Ask Ethan: Where Does The ‘Energy’ For Dark Energy Come From?

Perhaps energy isn’t conserved at all in an expanding Universe.
If you have a Universe full of stuff — whether that’s atoms, dark matter, radiation, neutrinos, or anything else — it’s virtually impossible to keep it static. The fabric of your Universe, at least in General Relativity, must either expand or contract on the largest scales. But if you have a Universe filled with dark energy, as we appear to have, something even more troubling happens: the total amount of energy contained within our observable Universe increases over time, with no end in sight. Doesn’t this violate the conservation of energy? That’s what David Ventura wants to know, as he asks:
[T]he total energy of the universe is increasing such that the energy inherent of space-time is kept constant as the universe expands. It is like, in order to build an extra cubic kilometer of space-time you need this quanta of energy. No more and no less. This energy has to come from somewhere. In everything else I know of, energy (including matter via E = mc²), cannot just appear from nowhere. So something must be giving energy into our universe to cause it to expand. […] Will it ever stop?
The actual, scientific truth of what’s going on is much more troubling than you might imagine.
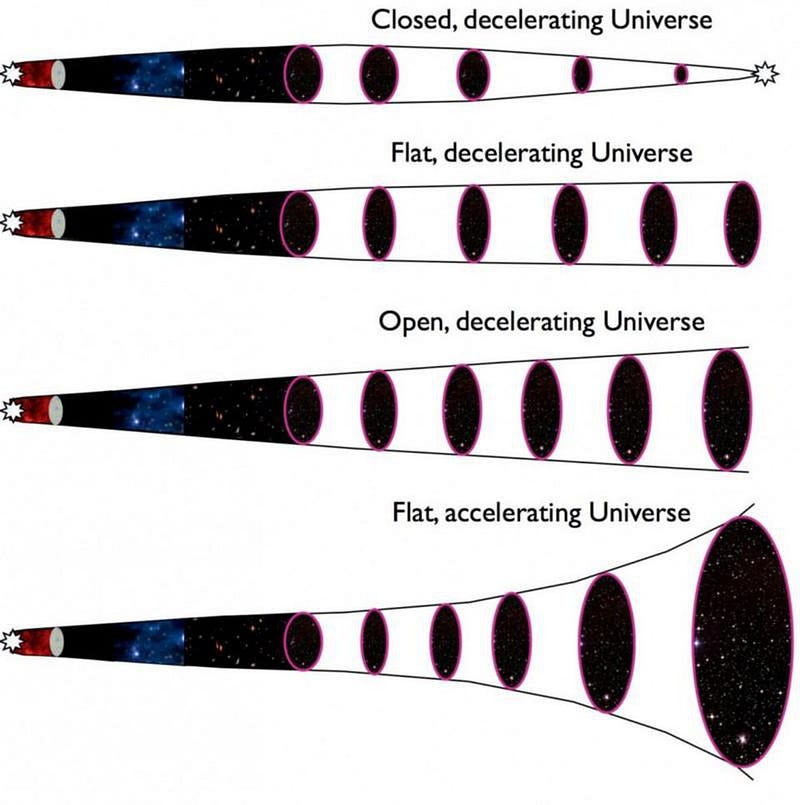
In our physical Universe, there are two things that are inextricably linked together: the expansion rate of the Universe and the breakdown of all the different types of energy present within it. The cardinal rule of General Relativity is that matter tells space how to curve, while curved space tells matter how to move. This is true, but it’s not complete. It isn’t just matter but also energy that affects the curvature of space, and it isn’t simply curvature but also the expansion (or contraction) rate of space that gets affected. In particular, it’s the energy density that determines the expansion rate.
But there are different forms of energy in the Universe, and they each play slightly different roles in how the expansion rate changes over time.

For something like normal matter, its energy contributions are actually intuitive. Matter is made of particles that contain mass, and even as the Universe changes, the individual particles themselves remain the same. Over time, the volume of the Universe increases, and as it does, the total matter density drops. Density is mass over volume: mass remains the same, volume increases, and so the density goes down. If all we had in the Universe was matter, the expansion rate would drop as the matter density dropped.
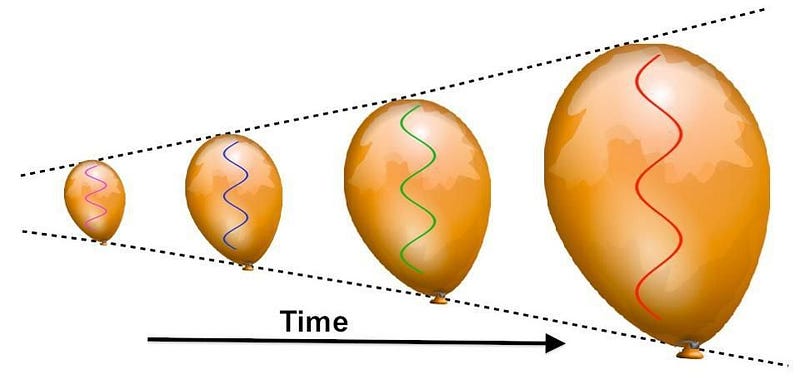
For radiation, there’s an extra component to it. Sure, radiation is also made of particles, and as the volume expands, the number density of those particles decreases just as it does for matter. But radiation has a wavelength, and that wavelength gets stretched by the expanding Universe. Longer wavelengths mean lower energies, and so the expansion rate drops faster in a radiation-filled Universe than in a matter-filled one.
But for a Universe filled with dark energy, the story is very different. Dark energy is caused by energy inherent to the fabric of space itself, and as the Universe expands, it’s the energy density — the energy-per-unit-volume — that remains constant. As a result, a Universe filled with dark energy will see its expansion rate remain constant, rather than drop at all.
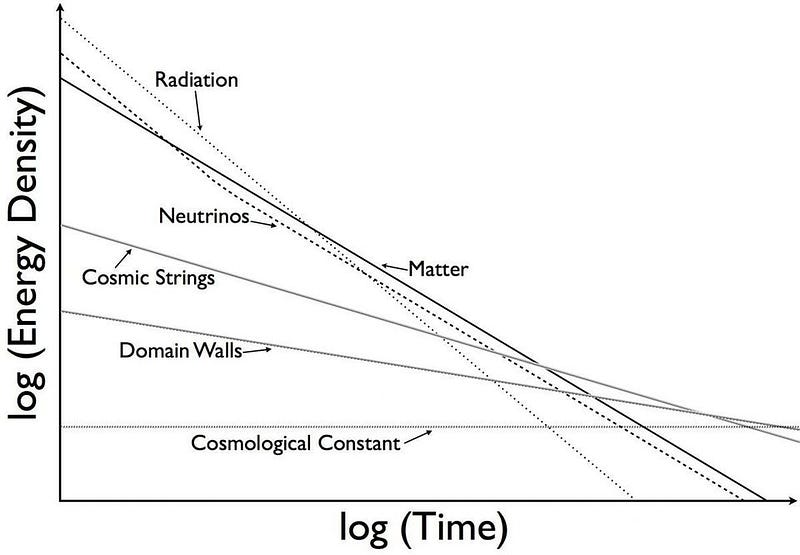
“Hang on,” you might object, thinking, “I thought you said the Universe’s expansion was accelerating?”
There’s a very important point here that doesn’t get emphasized enough: there are two different things scientists talk about when it comes to the expansion of the Universe. One is the expansion rate — or the Hubble rate — of the Universe. This behaves exactly as we described above: it drops for matter, it drops faster for radiation, and it asymptotes to a positive constant for dark energy. But the second thing is how quickly an individual galaxy appears to recede from us over time.
As time goes on, a galaxy gets farther and farther away from us. Since the expansion rate is a speed-per-unit-distance (e.g., 70 km/s/Mpc), a galaxy that’s farther away (say, 100 Mpc vs. 10 Mpc) will appear to recede at a faster speed (7,000 km/s vs. 700 km/s). If your Universe is filled with matter or radiation, the expansion rate drops faster than your galaxy’s distance increases, so the net recession speed will drop over time: your Universe will be decelerating. If your Universe is dominated by dark energy, however, the net recession speed will increase over time: your Universe is accelerating.
Our Universe, today, is made of approximately 68% dark energy. Starting at around 6 billion years ago, our Universe made the switch to accelerating from decelerating, based on the balance of all the different things within it.
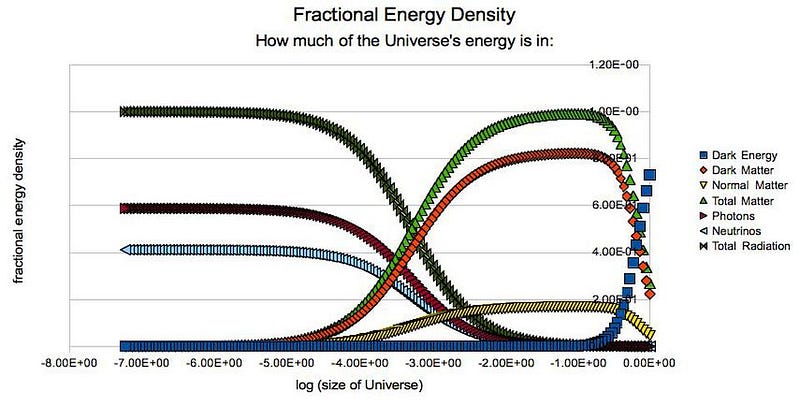
But how is this okay? It seems like a Universe filled with dark energy doesn’t conserve energy. If the energy density — energy-per-unit-volume — remains constant, but the volume of the Universe is increasing, doesn’t that mean the total amount of energy in the Universe is increasing? And doesn’t that violate the conservation of energy?
This should bother you! After all, we think that energy should be conserved in any and all physical processes that take place in the Universe. Does General Relativity offer a possible violation of energy conservation?
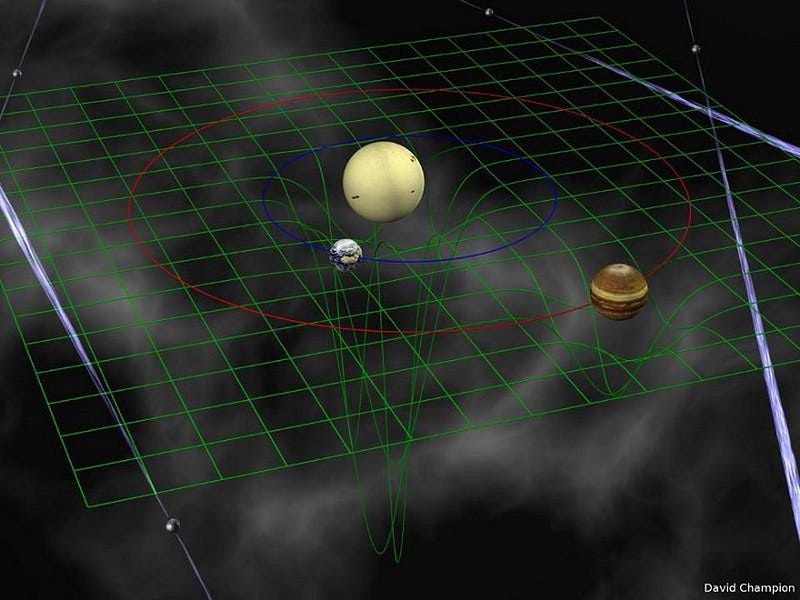
The scary answer is maybe, actually. There are a lot of quantities that General Relativity does an excellent and precise job of defining, and energy is not one of them. In other words, there is no mandate that energy must be conserved from Einstein’s equations; global “energy” is not defined by General Relativity at all! In fact, we can make a very general statement about when energy is and isn’t conserved. When you have particles interacting in a static background of spacetime, energy is truly conserved. But when the space through which particles move is changing, the total energy of those particles is not conserved. This is true for photons redshifting in an expanding Universe, and it’s true for a Universe dominated by dark energy.
But that answer, though technically correct, isn’t the end of the story. We can come up with a new definition for energy when the space is changing; but we have to be careful when we do.
There is a very smart way of looking at “energy” that allows us to show, in fact, that energy is conserved even in this seemingly paradoxical situation. I want you to remember that, in addition to chemical, electrical, thermal, kinetic, and potential energies, among others, there’s also work. Work, in physics, is when you apply a force to an object in the same direction as the distance it moves; this adds energy to the system. If the direction is opposite, you do negative work; this subtracts energy from the system.
A good analogy is to think of gas. What happens if you heat up (add energy to) that gas? The molecules inside move faster as they gain energy, meaning they increase their speed, and they spread out to take up more space more quickly.
But what happens, instead, if you heat up gas that’s enclosed in a container?
Yes, the molecules heat up, they move faster, and they try to spread out, but in this case, they often run into the walls of the container, creating an extra positive pressure on the walls. The container’s walls are pushed outward, which costs energy: the molecules are doing work on it!
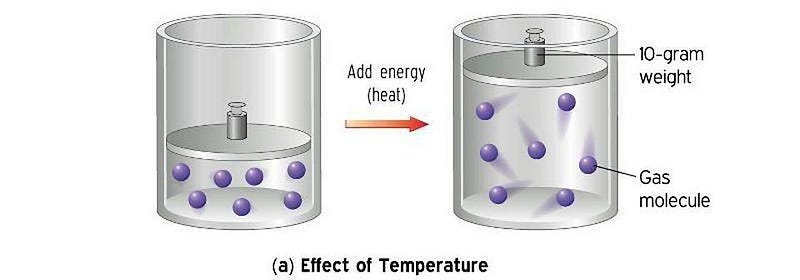
This is very, very analogous to what happens in the expanding Universe. If your Universe were filled with radiation (photons), each quantum would have an energy, given by a wavelength, and as the Universe expands, that photon wavelength gets stretched. Sure, the photons are losing energy, but there is work being done on the Universe itself by everything with a pressure inside of it!
Conversely, if your Universe were filled with dark energy, it also has not only an energy density, but a pressure, too. The big difference, though, is that the pressure from dark energy is negative, which means we have the opposite situation we had for radiation. As the container’s walls expand, they’re doing work on the fabric of space itself!
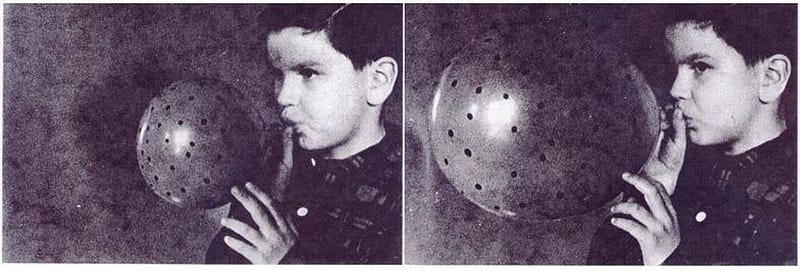
So where does the energy for dark energy come from? It comes from the negative work done on the expansion of the Universe itself. There was a paper written in 1992 by Carroll, Press, and Turner, which dealt with this exact issue. In it, they state:
…the patch does negative work on its surroundings, because it has negative pressure. Assuming the patch expands adiabatically, one may equate this negative work to the increase of mass/energy of the patch. One thereby recovers the correct equation of state for dark energy: P = — ρc². So the mathematics is consistent.
Which, again, still doesn’t mean that energy is conserved. It simply gives us an intelligent way to look at this problem.
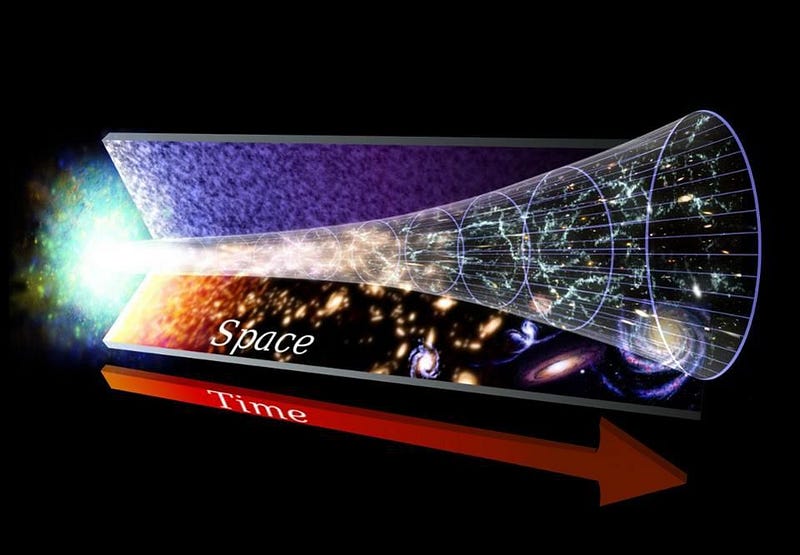
This is one of the deepest cosmology questions I’ve ever fielded for Ask Ethan. The two major takeaways are as follows:
- When particles interact in an unchanging spacetime, energy must be conserved. When the spacetime they’re in changes, that conservation law no longer holds.
- If you redefine energy to include the work done, both positive and negative, by a patch of space on its surroundings, you can save the conservation of energy in an expanding Universe. This is true for both positive-pressure quantities (like photons) and negative pressure ones (like dark energy).
But this redefinition is not robust; it’s simply a mathematical redefinition we can use to force energy to be conserved. The truth of the matter is that energy is not conserved in an expanding Universe. Perhaps in a quantum theory of gravity, it will be. But in General Relativity, we have no good way of defining it at all.
Send in your Ask Ethan questions to startswithabang at gmail dot com!
Ethan Siegel is the author of Beyond the Galaxy and Treknology. You can pre-order his third book, currently in development: the Encyclopaedia Cosmologica.