Ask Ethan: What Happens When Stars Pass Through Our Solar System?
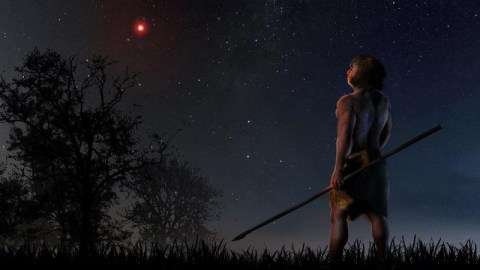
A recent study points to the possibility that just 70,000 years ago, a star passed through our Solar System. How often does this happen, and what are the consequences?
We like to think of our Solar System as a stable, mostly quiet place. Sure, we’ll find that the planets and other bodies in their orbits will kick around a comet or asteroid every once in a while, but for the most part, things are stable. Even the occasional interstellar visitor doesn’t pose much of a risk, at least, not to the integrity of worlds like our own. But our entire Solar System is orbiting through the galaxy, and that means it has hundreds of billions of chances to have a close interaction with another star. How often do we actually get one, and what are the potential consequences? That’s what our Patreon supporter Paweł Zuzelski wants to know (edited for English), as he asks:
How bad would it be if a star passed near the Sun? How close/large would it have to be to pose serious danger? How likely would such an event be?
The possibilities range from the mundane, where a few Oort cloud objects get thrown around, to the catastrophic, such as a collision with or ejection of an entire planet. Let’s take a look at what actually transpires.
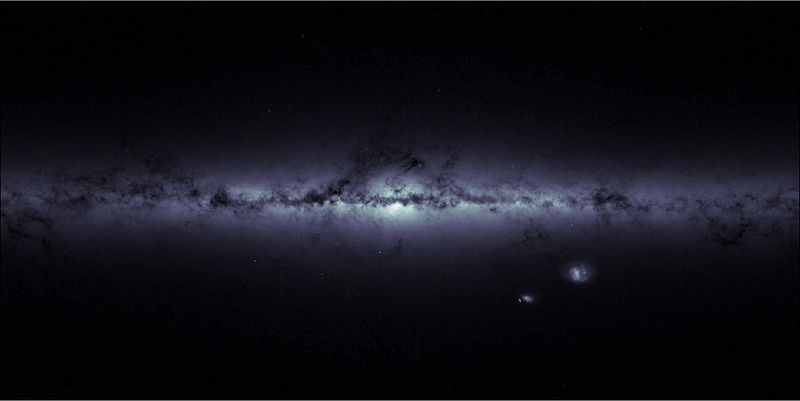
Our best estimates are that there are between 200 and 400 billion stars in our own Milky Way galaxy. Although stars come in a huge variety of sizes and masses, the majority of stars (around 3 out of every 4) are red dwarf stars: somewhere between 8% and 40% the mass of our Sun. These stars have similarly smaller physical sizes than our Sun: on average, about 25% the Sun’s diameter. And finally, we know roughly how big the Milky Way is: a disk around 2,000 light years thick, around 100,000 light years across, and with a central bulge that’s around 5,000–8,000 light years in radius.
Finally, relative to the Sun, the typical star moves at a speed of around 20 km/s: about 1/10th of the speed that the Sun (and all the stars) orbit through the Milky Way itself.
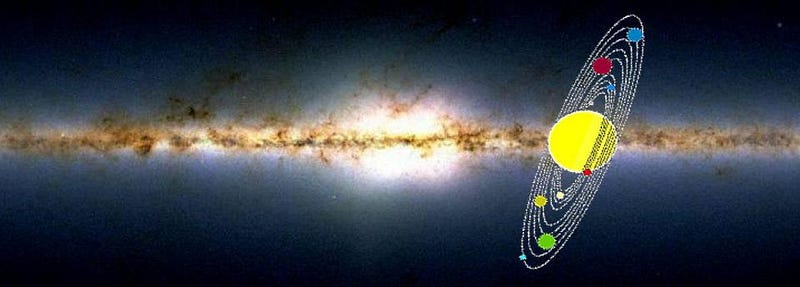
These are the stats on the stars within our galaxy. There are plenty of details, caveats, and nuances that we are ignoring here, like the density changing with respect to whether we’re in a spiral arm or not, the fact that there are more stars towards the center than the outskirts (and our Sun is mid-way towards the edge), the inclination of the orbits in our Solar System with respect to the galaxy, and slight changes dependent on whether we’re in the center of the galactic plane or not. But the reason we can ignore them is that just from the above approximation, these numbers allow us to calculate how often stars from the galaxy come within a specific distance of our Sun, and therefore, how often we can expect a close encounter of various impacts.
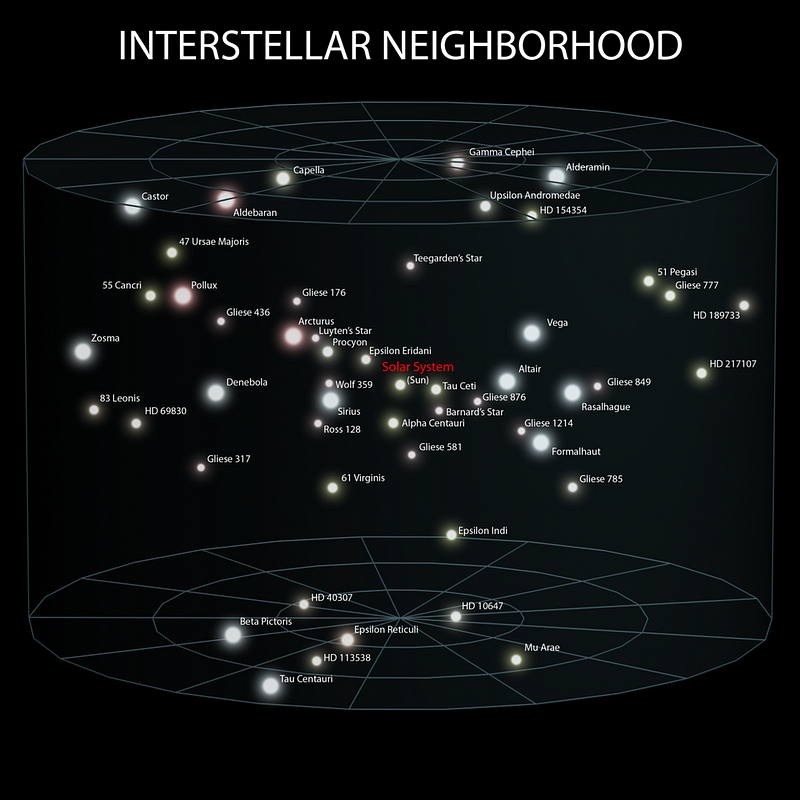
The way we compute it is very simple: we calculate the number density of stars, the cross-section we’re interested in (defined by how close you want another star to get to our own), and the speed with which the stars move relative to each other, and then multiply them all together to get the collision rate. This method of computation for a collision rate is useful for everything from particle physics to condensed matter physics (for the experts, this is basically the Drude model), and it applies just as readily to astrophysics. If we assume there are 200 billion stars in the Milky Way, that the stars are evenly distributed throughout the disk (and we ignore the bulge), and that 20 km/s is the speed that the stars move relative to one another, here’s what we get if we plot the interaction rate vs. the distance from the Sun.
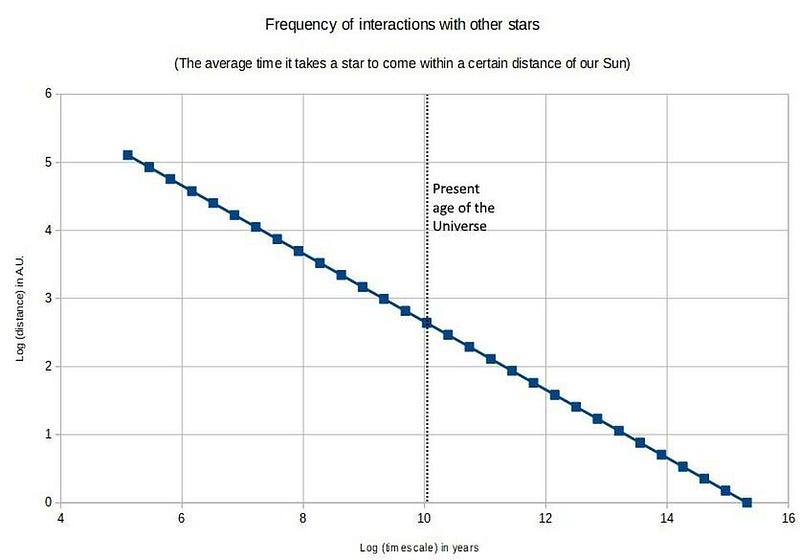
It tells us that, on average, the closest we can expect a star to have come to the Sun over the history of the Universe is around 500 A.U., or about ten times the distance from the Sun to Pluto. It tells us that, once every billion years, we can expect a star to come within about 1,500 A.U. of the Sun, close to the edge of the scattered Kuiper belt. And more frequently, about once every 300,000 years or so, we’ll get a star that comes within about a light year of us.
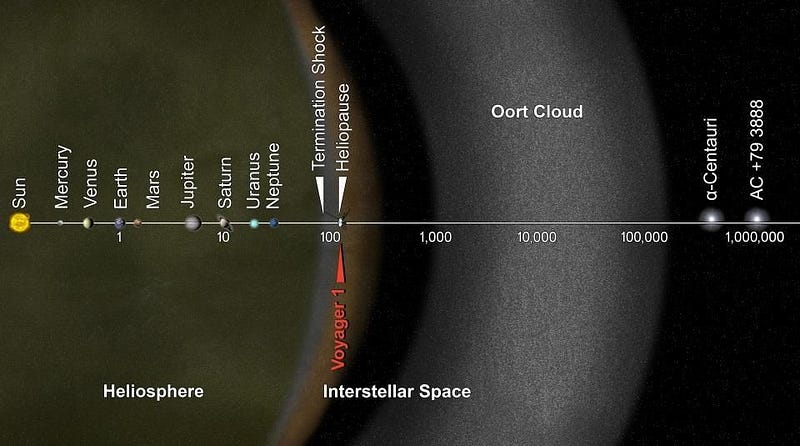
This is good for the long-term stability of the planets in our Solar System, for certain. It tells us that over our Solar System’s 4.5 billion year history, the odds that a star would come as close to any of the planets as our Sun is to Pluto is approximately 1-in-10,000; the odds that a star would come as close to a planet as the Sun is to Earth (which would severely disrupt an orbit and cause an ejection) is less than 1-in-1,000,000,000. It means that the likelihood of another star in the galaxy passing by us and causing us severe difficulties is terrifically low. We cannot bet on us losing the cosmic lottery, and the odds are that we haven’t lost it so far, and won’t for the foreseeable future.
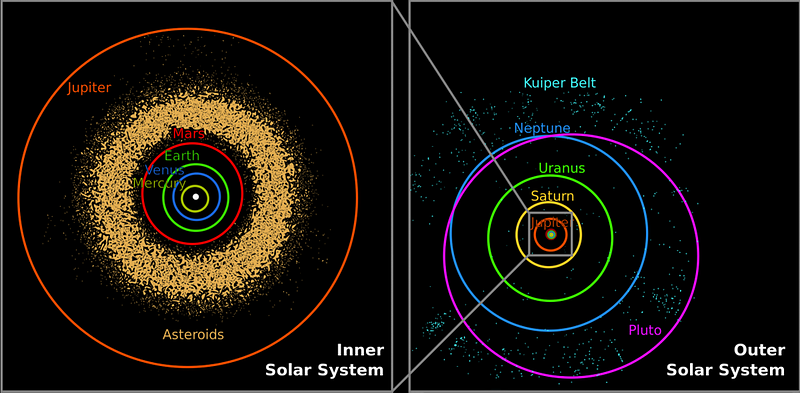
But there have probably been upwards of 40,000 times that a star has passed through the Oort cloud (defined as 1.9 light years from the Sun), disrupting a large number of icy bodies in the process. Stars are interesting when they pass through the Solar System like this, because of the combination of two factors:
- Oort cloud objects are very loosely bound to the Solar System, meaning that a very small gravitational tug is enough to alter their orbits significantly.
- Stars are very massive, so a star that passes the same distance from an object as that object is from the Sun can “kick” it enough to alter its orbit.
This tells us that whenever we do experience a close encounter with a passing star, we’re at an increased risk, for perhaps the next few million years, of a collision with an incoming object from the Oort cloud.
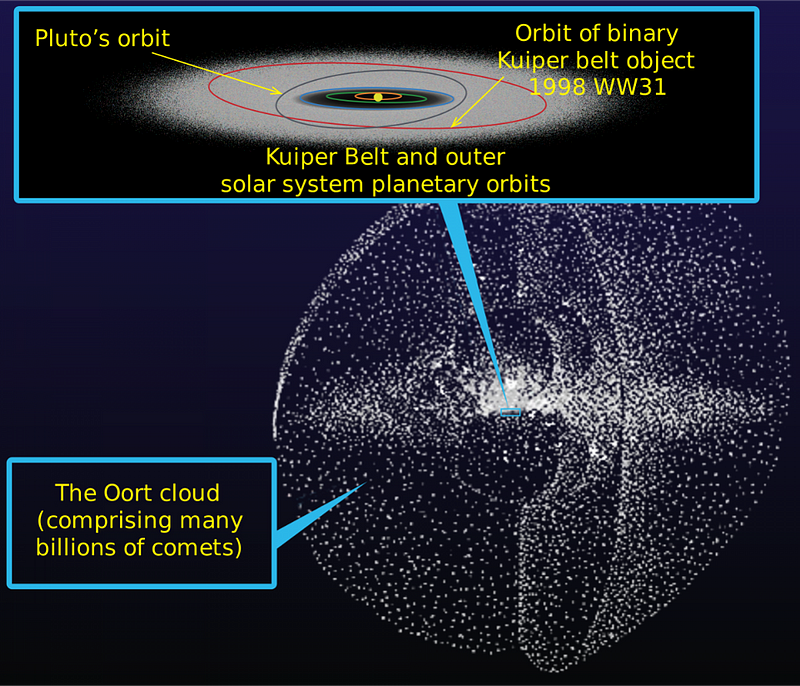
In other words, the effects of a passing star won’t have an observable effect on what icy, comet-like bodies come into the inner Solar System until another 20 or so additional stars have had a close encounter with our own! This is problematic, because the last star system that passed near our own Sun, Scholz’s star (which did so 70,000 years ago), is already 20 light years away from us. However, there is a potentially optimistic thing that comes from this analysis: as we come to better map out and understand the stars and their motions within the nearest 500 light years, we can better predict when-and-where rogue, incoming Oort cloud objects are likely to arise. If we’re concerned with planetary defense from objects hurled inwards by passing stars, this kind of knowledge is the obvious next step.
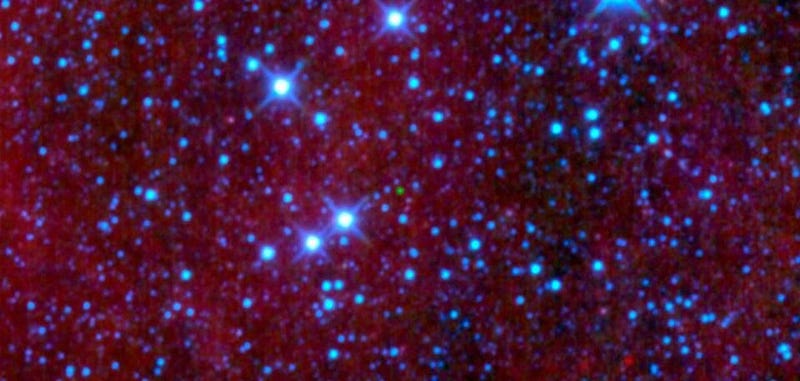
It will require building wide-field surveying telescopes capable of seeing faint stars out to great distances. NASA’s Wide-field Infrared Survey Explorer (WISE) mission was the prototype for this, but the distances over which it could observe the faintest, most common stars was severely limited by its size and observing time. An all-sky, infrared space telescope could map the neighborhood around us out, telling us what’s likely to arrive, on what timescales, from what directions, and what star caused these perturbations in the Oort cloud objects. Gravitational interactions are always occurring, as even though there’s a great distance between stars in space, the Oort cloud is huge, and we literally have all the time in the world for objects to pass by and affect us. Given enough chances, everything you can imagine will occur.
Send in your Ask Ethan questions to startswithabang at gmail dot com!
Ethan Siegel is the author of Beyond the Galaxy and Treknology. You can pre-order his third book, currently in development: the Encyclopaedia Cosmologica.