Ask Ethan: Why do stars come in different sizes?
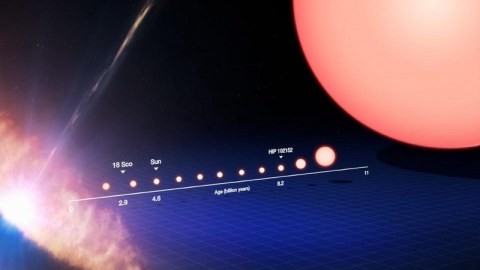
More massive is bigger, less massive is smaller, right? That’s not even half the story.
“Billions of years from now our sun, then a distended red giant star, will have reduced Earth to a charred cinder.” –Carl Sagan
If you were to compare planet Earth to the Sun, you’d find that you’d have to stack 109 Earths atop one another just to go from one end of the Sun to the other. Yet there are stars out there that are much smaller than the Earth… and much, much larger than even Earth’s orbit around the Sun! How is this possible, and what determines a star’s size? That’s what Roman Stangl wants to know:
Why can suns grow to… many different sizes? That is, ranging from somewhat larger [than] Jupiter up to suns exceeding Jupiter’s orbit?
It’s a tougher question than you think, because for the most part, we can’t see the size of a star.
Even through a telescope, most stars appear as simple points of light due to their incredible distances from us. Their differences in color and brightness are easy to see, but size is a different matter entirely. An object of a certain size a specific distance away will have what’s known as an angular diameter: the apparent size it appears to take up on the sky. The closest Sun-like star, Alpha Centauri A, is just 4.3 light years away, and is actually 22% larger than the Sun in radius.
Yet it appears to us to have an angular diameter of just 0.007″, or arc-seconds, where it takes 60 arc-seconds to make one arc-minute, 60 arc-minutes to make 1 degree, and 360 degrees to make a full circle. Even a telescope like Hubble can only resolve down to about 0.05″; there are very few stars in the Universe that a telescope can actually resolve. These tend to be giant stars that are close by, like Betelgeuse or R Doradus, which are among the largest stars in angular diameter in the entire sky.
Fortunately, there are indirect measurements that allow us to calculate the physical size of a star, and those are incredibly reliable. If you have a spherical object that gets so hot it emits radiation, the total amount of radiation emitted by the star is determined by only two things: the temperature of the object and its physical size. The reason for this is that the only place that emits light out into the Universe is the star’s surface, and the surface area of a sphere always follows the same formula: 4πr2, where r is the radius of your sphere. If you can measure the distance to that star, its temperature, and how bright it appears, you can know its radius (and hence, its size) just by applying the laws of physics.
When we make our observations, we see that some stars are as small as only a few tens of kilometers in size, while others go all the way up to more than 1,500 times the size of our Sun. Of the supergiant stars, the largest one known is UY Scuti at around 2.4 billion kilometers in diameter, which is larger than Jupiter’s orbit around the Sun. The thing is, these extreme examples of stars aren’t for stars like our Sun at all. Sure, the most common type of star is a main-sequence star like our Sun: a star made out of mostly hydrogen that gets its energy by fusing hydrogen into helium in its core. And these do come in a very large variety of sizes, determined by the mass of the star itself.
When you form a star, gravitational contraction results in the conversion of potential energy (gravitational potential energy) into kinetic energy (the heat/motion) of particles in the star’s core. If there’s enough mass, the temperature can get high enough to ignite nuclear fusion in the innermost regions, as hydrogen nuclei undergo a chain reaction to convert into helium. In a low-mass star, only a tiny portion of the very center will hit that threshold of 4,000,000 K and undergo fusion, and that will be at a very slow rate. On the other hand, the largest stars can be hundreds of times as massive as the Sun, and achieve core temperatures of many tens of millions of degrees, fusing hydrogen into helium at rate that are millions of times as large as our Sun’s.
The smallest stars, in this sense, have the smallest outward fluxes and radiation pressures, while the most massive stars have the largest ones. This outward radiation and energy is what holds the star up against gravitational collapse, but it might surprise you to learn the range is relatively narrow. The lowest-mass red dwarf stars, like Proxima Centauri and VB 10 are only 10% the size of the Sun; a little larger than Jupiter. On the other hand, the largest blue giant, R136a1, is over 250 times the Sun’s mass… but only about 30 times the Sun’s diameter. If you’re fusing hydrogen into helium, your star isn’t going to vary in size by all that much.
But not every star is fusing hydrogen into helium! The smallest stars aren’t fusing anything at all, while the largest ones are onto a far more energetic phase of their lives. We can break down the types of stars we have by size range, and what we find are five generic classes:
- Neutron stars: these supernova remnants contain the mass of one-to-three suns, but are basically compressed into one giant atomic nucleus. They still emit radiation, but only in tiny amounts due to their minuscule size. A typical neutron star is around 20–100 km in size.
- White dwarf stars: formed when a sun-like star runs out of the last of its helium fuel in its core, and the outer layers get blown off while the inner layers contract down. Typically, a white dwarf star has between 0.5 and 1.4 times the mass of the Sun, but is only the physical volume of Earth: around 10,000 km across, and is made out of highly compressed atoms.
- Main sequence stars: these include the red dwarfs, the sun-like stars, and the blue behemoths we talked about earlier. Ranging from about 100,000 km to 30,000,000 km, they cover a fairly wide set of sizes, but even the largest one, if it replaced the Sun, wouldn’t engulf Mercury.
- Red giant stars: so what happens when you run out of hydrogen in your core? If you’re not a red dwarf (in which case, you’ll just turn into a white dwarf), gravitational contraction will heat your core up so much that you’ll begin fusing helium into carbon. Oh, and fusing helium into carbon releases way more energy than plain old hydrogen fusion, causing your star to expand tremendously. The simple physics is that the outward force (radiation) at the edge of the star has to balance the inward force (gravitation) in order to keep your star stable, and with a much greater outward force, your star simply has to be much larger. Red giants are typically around 100–150,000,000 km in diameter: large enough to engulf Mercury, Venus, and possibly Earth.
- Supergiant stars: the most massive stars will go beyond helium fusion and begin fusing even heavier elements in their cores, like carbon, oxygen, and even silicon and sulfur. These stars are destined for supernova and/or black hole fates, but before they get there, they swell to tremendous sizes that can extend for a billion (1,000,000,000) kilometers or more. These are the largest stars of all, like Betelgeuse, and would engulf all of the rocky planets, the asteroid belt, and the biggest ones would even swallow up Jupiter if they were to replace our Sun.
For the tiniest stars of all, the remnants like neutron stars and white dwarfs, it’s the fact that their trapped energy can only escape through a tiny surface area that keeps them so bright for so long. But for all other stars, their sizes are determined by that simple balance: the force from the outward radiation, at the surface, has to equal the inward pull of gravitation. Larger radiation forces means the star swells to larger sizes, with the largest stars of all swelling to billions of kilometers.
In fact, as the Sun ages, its core heats up, and it both expands and grows hotter over time. In a billion or two years, it will be hot enough that it should boil Earth’s oceans, unless we do something to migrate our planet’s orbit outward to safety. Give it enough time, and we’ll swell to a red giant ourselves. For a few hundred million years, we’ll be larger and brighter than some of the most massive stars of all. As impressive as that may be, don’t be fooled: size matters in astronomy, but it isn’t the only thing. Both the smallest neutron stars and the largest supergiants, as well as many white dwarfs and main sequence stars, will still be more massive that we will as a red giant!
Send in your Ask Ethan submissions to startswithabang at gmail dot com!
Ethan Siegel is the author of Beyond the Galaxy and Treknology. You can pre-order his third book, currently in development: the Encyclopaedia Cosmologica.