Ask Ethan: What Is The ‘Zero-Point Energy’ Of Space?
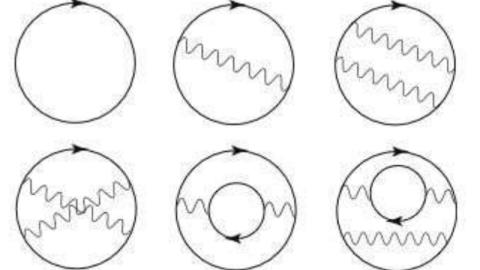
Even if you remove everything you can from the Universe, some energy will still remain. Here’s what that means.
Imagine, if you can, what it would mean to have a Universe with absolutely nothing in it. You could take all the various quanta of matter and energy and remove them, leaving a Universe with no particles or antiparticles of any type. You could remove any sources of gravitation or spatial curvature, reducing your Universe to nothing more than purely empty space. You could shield the Universe from any external electric, magnetic, or fields that exert a nuclear force, eliminating any possible influence they may have on the spacetime you’re considering. Even if you do all that, you still wouldn’t get “zero” on your balance sheet for the energy of the Universe. That’s what Niels Hermes wants to know, as he writes in to ask:
Would it be possible to shine a light on the concept of zero point energy?
It’s a challenging concept, but let’s take a crack at it.
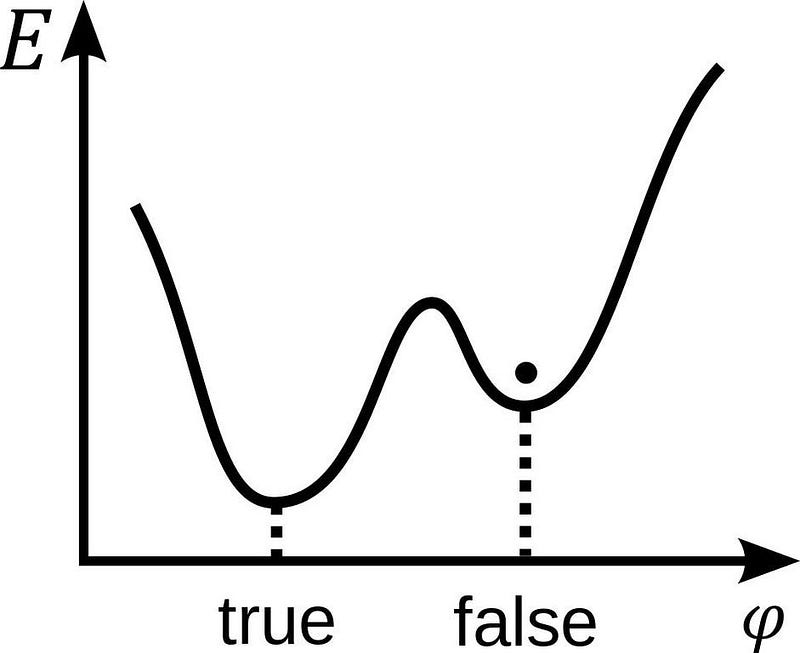
For any physical system we can dream up, there’s always going to be at least one configuration that we can place it in that will have the lowest total amount of energy. For a series of masses isolated from the rest of the Universe, that’s a black hole. For a proton and an electron, that’s a hydrogen atom in the ground (i.e., lowest-energy) state. And for the Universe itself, that’s to set up empty space in the absence of any external fields or sources.
That lowest-energy state is known as the zero-point energy state. For a long time, scientists who studied the Universe assumed that the zero-point energy was zero. Not for any physical reason, mind you, but because we only had two ways to attempt to arrive at it, and both of them gave answers that pointed to problems with any value other than zero.
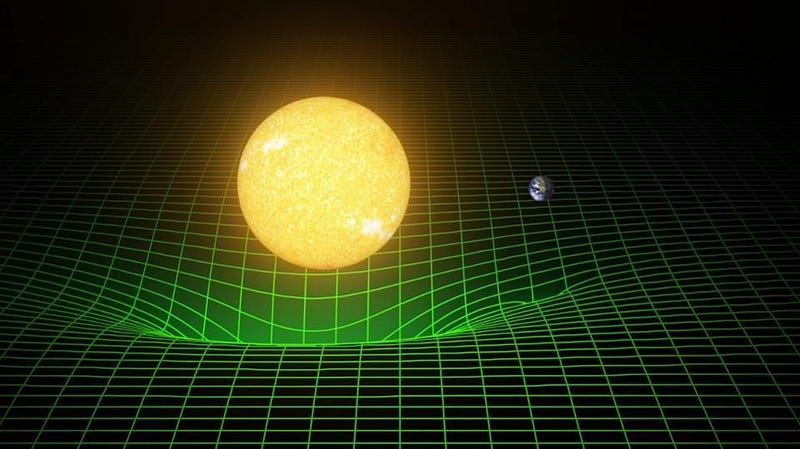
The first time the idea of a zero-point energy to space arose was in the context of Einstein’s (then-new) theory of gravity: General Relativity. According to Einstein, the curvature of space determines the future behavior of matter and energy in the Universe, and the presence of matter and energy determines the curvature of space.
Well, almost. The presence of matter and energy determines the curvature of space almost completely, but you are free to add a constant to space itself. (Those of you who’ve taken calculus will recognize this constant as something that arises whenever you do an integral: you determine the answer completely, up to an additive constant, “plus c.”) That constant, whatever it is, represents the zero-point energy of space. When we discovered the expanding Universe, the constant was completely unnecessary, and was thrown away as such for some 60+ years.
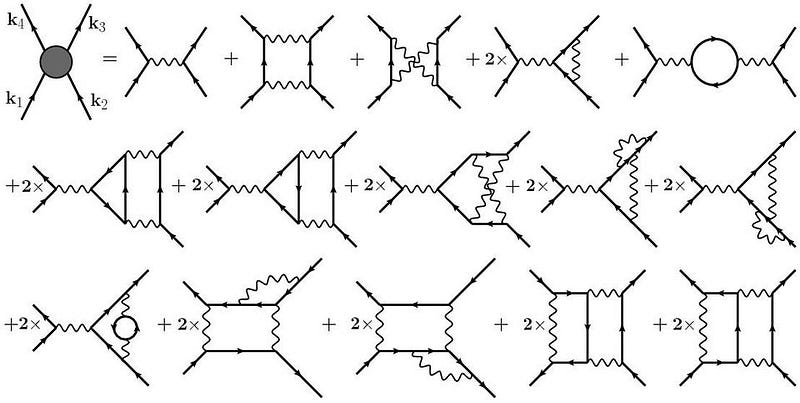
The second time the idea of zero-point energy showed up was when quantum field theory rose to prominence. In addition to all of the ways that particles could interact with one another through the quantum fields permeating the Universe, there were also “vacuum” contributions, which represented how quantum fields in the vacuum of space behaved.
Individual channels contributed enormous amounts to what we called the “vacuum expectation value” of these fields, which were typically some ~120 orders of magnitude greater than the observational limits. But some were positive and some were negative, and many assumed that they’d all cancel out. Additionally, some field theories were proven to be exactly equivalent to free theories (whose vacuum expectation values were zero), and so again, we assumed that the zero-point energy was zero.
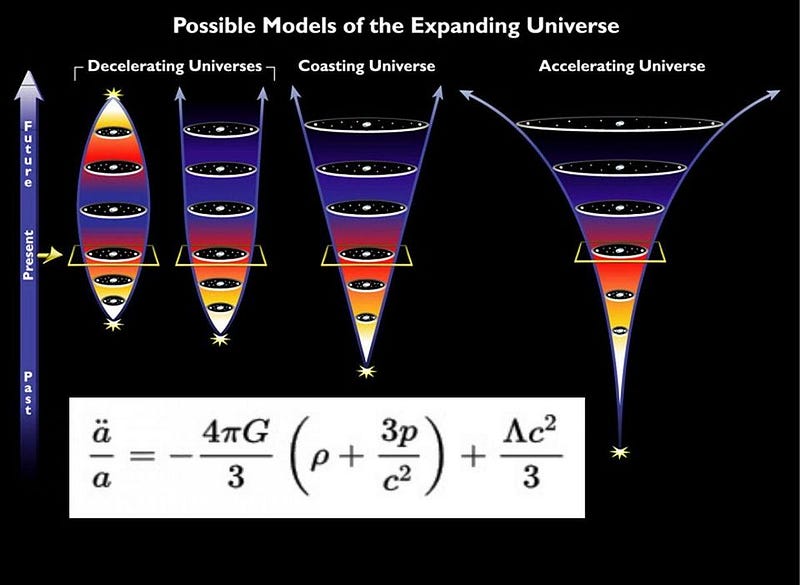
And then, at the very end of the 20th century, the unthinkable happened. We had always anticipated that the Universe was expanding, that gravity was working to slow the expansion, and that either:
- gravitation would win and the expansion would reverse,
- the expansion would win and continue to slow forever and ever,
- or they would balance exactly, and the expansion would asymptote to zero but never quite reverse.
But then we discovered that the expansion of the Universe wasn’t slowing down at all, but that distant galaxies were receding from us faster and faster as time went on. The Universe didn’t just have matter and radiation in it, but appeared to have a new form of energy in it: what we now call dark energy. In the 22 years since that first discovery occurred, not only has dark energy been confirmed by many lines of evidence, but it’s been demonstrably shown to be indistinguishable, to great precision, from a cosmological constant.
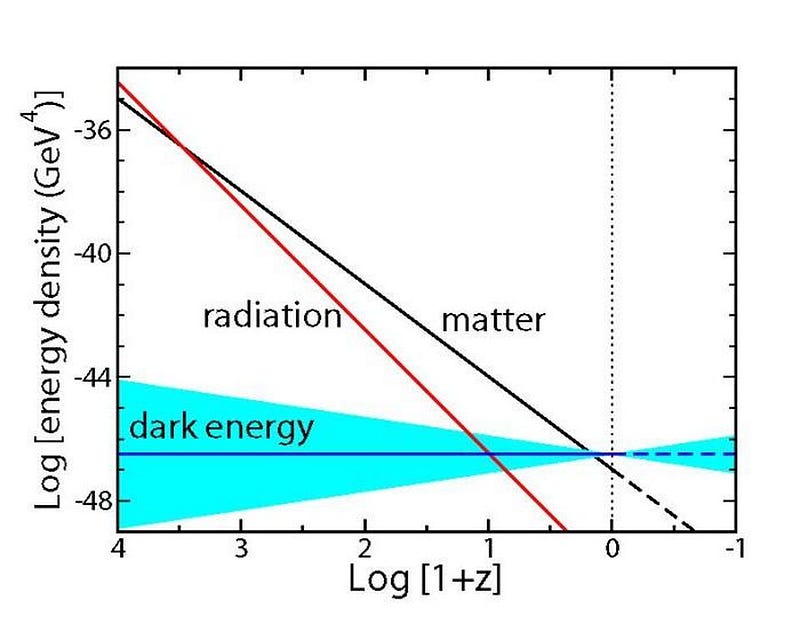
This is why we care about the zero-point energy of space. Observations from many lines of evidence — including the cosmic microwave background, distant sources of light (like supernovae), and the clustering of galaxies in the Universe — all point to the same tiny, non-zero value of the amount of dark energy in the Universe. It appears to be a form of energy inherent to space itself, it appears not to change with time, it appears to be of a constant density everywhere and always, and we don’t know what’s causing it.
That’s why we have such a strong motivation to try and understand what the zero-point energy of space is: precisely because we measure the Universe’s expansion, which depends on it, to be inconsistent with a value of zero for this quantity. Just as hydrogen atoms have a finite energy to their ground state, so must the ground state energy of empty space itself.
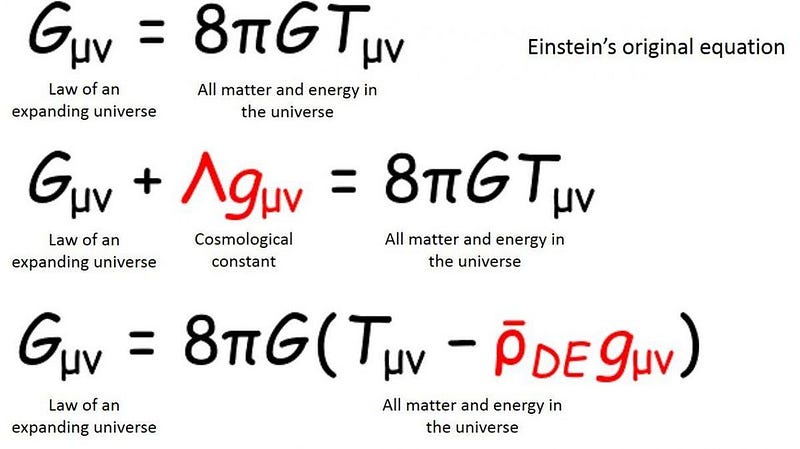
That brings us to the big question: why? Why is the zero-point energy of space the value that it is? There are many plausible answers, but each one of them is dissatisfying in some way.
It could be that the cosmological constant from General Relativity simply has the positive value that it does. It’s allowed to take on any value, and everything that we observe is consistent with the zero-point energy of space having a small, constant, positive value since the start of the hot Big Bang. This is appealing because it doesn’t need to invoke any new physics: we can explain what we observe by setting one free parameter equal to the correct observed value. But it’s dissatisfying because there’s no mechanism or reasoning to help us understand why it has the value that it does.
Alternately, it could be that the zero-point energy of all the quantum fields permeating the Universe sum up to the observed value required for dark energy. Perhaps, if we knew how to calculate this value correctly, we’d arrive at the correct answer.
The problem with this scenario is that we don’t know how to do this calculation, and all of our attempts give us an answer that’s ridiculously too large. It’s possible that there’s an almost-perfect-but-not-quite cancellation that will occur, leading us to the correct value, but that’s a tough proposition to bet on. “We don’t know how to do this, and the task seems difficult but isn’t proven to be impossible,” isn’t exactly a compelling line of thought.
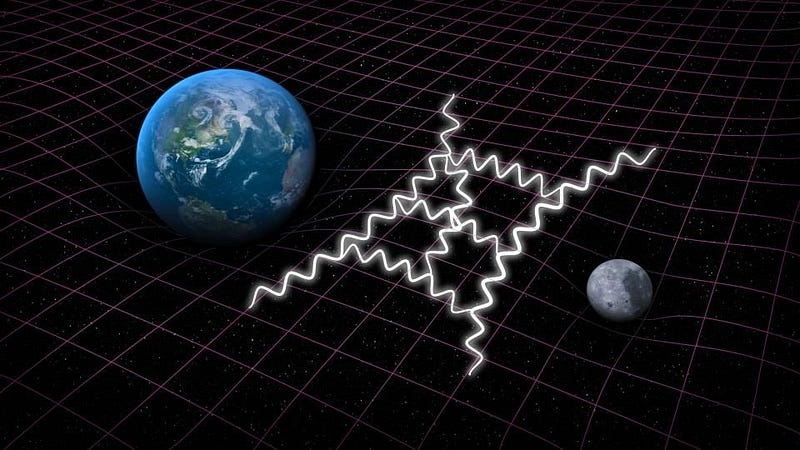
But there are always “novel physics” scenarios to consider as well. It’s possible that there’s no cosmological constant and no contribution to the zero-point energy from the quantum fields we know. We could instead postulate a new type of field in the Universe, which could be:
- a contribution from whatever quantum theory of gravity turns out to be correct,
- a leftover relic from an earlier broken symmetry in the Universe (from the grand unification scale, the Higgs scale, the neutrino sector, etc.) that simply sets the zero-point energy to its presently non-zero value,
- that there’s a relic amount of energy that didn’t quite go to zero from our earlier inflationary epoch,
- or that the highly speculative idea of the string landscape, which itself requires many unproven, evidence-free assumptions about how the Universe behaved prior to the hot Big Bang, simply “landed on the value we see today” for the value of the zero-point energy (or vacuum expectation value) of empty space.
In the absence of a solution, all possibilities — no matter how ill-motivated they appear — ought to be considered.
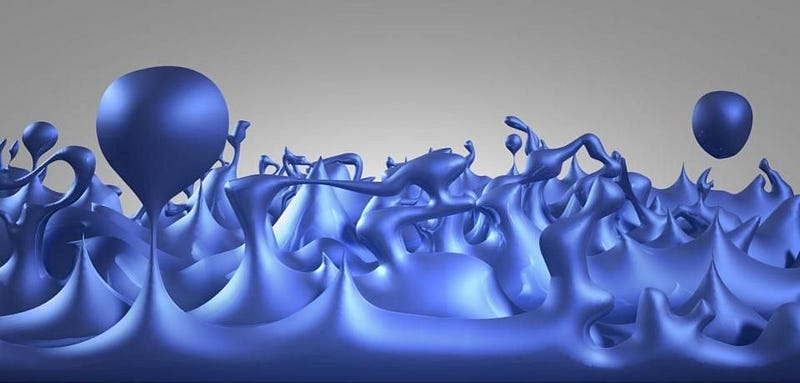
But no matter what the answer to the zero-point energy puzzle is, there are two facts we cannot deny. The first is that dark energy is real, corroborated by a slew of independent lines of evidence that our Universe simply cannot do without. It is consistent with having a constant value everywhere in space and throughout time: it behaves in a fashion that’s indistinguishable from space having a non-zero, constant zero-point energy.
The second fact is that, whatever the solution is, we still must reckon with the presence of quantum fields — mandated by the laws of physics — permeating our Universe. Until we know how to calculate that value, any proposed solution requires that we make an unfounded assumption for whatever that value is. The zero-point energy of empty space is inconsistent with a value of zero. There are many possible origins of this non-zero value, but its ultimate cause still remains a mystery.
Send in your Ask Ethan questions to startswithabang at gmail dot com!
Ethan Siegel is the author of Beyond the Galaxy and Treknology. You can pre-order his third book, currently in development: the Encyclopaedia Cosmologica.