Ask Ethan: Why Was The Universe Dark For So Long?
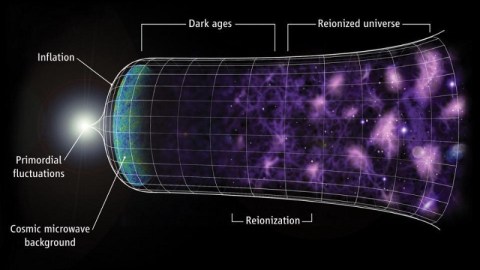
The first stars formed almost half a billion years before we could see their light. Here’s why.
At the moment of the Big Bang, the Universe was full of matter and radiation, but there were no stars. As it expanded and cooled, you formed protons and neutrons in the first fraction of a second, atomic nuclei in the first 3–4 minutes, and neutral atoms after about 380,000 years. After another 50–100 million years, you form the very first stars. But the Universe remains dark, and observers within it are unable to see that starlight, until 550 million years after the Big Bang. Why so long? Iustin Pop wants to know:
One thing I wonder though is why did the dark ages last hundreds of millions of years? I would have expected an order of magnitude smaller, or more.
Forming stars and galaxies is a huge step in the creation of light, but it isn’t enough to end the “dark ages” on its own. Here’s the story.
Try and imagine the Universe as it was when it was only a few minutes old: before the formation of neutral atoms. Space is full of protons, light nuclei, electrons, neutrinos, and radiation. Three important things happen at this early stage:
- The Universe is very uniform in terms of how much matter there is in any location, with the densest regions only a few parts in 100,000 more dense than the least dense regions.
- Gravitation works hard to pull matter in, with overdense regions exerting an extra, attractive force to make that happen.
- And radiation, mostly in the form of photons, pushes outwards, resisting the gravitating effects of the matter.
As long as we have radiation that’s energetic enough, it prevents neutral atoms from stably forming. It’s only when the expansion of the Universe cools the radiation enough that neutral atoms won’t immediately get reionized.
After this occurs, 380,000 years into the history of the Universe, that radiation (mostly photons) simply free-streams in whatever direction it was traveling last, through the now-neutral matter. 13.8 billion years later, we can view this leftover glow from the Big Bang: the Cosmic Microwave Background. It’s in the “microwave” part of the spectrum today because of the stretching-of-wavelengths due to the Universe’s expansion. But more importantly, there’s a pattern of fluctuations in there of hot-and-cold spots, corresponding to overdense and underdense regions of the Universe.
Once you form neutral atoms, it becomes much easier for gravitational collapse to ensue, since photons interact very easily with free electrons, but much less so with neutral atoms. As the photons cool to lower and lower energies, the matter becomes more important to the Universe, and so gravitational growth begins to occur. It takes roughly 50–100 million years for gravity to pull enough matter together, and for the gas to cool enough to allow collapse, so that the very first stars form. When they do, nuclear fusion ignites, and the first heavy elements in the Universe come into existence.
But even with those stars, we’re still in the dark ages. The culprit? All those neutral atoms spread throughout the Universe. There are some 1080 of them, and while the low-energy photons left over from the Big Bang are transparent to this normal matter, the higher-energy starlight is opaque. This is the same reason why you can’t see the stars in the galactic center in visible light, but at longer (infrared, for example) wavelengths, you can see right through the neutral gas and dust.
In order for the Universe to become transparent to starlight, these neutral atoms need to become ionized. They were ionized once a long time ago: before the Universe was 380,000 years old, so we call the process of ionizing them one more time reionization. It’s only when you’ve formed enough new stars, and emitted enough high-energy, ultraviolet photons, that you can complete this process of reionization and bring the dark ages to an end. While the very first stars may exist after just 50–100 million years after the Big Bang, our detailed observations have shown us that reionization doesn’t complete until the Universe is around 550 million years old.
How is it, then, that the earliest galaxies we see are from when the Universe was only 400 million years old? And how is it the case that the James Webb Space Telescope will see even farther back than that? There are two factors that come into play:
1.) Reionization is non-uniform. The Universe is full of clumps, imperfections, and inhomogeneities. This is great, as it allows us to form stars, galaxies, planets, and also human beings. But it also means that some regions of space, and some directions on the sky, experience total reionization before others. The farthest known galaxy we’ve ever seen, GN-z11, is a bright and spectacular galaxy for as young as it is, but it also happens to be located in a direction where the Universe is mostly already completely reionized. It’s mere serendipity that this occurred 150 million years before the “average” reionization time.
2.) Longer wavelengths are transparent to these neutral atoms. While the Universe is dark at these early times as far as visible and ultraviolet light goes, the longer wavelengths are transparent to those neutral atoms. For example, the “Pillars of Creation” are famously opaque to visible light, but if we view them in infrared light, we can easily see the stars inside.
The James Webb Space Telescope will not only be a primarily infrared observatory, but will be designed to view light that was infrared when it was emitted from these early stars. By extending out to wavelength of 30 microns, well into the mid-infrared, it will be able to view objects during the dark ages themselves.
The Universe was dark for so long because the atoms within it were neutral for so long. Even a 98% reionized Universe is still opaque to visible light, and it takes roughly 500 million years of starlight to completely ionize all the atoms and give us a Universe that’s truly transparent. When the dark ages end, we can see everything in all wavelengths of light, but prior to that, we need to either get lucky or look in longer, less-well-absorbed wavelengths.
Letting there be light, by forming stars and galaxies, isn’t enough to end the dark ages in the Universe. Creating light is only half the story; creating an environment where it can propagate all the way to your eyes is just as important. For that, we need lots of ultraviolet light, and that requires time. Yet by looking in just the right way, we can peer into the darkness, and see what we’ve never observed before. In less than two years, that story will begin.
Send in your Ask Ethan questions to startswithabang at gmail dot com!
Ethan Siegel is the author of Beyond the Galaxy and Treknology. You can pre-order his third book, currently in development: the Encyclopaedia Cosmologica.