Ask Ethan: What Caused This Remarkable ‘Sun Pillar’ Shortly After Sunrise?

They say that seein’ is believin’. But everything we see has to have a scientific explanation.
When you look at the Sun on a day where there’s a clear sky, you normally expect to see a blinding orb of light. But every once in a while, the atmosphere has a bit of a surprise for us, and gives us a sight that’s rare and unfamiliar. Rachel Perry, an amateur photographer from Virginia Beach, wrote in (along with the above picture) after observing this phenomenon she’d never captured before.
On Tuesday, April 21, 2020, the most remarkable astronomical phenomenon was observed from the shores of Virginia Beach VA. Nearly 60-minutes following sunrise, at approximately 7:33 a.m. EST., multiple flares of cone-shaped light, were observed emitting from the top and bottom of the Sun forming 22-degree arcs on either side. […] Any feedback on what I witnessed would be greatly appreciated!
The short answer is that this is known as a Sun Pillar, but the science behind it is completely fascinating. Let’s dive in.
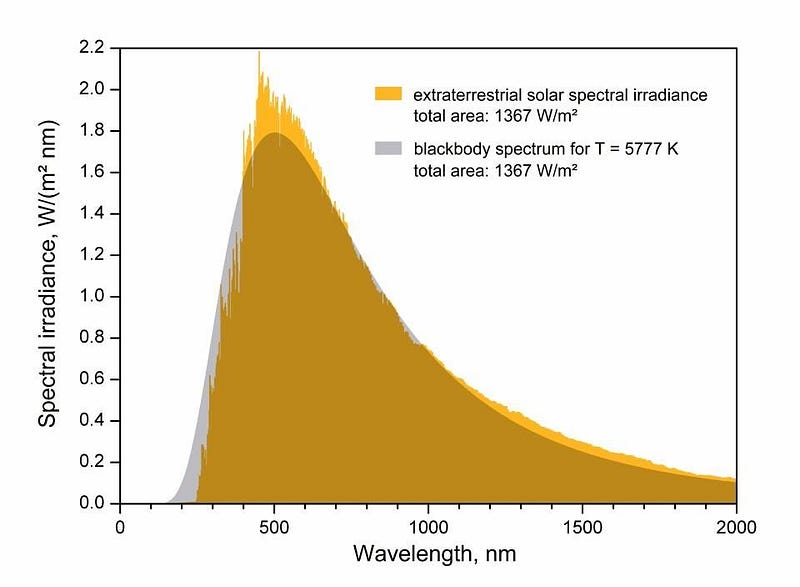
If all we had to work with were the emitted light from the Sun, optical phenomena like this would never, ever occur. The Sun emits a fascinating set of light that we can coarsely model as a blackbody: a perfect absorber that’s heated up to a specific temperature, where it radiates energy away. It’s an excellent approximation, but science has done even better than this approximation.
The Sun isn’t actually a solid, perfectly absorptive body, but rather emits light from many different surfaces throughout its tenuous outer layers. This matters, because the lower-down layers are at a higher temperature than the uppermost layers, so it’s more accurate to model the Sun as the sum of a series of blackbodies, as you see above. Moreover, the Sun contains a wide variety of atoms, and these atoms absorb light at specific frequencies (below), meaning there are “gaps” in the light that actually leaves the Sun.
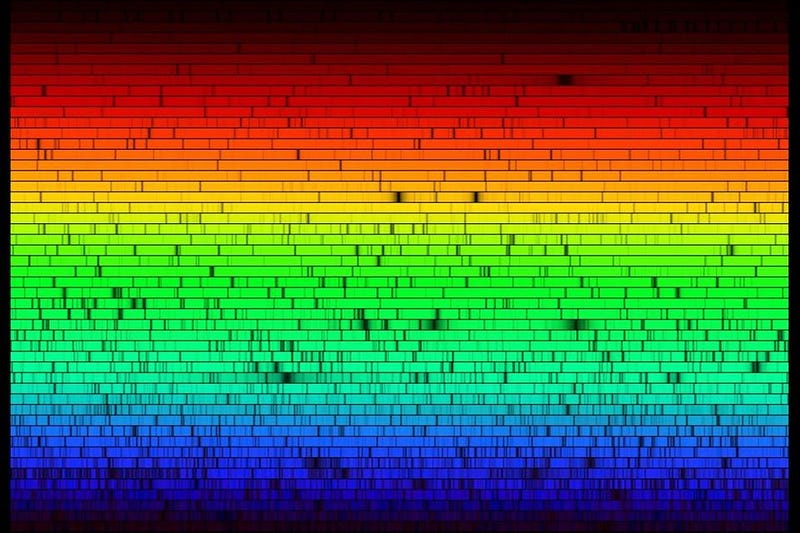
As it travels through the void of empty space, this light simply spreads out in a spherical shape as it radiates omnidirectionally away from the Sun. If we lived on a world without an atmosphere at all, this is exactly the light we’d observe: the same light that the Sun itself radiated away.
But we live on planet Earth, which — to an astronomer, at least — is like viewing the entire Universe from the bottom of a swimming pool. Our atmosphere absorbs, scatters, or reflects a large portion of the sunlight that strikes it, even on a perfectly clear day. The absorbed light gets re-radiated as infrared light; the scattered light affects different wavelengths to different degrees and turns the sky blue; the reflected light returns back to space. The majority of the sunlight that strikes our atmosphere will makes it through, however, and that’s what we observe when it’s perfectly clear.
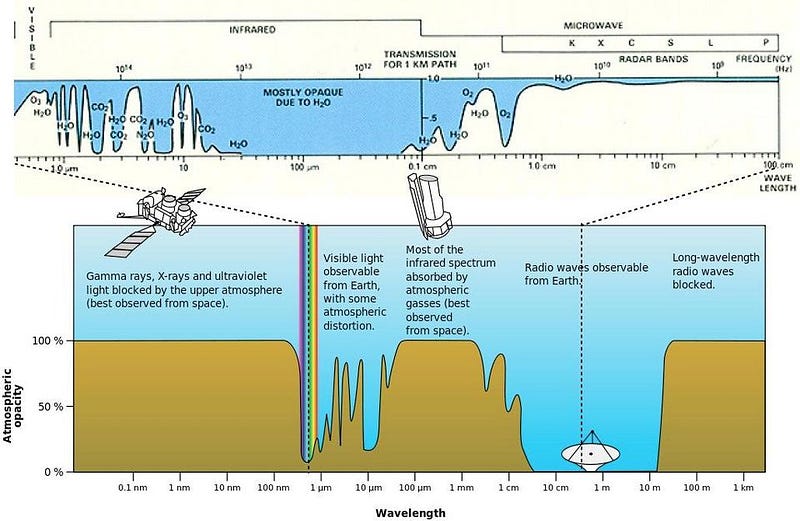
Now, we have to add one more layer of complexity to understand what’s going on: the properties of our atmosphere. If you thought our atmosphere was about 4 parts nitrogen to 1 part oxygen, congratulations, as that’s exactly what the majority of Earth’s atmosphere is composed of. There’s about 1% argon sprinkled in there, along with trace amounts of carbon dioxide, methane, and other gases as well.
But the atmosphere also contains water vapor: in large (about 1–2%) quantities that vary with time and specific conditions. Moreover, the atmosphere also has severe temperature gradients to it, which does something very interesting when you throw water vapor into the mix. At some point, the temperature will be such that the water will no longer remain in the gaseous phase, and will either condense into liquid droplets (forming the clouds you’re familiar with) or go all the way into the solid phase, forming ice.
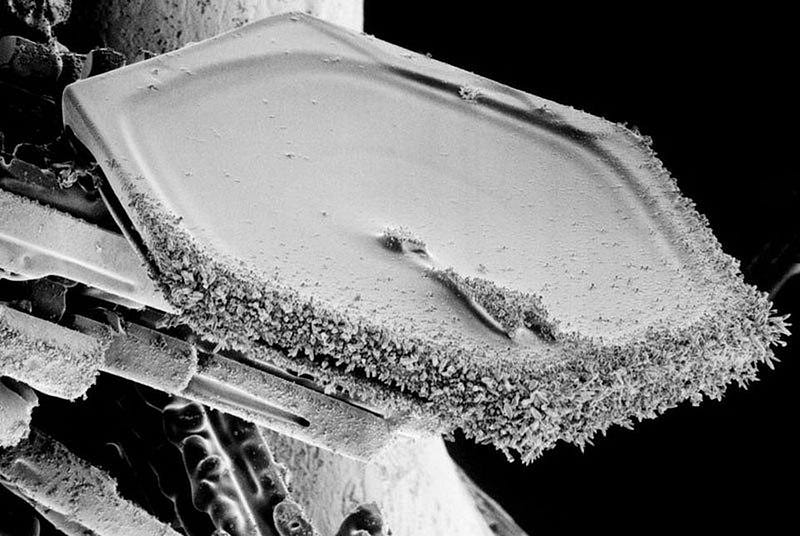
While you might think about atmospheric ice in the form of hailstones or sleet, what’s actually far more common, particularly at very high altitudes, is that very small crystals are formed high up in the atmosphere. These crystals don’t look like the complex snowflakes you’re accustomed to, but rather preferentially form into a hexagonal shape: one of the most common shapes for ice crystals made of small numbers of water molecules.
All hexagonally shaped ice crystals have the same angles at their vertices, which leads to the same angles-of-reflection for any sunlight that strikes it. Those same optical properties that are at play in the atmosphere in general — refraction, reflection, transmission, scattering, etc. — still occur among these ice crystals, but the results are far more striking and spectacular. The hexagonal symmetry can make long pillars (known as columns) or thin plates, but they all have the same angles between each of their faces.
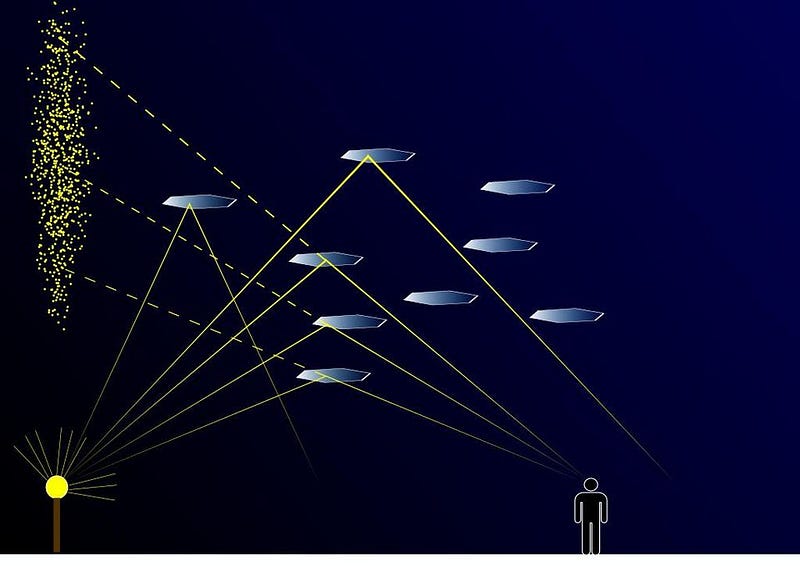
When those crystals are created, they’re always heavier-than-air, which is true for all forms of ice. As these ice crystals start to fall, they’re slowed by air resistance, and the specific balance between air resistance and the crystals themselves will determine their orientation relative to our line-of-sight. Plate crystals normally drift downward like leaves, with the large face parallel to the ground, while column crystals are normally oriented horizontally.
When the sunlight strikes those crystals, however, it always causes the light to reflect off at a set of predictable angles, while we’re only capable of observing the light that’s at just the right angle to strike our eyes. This typically manifests in only three ways:
- a large halo of light that’s at a specific separation angle (22°) from the Sun (from randomly oriented crystals),
- a pillar of light that’s due to vertical reflections from either plate crystals (when the Sun is very close to the horizon) or column crystals (when the Sun is somewhat higher),
- or a combination of the two effects, where horizontal crystals reflect light from the vertical parts of a halo, creating the “flared” halo effect known as a sun dog.
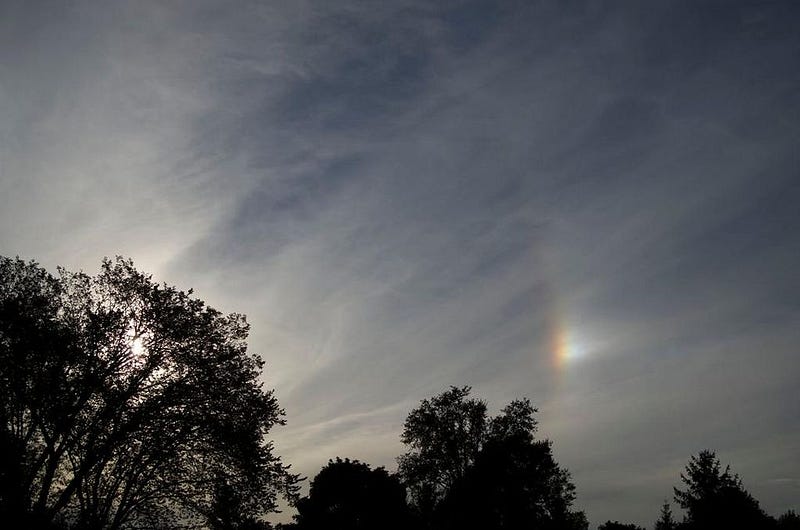
The fact that the picture in question was taken about an hour after sunrise indicates that the dominant pillar effect is due to largely to column crystals that are falling through the atmosphere, both above and below the Sun’s apparent location. Given the date, time, and location of the event, the Sun is approximately 9° above the horizon at the moment this photograph was snapped.
Investigations into the optical properties of various light pillars (which include not only sun pillars, but similar pillars due to the Moon or any other, even artificial, light sources) have taught us that the thin plate crystals are responsible for pillars where the Sun is below or very near (within 6° of) the horizon, while the horizontally-oriented column crystals are primarily responsible for the pillars when the Sun is at higher positions (up to 20° above the horizon). The dominant cause of this observed pillar, therefore, is likely due to column crystals.
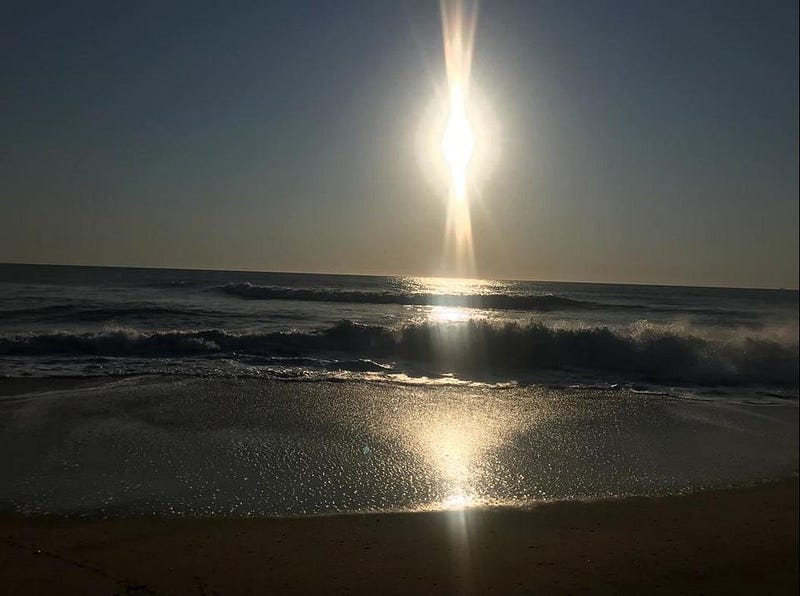
What’s fascinating about this particular sun pillar that was captured here is that it comes along with an even rarer optical phenomenon: an elliptical halo. Elliptical haloes are only rarely visible, and are one of the least well-understood optical phenomena we observe in our atmosphere. There can be up to three elliptical rings seen at once around the Sun, but normally they’re entirely lost in the Sun’s bright glare.
While we don’t know for certain what causes these elliptical haloes, one fascinating approach is to simulate what could create this optical phenomenon with ray-tracing. Instead of hexagonal plate or column crystals, it’s plausible that some of these crystals could be flawed plates: where the top and bottom faces aren’t completely flat, but are instead very shallow pyramids, with angles that depart from perfect flatness by only 1°-to-3°.
But we also have to be careful about ascribing 100% of the optical phenomena we see here to the atmosphere alone. Oftentimes, particularly when it comes to photographing bright objects like the Sun, there are internal reflections and optical effects that occur inside the components of the camera itself. Many of the rays you see in photos like this may not appear to your eyes; this becomes clear when you notice the rays coming off of the water’s reflection, which one only sees in photographs, not with direct observations.
Nevertheless, both the pillar and the elliptical halo are definitely real, and the photographer was very fortunate to be able to catch them. Fewer than 1-in-1,000 sunsets and sunrises contain these remarkable phenomena; to everyone who gets to see or experience one for themselves, appreciate that you’re being treated to something that most humans will never encounter even once.
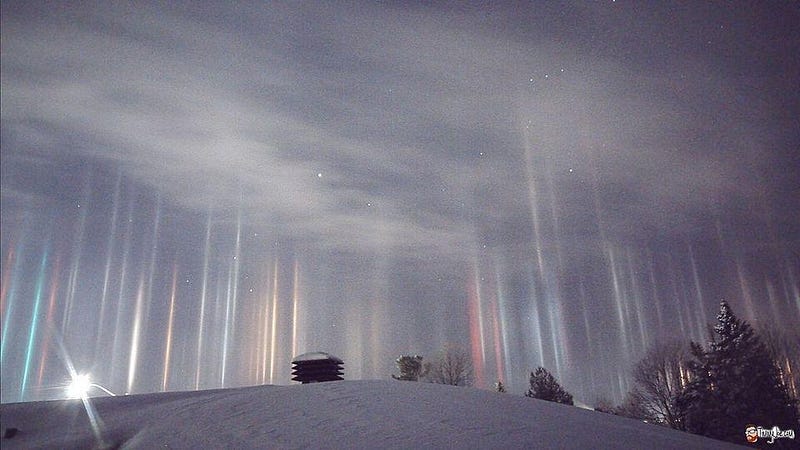
Almost all of the atmospheric phenomena that result in light appearing in a location other where the main source is are due to either ice crystals or water droplets in the atmosphere. While rainbows and glories arise from water droplets, almost everything else we observe is due to ice crystals. Haloes, pillars, sundogs, subsuns (the bright light you see from airplanes in the opposite direction from the Sun) and more all arise from the same source: tiny ice crystals.
Here, we’re treated not only to the sun pillar phenomenon, but also an accompanying set of very rare elliptical haloes, made possible only by the right conditions that are still in the process of being uncovered. Whatever the cause, it’s one more reminder to take in the spectacular sights that the natural world has to offer. You never know how it will wind up surprising and amazing you unless you look.
Send in your Ask Ethan questions to startswithabang at gmail dot com!
Ethan Siegel is the author of Beyond the Galaxy and Treknology. You can pre-order his third book, currently in development: the Encyclopaedia Cosmologica.