now and how that expansion rate changed in the past, we can determine what the various forms and ratios of energy in the Universe are, were, and will be at any moment in time.
This is the secret to uncovering not only the Universe’s origins, contents, and past behavior, but it allows us to know what’s in store for us well into the distant future as well. Matter, for example, is made of massive particles whose rest-mass energy, given by E = mc², doesn’t change over time. However, the matter density — determined by energy-per-unit-volume — does change, as the volume keeps increasing as the Universe expands. (This applies equally to both normal matter and dark matter.) Radiation density changes even more severely, as not only does the volume for each quantum of radiation increase as the Universe expands, but the energy-per-quanta drops as the expanding Universe stretches the wavelength (and hence, decreases the energy) of each photon.
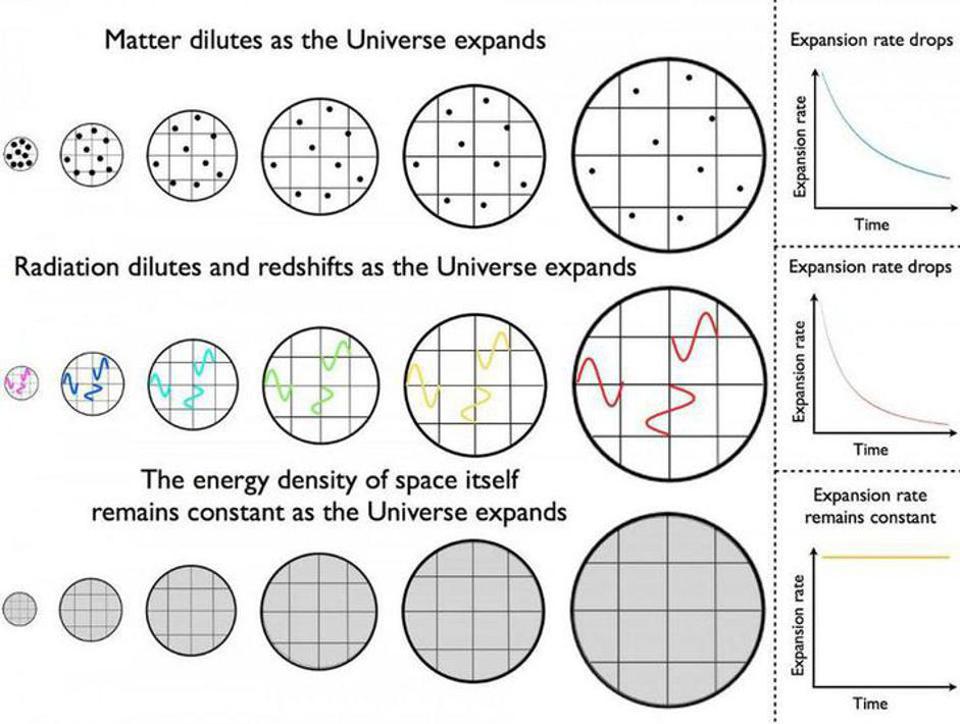
But dark energy is different than all of these. In fact, dark energy’s defining property is that it functions not as something made out of particles — something that gets less dense as the Universe expands — but rather as a form of energy that’s inherent to space itself. Regardless of what dark energy’s nature is:
- a new field that’s inherent to space,
- a form of energy that gets newly created as new space gets created in the expanding Universe,
- a manifestation of the zero-point energy of the quantum fields present in the Universe,
- or a positive cosmological constant that shows up in Einstein’s General Relativity,
its measured properties are consistent with it having a constant energy density. As the Universe continues to expand, all the other forms of energy within it see their densities drop, but dark energy’s density remains constant.
Early on, radiation was the most important form of energy in determining the Universe’s expansion; after about ~10,000 years, matter (both normal and dark, combined) became the dominant factor. It was only after billions of years that matter diluted enough so that dark energy could become detectable. Over the past 6 billion years, it’s become the most important component in the Universe, primarily determining how it expands.
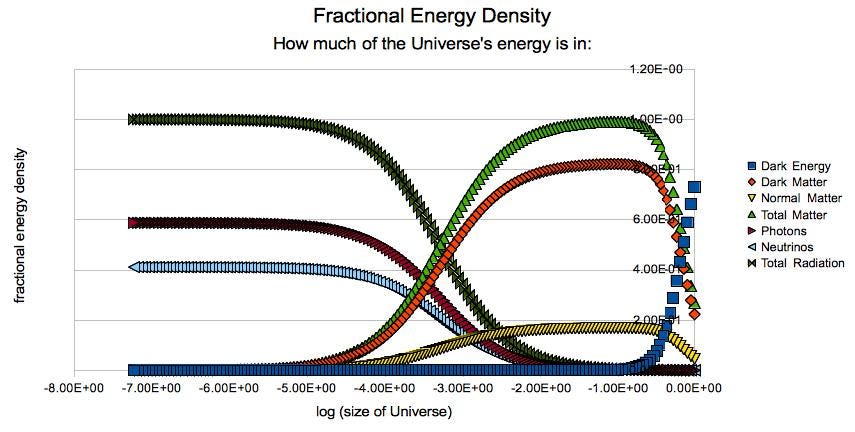
We can now state, with a very strong degree of confidence, that dark energy really is neither increasing nor decreasing in density over time, something that the future Nancy Roman Observatory should be able to constrain all the way down to the ~1% level. This allows us to infer the fate of the Universe, which is as follows.
- Regions of space that attracted enough matter into them to exceed the cosmic average by a critical amount — about ~68% — become gravitationally bound, which leads to structures like galaxies, galaxy groups, galaxy clusters, and even collections of clusters.
- Individually bound regions that aren’t bound to any larger structures — like our Local Group of galaxies, which isn’t bound to a larger group or cluster — will expand away from one another forever more.
- And that if you measure any one distant, unbound object over time, you’ll find that it appears to recede from us faster and faster as time goes on, as its redshift (and inferred recession speed) only increases with time.
That last point, of a distant, unbound object appearing to recede faster and faster from any other object that it’s not bound to, is where the idea of an accelerated expansion to the Universe comes from.
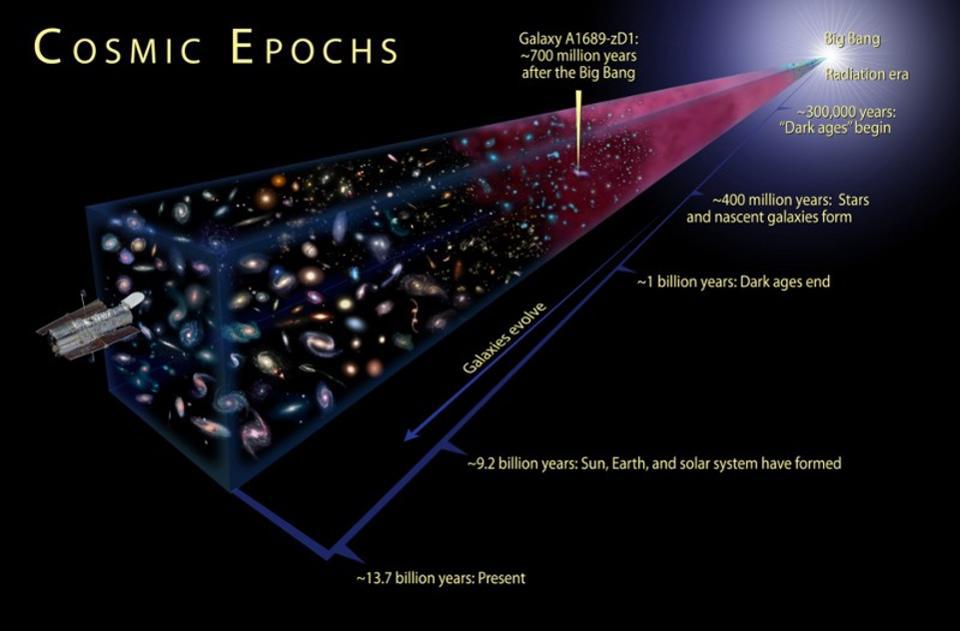
But the implications that come from an accelerated expansion of the Universe are not necessarily what you might intuit, even if you’re an astrophysicist yourself. If the Universe didn’t have dark energy — if it were composed of various forms of matter and radiation alone — things would have been a lot simpler. As time went on and the Universe expands, in a dark energy-free Universe:
- The expansion rate, measured in speed-per-unit-distance (km/s/Mpc), would asymptote to zero.
- Any distant galaxy, anywhere in the Universe, would seem to slow down over time as far as its apparent recession speed went.
- Once a distant object became visible, it would remain visible for all eternity.
- Any object that can be seen can also be reached, eventually, even if the journey would take longer than the present age of the Universe.
- And that greater and greater portions of the Universe — including regions well outside of the presently observable Universe — would continue to come into view and come within reach as time went on.
All of these things, so intuitive and simple in a Universe without dark energy, need to be questioned and re-evaluated in the modern context of a Universe with dark energy.

Once you add in even a sprinkling of dark energy — no matter how tiny the amount — many of these features change. With even a small initial amount of dark energy, you’re guaranteed that, as the matter and radiation density drop, the dark energy density (which remains constant) will someday become dominant, as there’s no limit to how dilute the matter and radiation in the Universe will get. With dark energy present, here’s how each of those earlier facts change:
- The expansion rate, in km/s/Mpc, doesn’t asymptote to zero, but rather to a finite, positive value that’s greater than zero.
- Any distant galaxy, unbound from the observer, will recede for all eternity, with its apparent recession speed increasing the farther and farther away it gets.
- Once a distant object becomes visible, it remains visible, but only as it was long ago in the past; what’s happening today in a distant galaxy isn’t necessarily visible to an observer.
- Only objects that recede at speeds below a critical threshold, necessarily below the speed of light, can ever be reached by an ambitious space traveler; much of what can be seen can never be accessed.
- And that only a finite volume of space, beyond what’s presently visible, will ever be observable even as an arbitrary amount of time passes.
This is a very different story from the one that would come to pass in a dark energy-free Universe, with astounding implications for what the far future of the Universe holds.
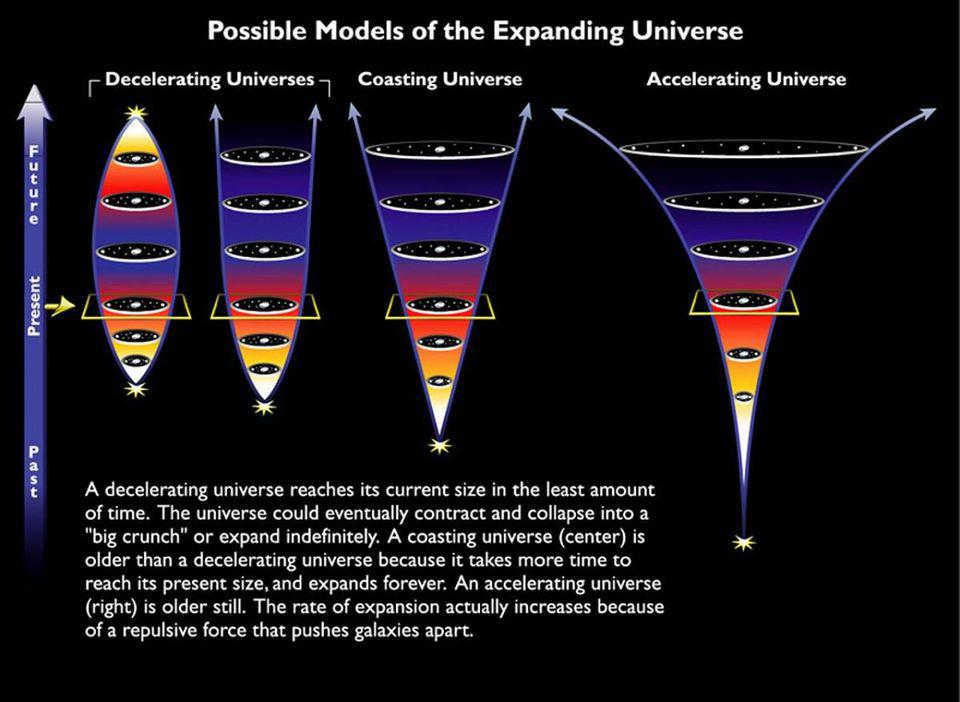
One way to view the Universe is as a great cosmic race. On the one hand, there’s the initial expansion, which works to drive all the objects in the Universe apart from one another. On the other hand, there’s gravity, which works to attract everything back together. The Big Bang — the moment at which this race begins — is like the starting gun of this race. If your Universe has too much energy, you’ll overcome the expansion, leading to a recollapse. If your Universe has too little energy, the expansion will easily win, driving all the “bits” of energy apart before any stars, galaxies, or bound structures can form.
On small cosmic scales, there are regions where gravitation wins, leading to stars, galaxies, clusters of galaxies, and more, but more regions where it loses, leading to empty regions of space, or cosmic voids. The light from distant objects gets emitted in all directions, and as time marches onward, there’s a finite distance that light can reach at each moment. If there were no dark energy, the initial expansion and the effects of gravity would have balanced, leaving the Universe’s expansion rate to asymptote toward zero but never to cease, reverse, and recollapse.
With dark energy present, however, there will be a minimum recession speed reached by all objects, and then beyond that, they get driven farther and farther apart. As a result:
- only objects within a finite distance can ever be seen,
- only a subset of those objects can ever be reached,
- and the ones that can be seen but not reached can only be seen up to a finite moment in time. We’ll always be able see things as they were, but not necessarily as they are or as they will be.
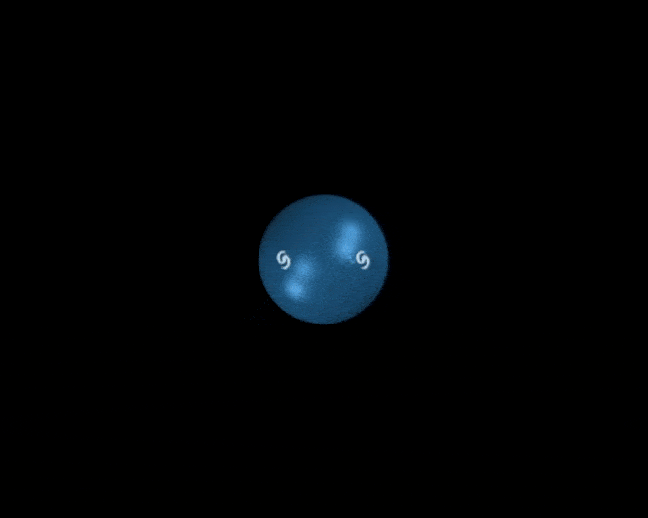
Today, the observable Universe extends for 46.1 billion light-years from the perspective of any observer; it’s grown to this size over the past 13.8 billion years. This means that light, after journeying at the speed of light since the first instant of the hot Big Bang, would just be arriving at our eyes right now from an emitted location that is presently 46.1 billion light-years away. Everything within that imaginary sphere can be observed, and light from any such object will always continue to arrive.
However, light from farther away — emitted from objects that are up to about 61 billion light-years away at the present moment — is still on its way, and will eventually arrive at our eyes. This represents about an additional 130% of the volume of the Universe, which will eventually become visible; we call this the future visibility limit.
But only objects within about 18 billion light-years, representing (by volume) only about 6% of the observable Universe, can ever be reached. A spaceship that left today at the speed of light (or arbitrarily close to it) could reach a distant galaxy that’s 18 billion light-years or closer, but not one that’s farther away. A light signal emitted by us right now won’t ever reach a more distant object, and a light signal emitted by a more distant object can never reach us.
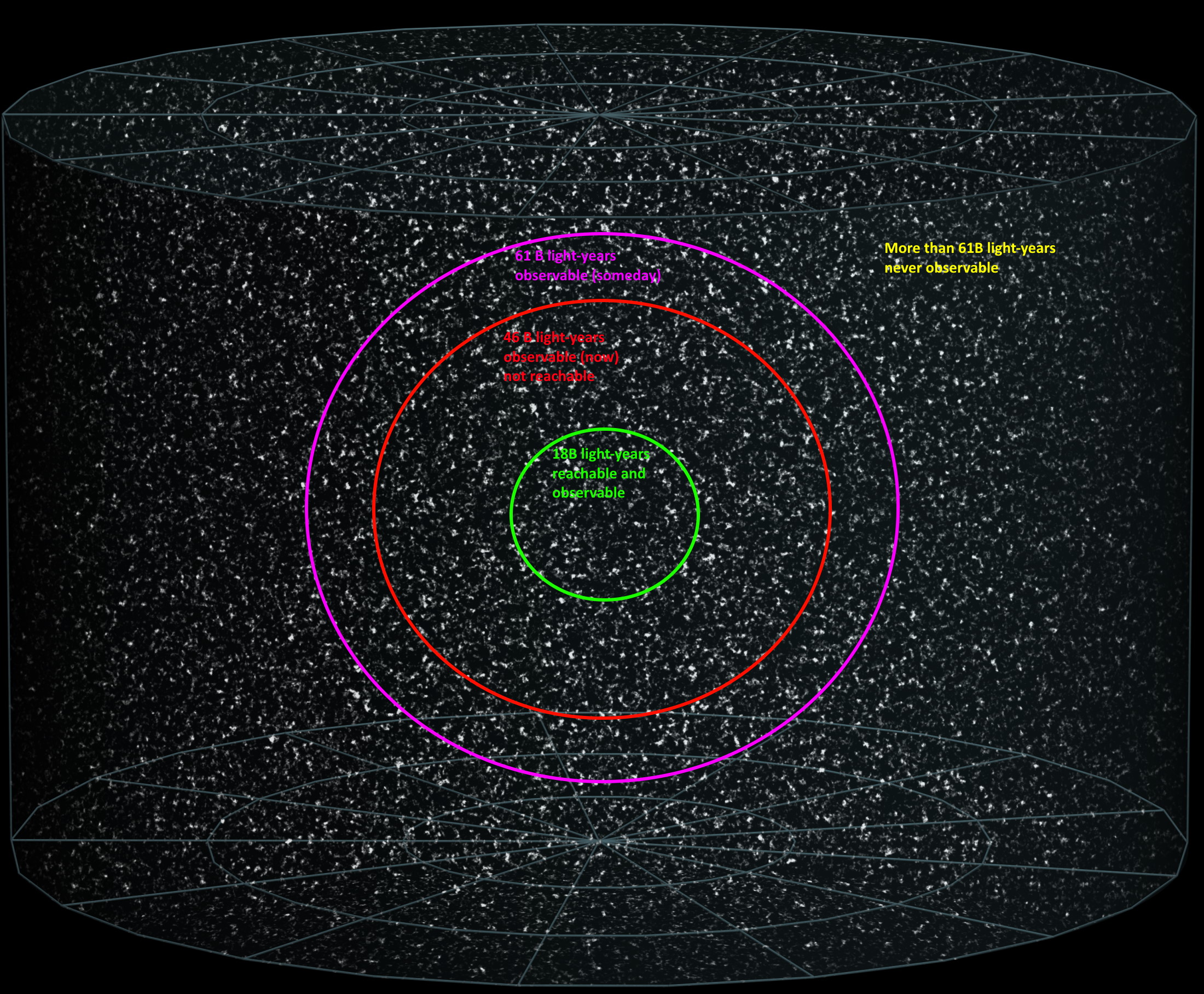
All told, this means there are four different categories that objects fall into, depending on how far away they are and how strong dark energy is relative to the other forms of energy (and the expansion rate) in the Universe.
- Reachable and observable: objects that are still receding slower-than-light can be seen (forever) and reached (for now), so long as they’re closer than 18 billion light-years away.
- Observable but unreachable: objects being driven away by dark energy can’t be reached, even now, but their light has already arrived and will continue arriving for as long as we’re observing. We can see these objects as they were but not as they are or will be, corresponding to objects between 18 and 46 billion light-years away.
- Someday observable: some objects that can’t be reached and can’t be seen, if they’re close enough to our present cosmic horizon, will have their long-ago emitted light arrive someday in the future. These objects will be observable in the future (but aren’t yet today), and correspond to objects between 46 and 61 billion light-years distant.
- Always unobservable: and then there’s that last category of objects whose light has never arrived and never will, which corresponds to everything over 61 billion light-years away today.
Objects in the first category are disappearing from reach, but objects in categories 1, 2, and 3, once they become observable, will always remain observable and will never disappear from view. Our ability to reach or communicate with objects beyond a certain distance is what’s disappearing, but the objects themselves will always remain perceptible. And that’s the truth behind the myth of the disappearing Universe!