What will happen when a black hole hits Earth?
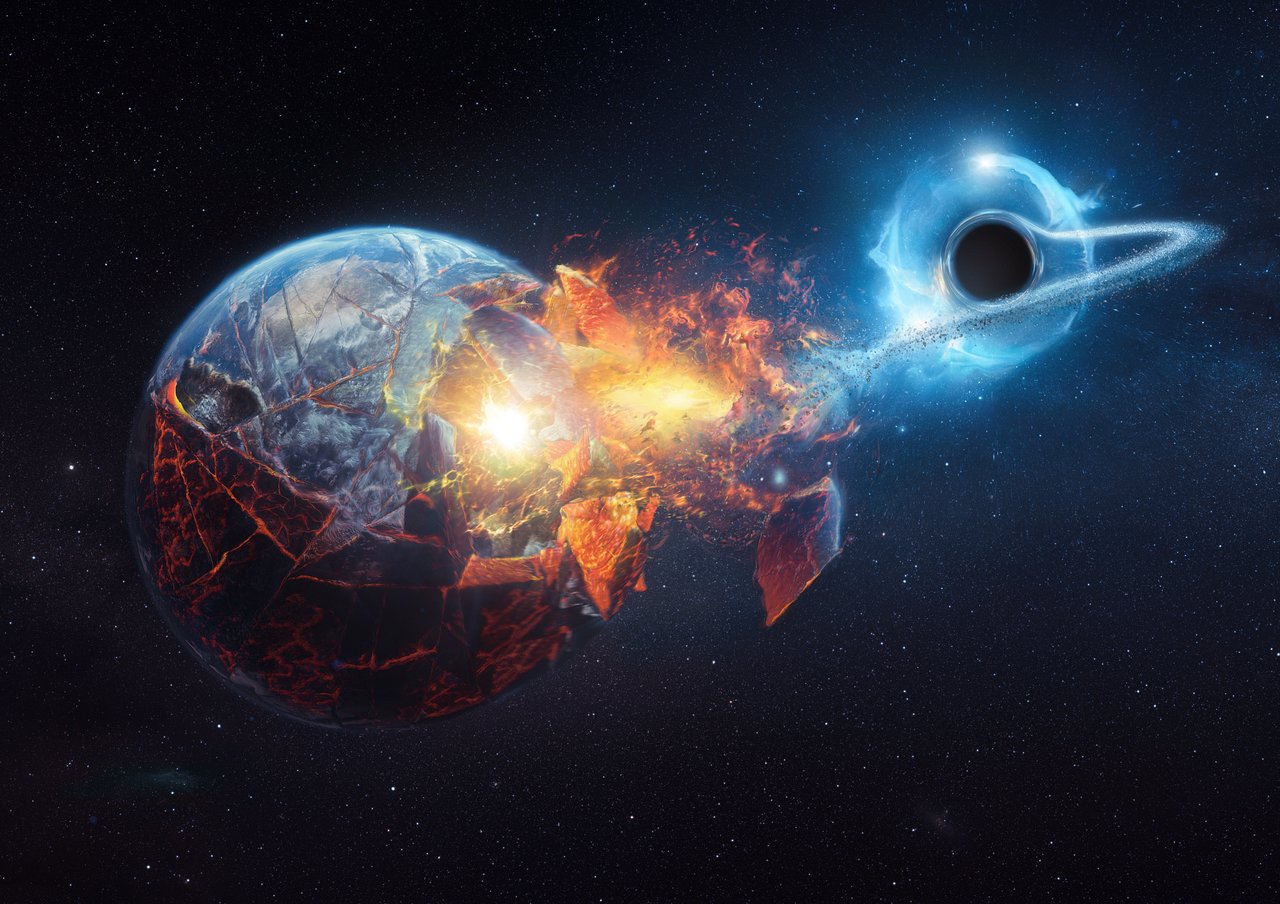
- In the Milky Way alone, hundreds of billions of stars have been born, which means there are hundreds of millions of black holes to look out for.
- Just like stars, black holes move through space, but unlike stars, they emit no light, so a “stealthy” black hole could sneak up on us, unseen.
- The ultimate catastrophe imaginable is that a black hole collides with Earth. Even though it’s unlikely, there’s a lot to learn from just thinking about the problem.
Out there, somewhere in the Universe, our potential doom awaits us. Although the stars in the night sky seem fixed and unmoving, much like our Sun, they’re all engaged in the same gravitational dance that keeps us in orbit around the center of the Milky Way. Every stellar system out there is in motion relative to the Sun, and periodically — a few times every million years or so — one of those objects gets perilously close to our Solar System. When that occurs, it typically perturbs some of the members of our Oort cloud, leading to a potential barrage of comets down the line.
Although that’s what most frequently occurs, worse outcomes can indeed ensue. Stars could pass through the Solar System, affecting the orbits of the planets. Other objects like black holes, neutron stars, white dwarfs, and rogue planets can do the same thing, knocking objects around like a cosmic game of billiards. In the worst case scenario, you can even imagine a black hole striking the Earth. The odds of it may be unlikely at any moment in time, but astronomical timescales are very long and the Universe has many, many opportunities to create even extremely unlikely catastrophes. Here’s what will happen when a black hole hits Earth, and what chances we have to do something about it.
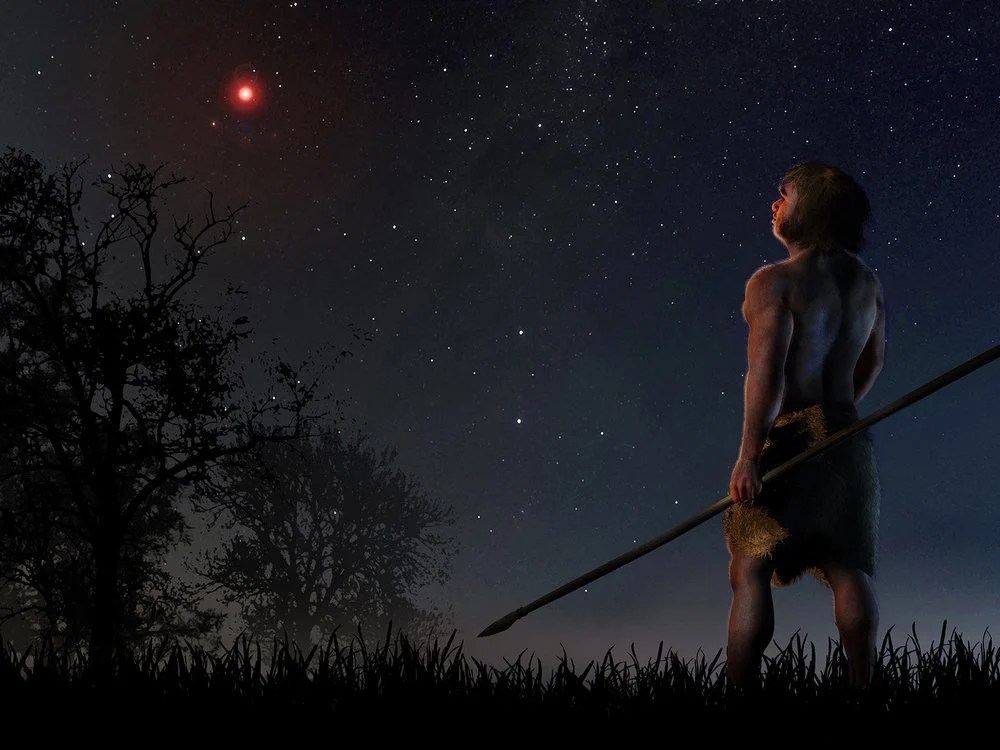
Credit: José A. Peñas/SINC
What are the odds of anything hitting us?
Let’s start with some good news: although we have an enormous number of black holes in the Universe, including in our own galaxy, the odds that one of them will strike the Earth are incredibly small. There are an estimated 400 billion stars in the Milky Way galaxy, and even though the individual stars themselves are large, the distances between the stars are enormous compared to not only their sizes, but even the sizes of the stellar systems that they anchor.
A star like our Sun is about 1.4 million kilometers in diameter, and planet Earth orbits around our Sun at a distance of about 150 million kilometers (what we define as one Astronomical Unit, or A.U.): about 100 times as distant. The Kuiper belt, out beyond the orbit of Neptune, is about another 50 times as distant: several billion kilometers are required to map it out, and the Oort cloud persists for about a thousand times the distance to the Kuiper belt, measured in the trillions or even tens of trillions of kilometers.
That last figure — ten trillion kilometers — is approximately equal to one light-year. For comparison, the nearest star to us, Proxima Centauri, is a little over 4.2 light-years away; light-years are typically what we use to describe the distances between stars.
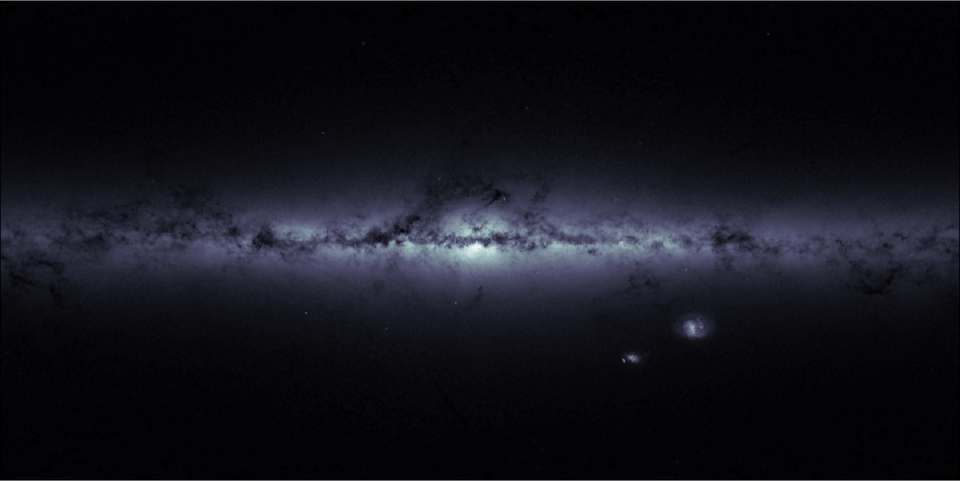
Based on the number of stars and how they’re distributed throughout our galaxy, something we now have an extraordinary census of thanks to the achievements of the Gaia mission, we know a variety of fantastic facts about our nearby Universe.
- The stars in our neighborhood typically move at speeds, relative to us, of around 20 km/s, or about 10% of our speed around the galactic center.
- Almost all of the stars at our distance from the galactic center move in ellipses that are not particularly eccentric: they’re pretty close to circles.
- In addition, almost all of them orbit in the (thin) galactic disk at our distance; very few are in the central bulge or in the larger, spheroidal galactic halo.
- And about half of those stars exist as part of a multi-star system, where only approximately half are like our own Solar System: with a single star all by its lonesome.
If we do the math, we can calculate, on average, how long it takes a star to get within a certain distance of our Sun. The results are interesting, but thankfully, not frightening.
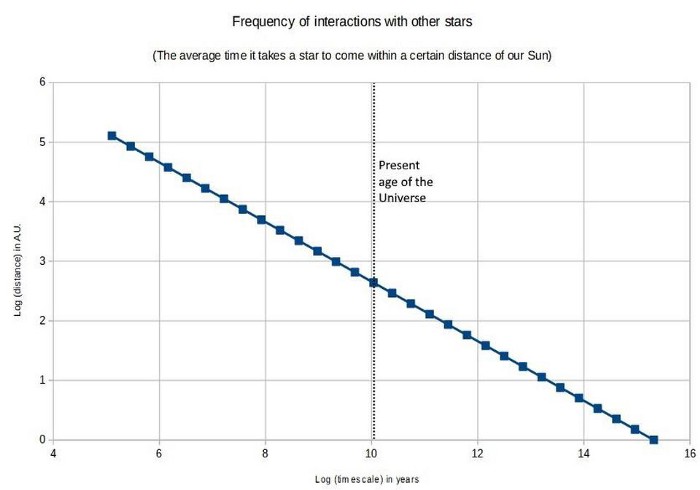
Credit: E. Siegel
Our Solar System has been around for about 4.5 billion years, or just to the left of the “10” on the x-axis on the graph above. Once every few hundred thousand years, a star comes close enough to influence our Oort cloud, with the most recent one being Scholz’s star, having done so about 70,000 years ago.
It’s unlikely, however, that any star ever came close enough to knock the other large bodies in our Solar System off course. The closest we can expect another star to have come, over our entire planet’s existence, is about ~500 A.U. away, or about ten times the distance from the Sun to Pluto. In particular, over our entire Solar System’s history, there’s only been:
- a ~1% chance of a star coming close enough to disrupt our Kuiper belt,
- a ~0.01% chance of a star coming close enough to disrupt Jupiter or Saturn,
- and a ~0.0001% chance, or around 1-in-a-million, of a star coming close enough to gravitationally disrupt the Earth,
- with only a ~0.000001% chance, or 1-in-100,000,000, of a star actually colliding with the Earth.
Given that the planets and, indeed, the Kuiper belt appear not to be disrupted from what we presume our initial configuration was some 4.5 billion years ago, these numbers pass the smell test. The greatest danger to Earth comes from a passing star disrupting our Oort cloud, and sending a potential planet-killer our way. In the far future, however, it’s almost a certainty that the gravitational dance of objects in our galaxy will lead to the eventual ejection of most planets contained within stellar systems.
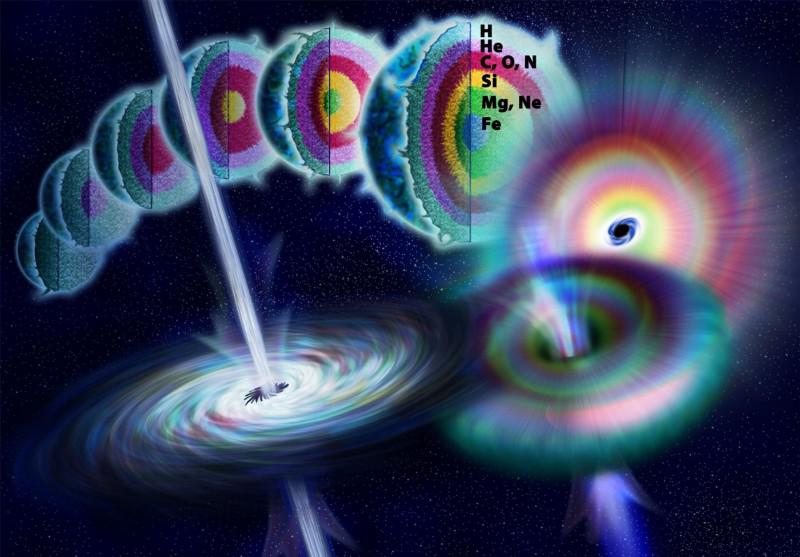
Credit: Nicolle Rager Fuller/NSF
So what about black holes?
You have to understand that all of this “talk about stars” isn’t just for kicks, but rather sets us up extraordinarily well for having the same discussion about black holes. Sure, black holes are harder to detect because they emit no light, but the physics behind their existence and their motion through the galaxy is identical. The reason why is simple:
pretty much every black hole in the Universe, especially in our portion of the galaxy, arose from a pre-existing star.
Yes, there are supermassive black holes, but those are pretty much exclusively found in the centers of galaxies; we are tens of thousands of light-years away.
No, there isn’t any observational evidence for primordial black holes, which would be more numerous and lower in mass, and some serious theoretical difficulties stacked against their existence.
Instead, the two most common ways of making a black hole in the Universe are to either create a massive enough star that its core will collapse and form a black hole, or to have two neutron stars collide and merge above some mass threshold, again giving rise to a black hole.
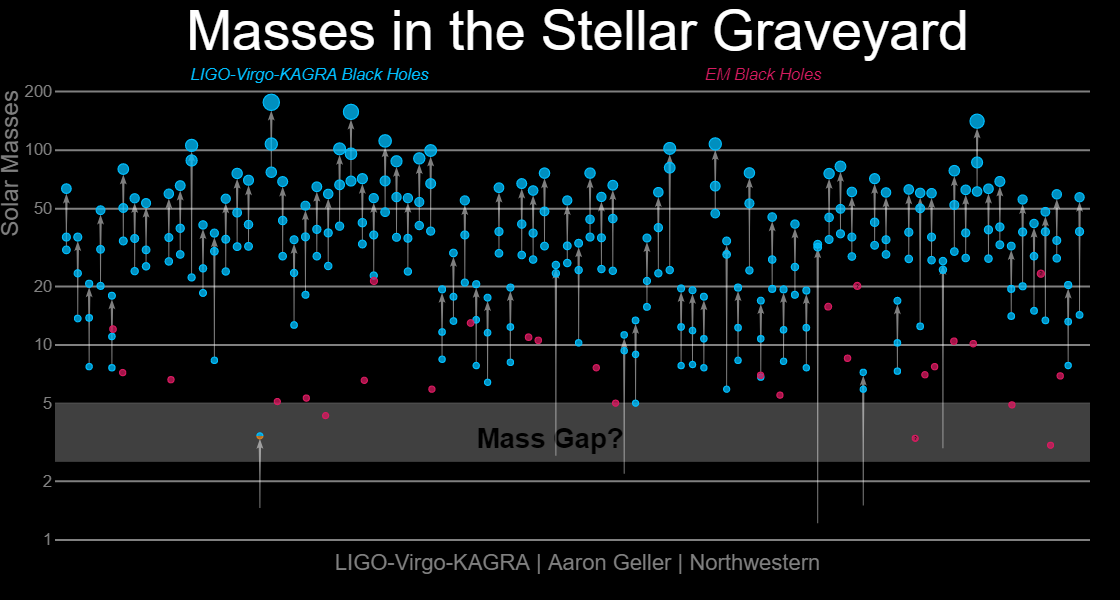
Once we understand this, we can estimate the number of black holes relative to the number of stars. Approximately 0.12% of all the stars that have ever formed, or about 1-in-800 of them, are massive enough so that when they finish their life cycle, they’ll produce a stellar mass black hole: greater than about 3 solar masses but no more than a few hundred solar masses, tops. It’s plausible — although some would argue that it’s generous — that perhaps neutron star-neutron star mergers, which have been observed by gravitational wave detectors such as LIGO and Virgo, might account for up to just as many black holes as these massive stars do, although they’d be exclusively on the low end of the mass range.
Even taking the optimistic estimate, that would mean there are up to approximately ~1 billion black holes traveling through the Milky Way right now, as opposed to ~400 billion stars. That’s an incredible number of black holes to reckon with, but even though astronomical timescales are, well, astronomically long, we still have extremely low odds of experiencing an interaction with a black hole. In fact, if we’re only considering a collision between a black hole and Earth, the odds are minuscule: about 1-in-40 billion over the history of the Earth, and about 1-in-1020 (or, written out, 1-in-100,000,000,000,000,000,000) with each passing year, or your odds of winning the lotto jackpot three times in a row.
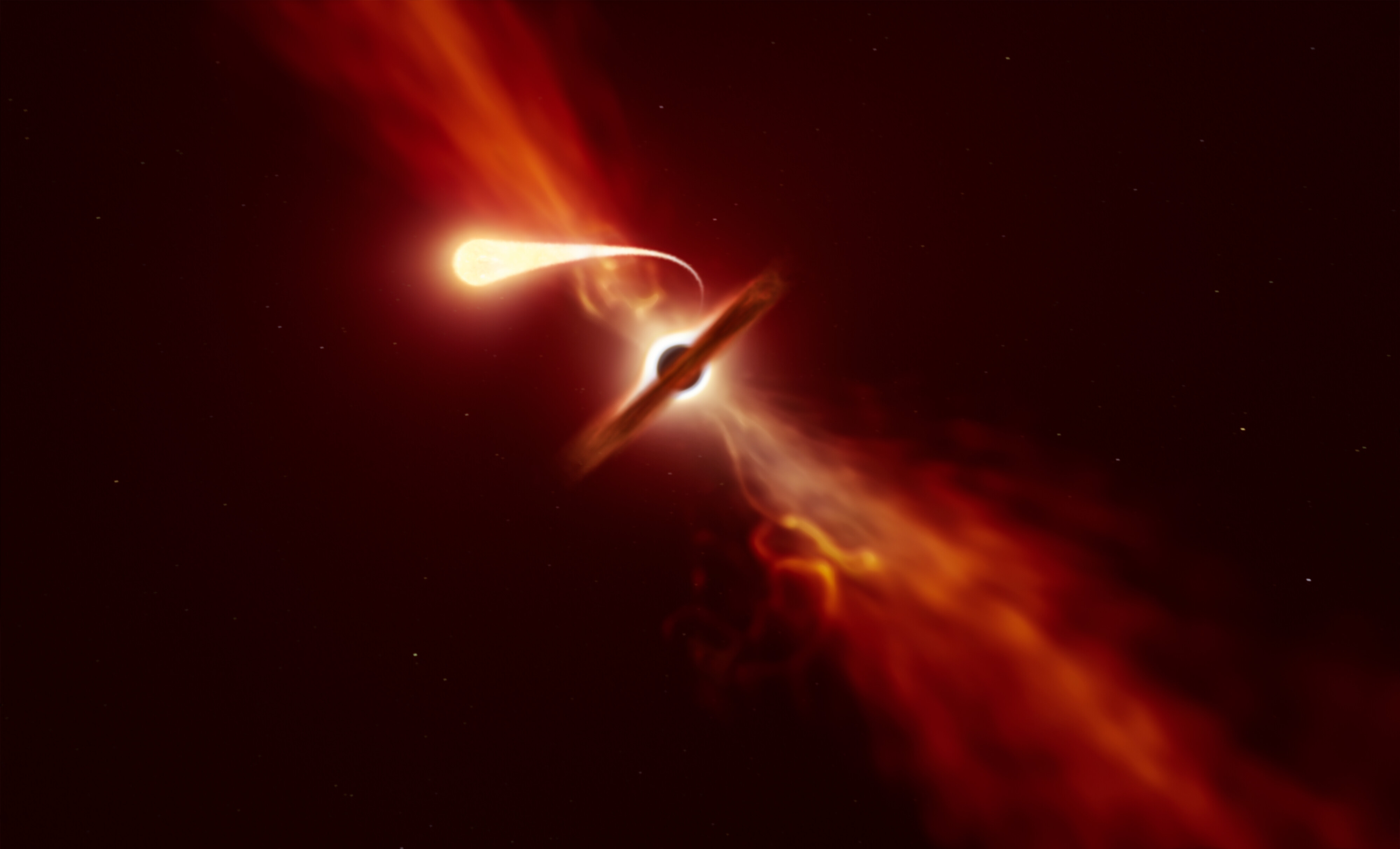
Credit: ESO/M. Kornmesser
Collisions aren’t the only danger
Of course, a black hole doesn’t need to collide with you to pose a threat. If it gets close enough to you, it can:
- gravitationally wreck your orbit,
- eject you from your stellar system entirely,
- or even spaghettify you, where the tidal forces shred the planet apart entirely.
These are things to be wary of, but fortunately, a black hole would have to get remarkably close to cause any of these problems.
The black hole would have to get close enough to Earth to exert a gravitational force comparable to that of the Sun, but remember that gravity falls off as one over the distance squared. Even a black hole that was 100 times as massive as the Sun — more massive than 99% of black holes in the galaxy — would have to come within about 10 Astronomical Units of the Earth to compete with the Sun in terms of a gravitational force. That’s riskier, as there’s about a 1-in-400,000,000 chance of that happening over our Solar System’s history, but that’s only 100 times likelier than being hit by the black hole directly. (The other options, of ejection or spaghettification, fall in between those two estimates.)

Can we know if danger is coming?
“At least,” you might think, “if a star is going to come into our Solar System and give us a serious cosmic makeover, we’ll see it coming.” But is there any way to get a warning that a black hole is on its way?
The answer, remarkably, is absolutely yes. Black holes might not be luminous, but they do gravitate just as strongly as anything with the same amount of mass. In addition, because black holes aren’t extended objects that take up a large volume, like stars are, but rather are collapsed down into very small regions of space hidden behind a tiny event horizon, they strongly distort the light from objects that appear behind it relative to our perspective.
That means we have three ways to detect the presence of a black hole that gets close enough to our neighborhood.
- It can cause strong gravitational lensing, where background objects that are near the same line-of-sight will have their light bent, stretched, and distorted in an easily identifiable fashion.
- It can cause weak gravitational lensing, where background objects that are farther away will have their apparent shapes distorted in a way that would never occur naturally.
- And it can cause microlensing, where the passing black hole, instead of occulting a background star, would amplify its light and cause a temporary but tremendous brightening; an unmistakable signature of even an invisible mass.
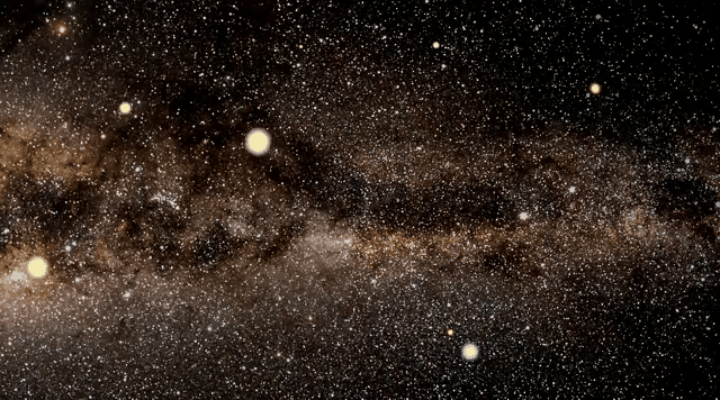
Credit: Jan Skowron/Astronomical Observatory, University of Warsaw
Is there a way to save ourselves?
In many ways, it’s clear that we’ve won the cosmic lottery by simply coming into existence, and having life survive and thrive on our planet for pretty much the entirety of its existence. What would happen, then, if we discovered we were about to lose the ultimate cosmic lottery, and found that a black hole was headed straight for us? Despite the long odds, it’s astronomically possible, and given the fact that there are 400 billion stars in the Milky Way, a 1-in-400 million chance means that a black hole has probably “gotten” 1000 or so stellar systems over our planet’s existence.
Unfortunately, the only option, at that point, would be to take the Ludacris solution and get out of the way. We couldn’t move the Earth by itself; we’d have to move the entire Solar System to avoid the black hole, and the only thing that can move a Solar System by a substantial amount is, disappointingly, a gravitational interaction with another large mass. In other words, the only thing that could save us from an oncoming black hole is the very phenomenon we’re trying to avoid: significantly gravitationally perturbing the orbits of the planets in our Solar System. If this scenario were to become reality, the only sensible course of action, after an unbroken string of billions of years of continued life on our planet, would be to either abandon spaceship Earth, or otherwise resign ourselves to going down with the ship.