Ask Ethan: What Causes Light To Redshift?
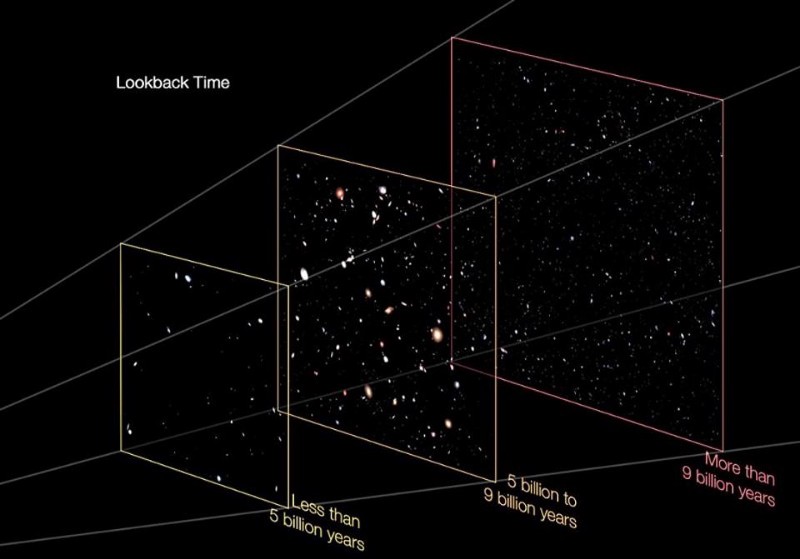
The light we observe isn’t the same as the light that gets emitted. Here’s what causes it.
The light you see, when you look out at the stars and galaxies that fill the Universe, isn’t the same as the light that gets emitted by those very stars and galaxies. Before it can arrive at our eyes, that emitted light has to travel large distances — from a few light-years for the nearest stars to billions of light-years for the most distant galaxies — and contend with every obstacle the Universe puts in its way. So how do we know what the light we see actually tells us? That’s what Peter Ehret wants to know, writing in to ask:
If light is moving across space that’s expanding, is the speed credited with the underlying space expansion? […] A pitcher throwing a ball from a standstill throws at 100mph, but that same pitch from a platform moving at 25mph flies at 125mph. Is it like that for light? What does a red or blue shift mean in terms of the speed of light?
There’s a lot to unpack, but the Universe must contend with it all.
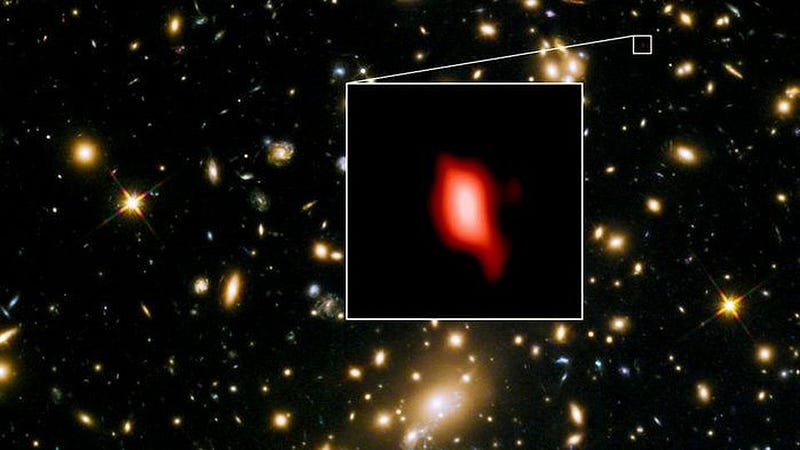
Imagine you have a distant object that lies far outside of the Milky Way. In your mind, you could simply draw a straight line that connects that distant galaxy to us, and picture light traveling along that line straight to our eyes. It’s tempting to do the most straightforward thing you can imagine:
- calculate the distance of that line (in light-years),
- picture a photon leaving its home galaxy,
- traveling along that line for the right amount of time (in years) to traverse that distance through space,
- and then seeing the photon arrive here, where we are.
Only, when we measure the light coming from distant objects, that’s not the story the Universe tells. Instead, that light gets affected by everything along its path, and the light we wind up observing is very different from the light that’s emitted from that distant, extragalactic source.
Light, by virtue of having no rest mass but still carrying both energy and momentum, can never slow down as it travels through the Universe; it can only travel at the speed of light. Whereas an object with mass will always move slower than the speed of light — since accelerating it to the speed of light would require an infinite amount of energy — light itself must always travel at the same speed: c, or the speed of light in a vacuum.
Only when it’s not in a vacuum, i.e., when it passes through a matter-containing medium, does light slow down. This slowing affects different frequencies (or colors) of light by different amounts, just as how white light passing through a prism will split into different colors at different angles, because the amount that light slows down is dependent on the individual energy of the photons. Once it goes back into a vacuum, however, it resumes moving at the speed of light. The only difference is that the light, having passed through a medium, is now blurred.
Back in the early days of relativity, there were a large number of challenges to Einstein’s theories and the predictions they made. Did light always move at a constant speed through the Universe? Was there really no need for a medium for light to travel through? Did the fabric of space really curve and distort due to the presence of matter and energy? And was the Universe actually expanding?
One alternative was the tired light scenario, which predicted that light would lose energy as it traveled through the medium of space. Light that arrives appears to have less energy than the light that must’ve been emitted, but the lack of increased blurring at greater distances ruled that out. Light did move at a constant, wavelength-independent speed through the vacuum of space, with no need for a medium based on experiment and observation. Most excitingly, the fabric of space really did demonstrate curvature near masses, in line with Einstein’s predictions.
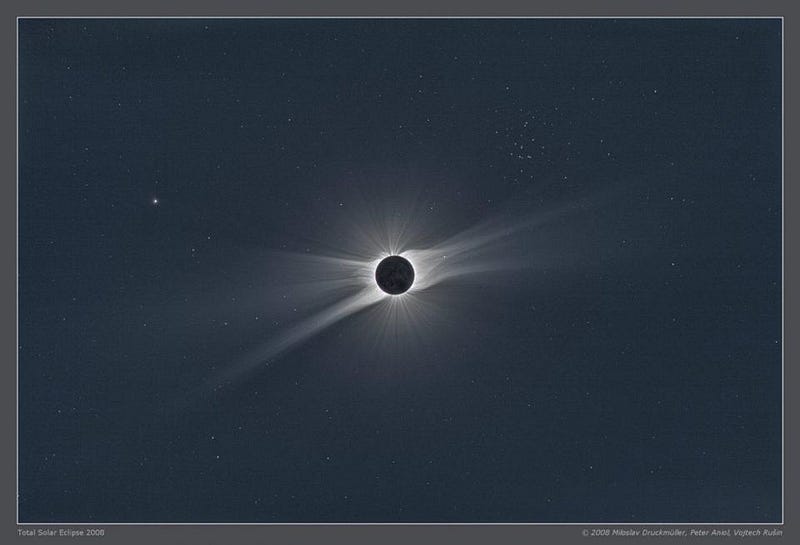
If Einstein’s General theory of Relativity — which combined Special Relativity and the constancy of the speed of light with gravitation — were correct, then the speed of light can never change as it moves through the Universe. All the different things light can experience, from traveling through curved and expanding space to passing through intervening matter (both normal and dark) to the relative motion of the emitting source and the observer, all affect it, but not by changing its speed.
The way light compensates for all the different things that can affect its energy is by gaining or losing energy, which translates into either:
- a blueshift, which corresponds to an energy gain, a shortening of its wavelength, and an increase in its frequency,
- or a redshift, which corresponds to an energy loss, a lengthening of its wavelength, and a decrease in its frequency.
When we take everything into account, we find that there are five major ways that light gets affected on its journey through the Universe.
1.) The fabric of space expands. This is the cause primarily responsible for the redshifting we see of distant galaxies. Light travels through the fabric of space, which is expanding as time progresses since the Big Bang, and that expanding space stretches the wavelength of the light that travels through it.
Since light’s energy is defined by its wavelength, the light gets redshifted more severely the farther away the emitting galaxy is, because more distant galaxies require more time for their light to eventually reach Earth. Our naive picture of light traveling along a straight line, unchanging path only works in a non-expanding Universe, which doesn’t describe either what we see or what General Relativity predicts. The Universe is expanding, and that’s the primary contributor to the redshifts we see.
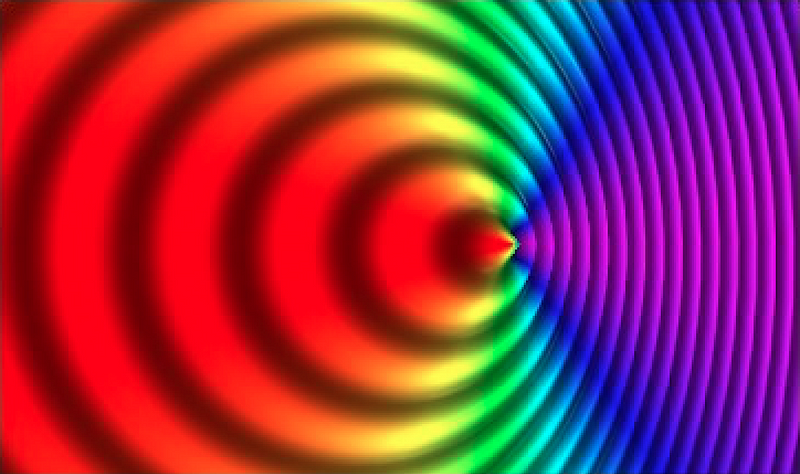
2.) The motion of objects relative to us. Just like a police siren sounds higher-pitched when it moves towards you and lower-pitched when it moves away from you, the frequency of the light we observe gets shifted towards either higher frequencies (blueshift) or lower frequencies (redshift) depending on the relative speed of the source and the observer.
In astronomy, we call this “peculiar velocity,” since it’s primarily due to the velocity of the galaxy in question relative to us, and is usually a few hundred or thousand kilometers per second. Two galaxies at the same distance can have their redshift or blueshift differ significantly, particularly inside rich galaxy clusters, where peculiar motions are fastest. The fact that we can account for and quantify this definitively tells us that this is not the dominant contributor to cosmological redshifts.
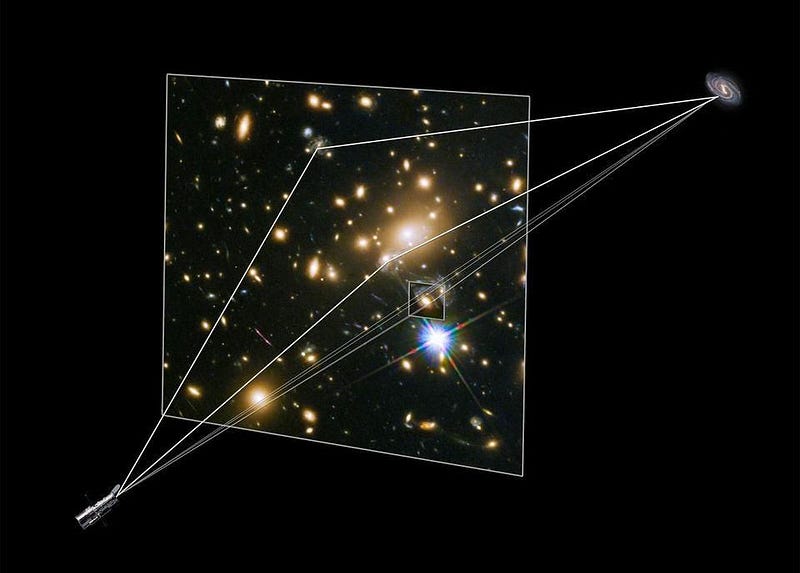
3.) Gravitational lensing. The fabric of space isn’t just expanding, it’s also curved by the presence of matter and energy within the Universe. This curvature means that the distance between any two points isn’t a straight and unbroken line, but rather is a curved path through space: a geodesic. Depending on how much space is curved by, this can delay the arrival of the light by significant amounts over and above the time it would take without these masses and the extra curvature, meaning that the light has to travel for longer than it would, otherwise, through the expanding Universe.
That additional time delay means that the light experiences an additional redshift, and even that a gravitationally lensed source that exhibits multiple images following separate paths through more (or less) severely curved space will have different redshifts for different images. General Relativity demands that this effect exists, even if our astronomical equipment isn’t yet advanced enough to detect it.
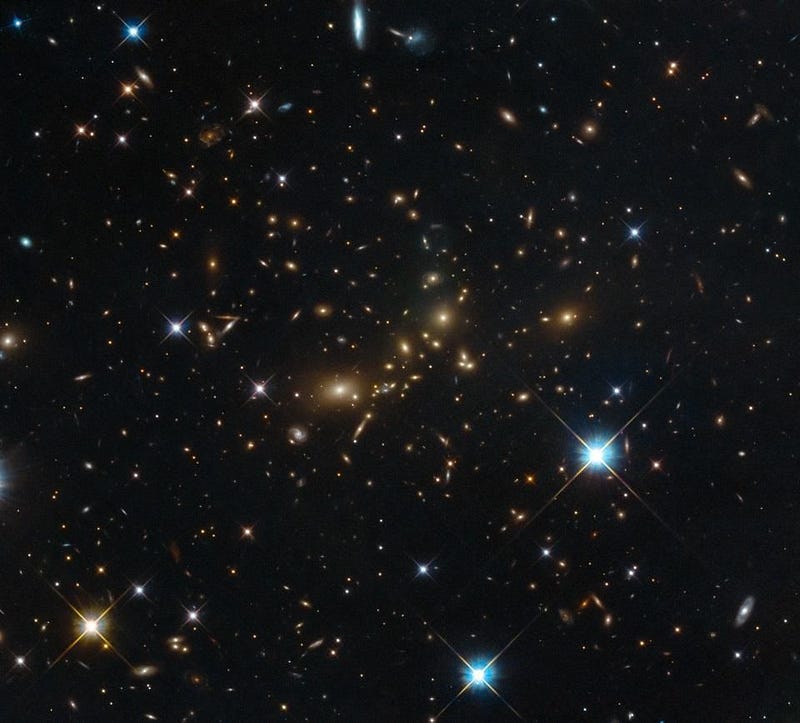
4.) Interactions with matter. The Universe is mostly empty space, but matter still exists. In particular, much of that matter is in the form of either gas (which comes in a variety of temperatures) or ionized plasma. When light passes through matter where it can interact with charged particles (electrons in particular), some of that light will get boosted to higher energies where it won’t be observed anymore, shifting the spectrum of that light.
Although this is most observable for the light leftover from the Big Bang, it occurs in principle for all forms of light, and changes the temperature and spectrum of the light we observe before it arrives in our detectors. This affects the light due to the temperature, motion, and polarization of the gas/plasma that interacts with the light that passes through it. It plays only a very minor role in practice, but it is a real effect.
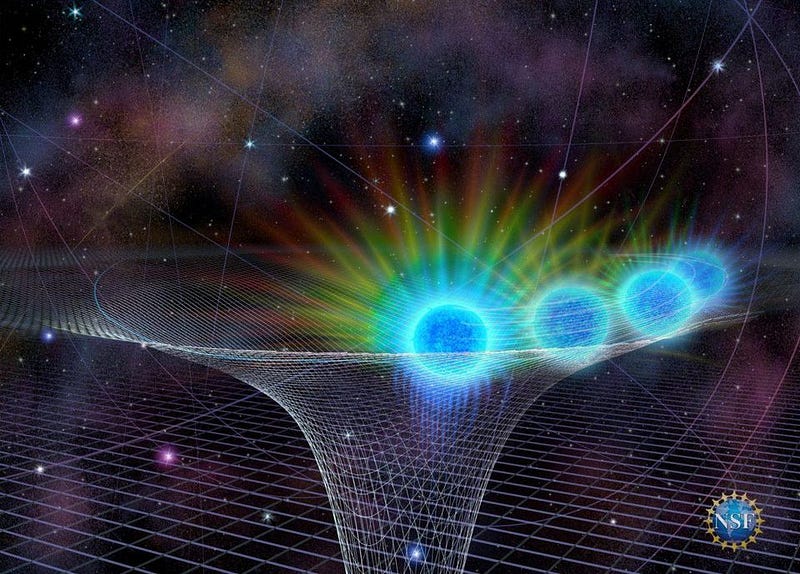
5.) Gravitational redshift. When you’re a massive object that emits light, that light has to climb out of the gravitational potential created by your mass. Since light can’t slow down (it always moves at the speed of light), that means it has to lose energy to reach interstellar or intergalactic space. Similarly, before that light arrives at your eyes, it has to fall into the gravitational potential of our own Local Group, galaxy, and Solar System, causing an energy gain and a blueshift.
All of this affects the light’s frequency. In addition to this, structure actively forms in the Universe over time, so that the gravitational potential that a photon falls into (say, if it passes through a galaxy cluster) may be different from the gravitational potential millions of years later, when the photon climbs out of it. These effects — of both the gravitational potential and the changes in gravitational potential — have been detected, and contribute to the final, observed redshift of light.
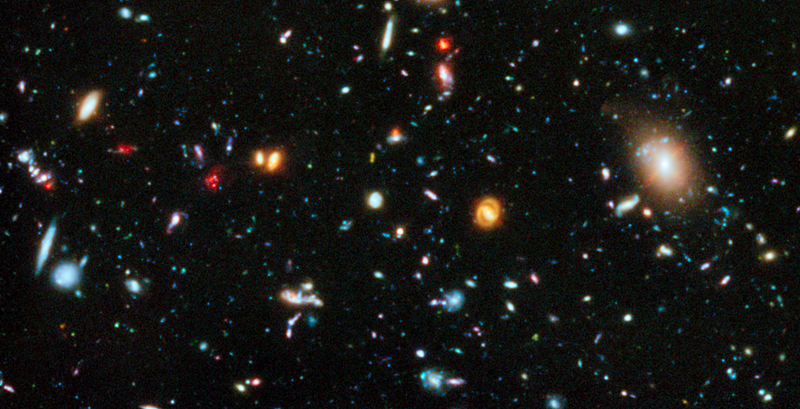
The speed of light never changes in the vacuum of space; only when passing through a medium (and only while light is passing through that medium) is the speed of light ever different from c, the ultimate cosmic speed limit. However, there are five real effects that can cause a redshift or blueshift as light travels through the Universe, and the most important lesson is that we can quantitatively account for all of them.
That’s the effect of the matter in the Universe, the expanding and evolving fabric of space, and how the different masses and forms of energy move through and affect that space. All of it impacts the light that travels through space, but not by altering its speed. Rather, they alter the path that light travels and the wavelength the light possesses, and that makes all the difference. Only by accounting for all the effects together can we truly understand what happens to light as it travels through the expanding Universe.
Send in your Ask Ethan questions to startswithabang at gmail dot com!
Ethan Siegel is the author of Beyond the Galaxy and Treknology. You can pre-order his third book, currently in development: the Encyclopaedia Cosmologica.