Ask Ethan: Is the Universe expanding faster than expected?

And if it is, what does that mean for dark energy, and our Universe’s fate?
“Until the 1990s, there were few reliable observations about movement at the scale of the entire universe, which is the only scale dark energy effects. So dark energy could not be seen until we could measure things very, very far away.” -Adam Riess
Governed by the laws of General Relativity and begun with the hot Big Bang some 13.8 billion years ago, most of us don’t recognize that the ultimate fate of the Universe was determined from the very moment of its birth. The initial conditions are basically a race: between the initial expansion on one hand, working to stretch all the matter and energy apart from each other, and from gravitation on the other, working to pull things back together, slow the expansion rate down, and, if possible, recollapse the Universe. If we know how the Universe is expanding both now and in the past, we can figure out both what makes it up and what its ultimate fate will be, but only if we can measure our past accurately.
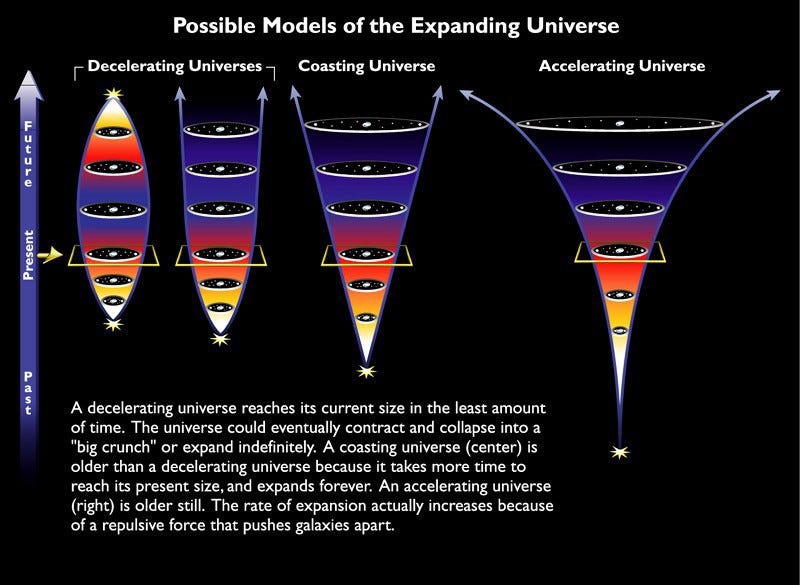
This week, I’ve gotten an overwhelming number of questions (from Zahra, Dianne, Alex, Rob, Ciro, Lautaro and others) about a recent report that the Universe is expanding faster than we’d thought. The worry is this: if the fate of the Universe depends on what the expansion rate is and has been in the past, and we’ve measured it wrong, could our conclusions about the Universe be wrong, too? Could there, perhaps, not be any dark energy? Could it be that the Universe isn’t accelerating away from us after all? Might the expansion rate continue to slow down in the far future, perhaps even recollapsing in a Big Crunch? In order to answer that, we’ve got to look at the science behind what’s actually going on.
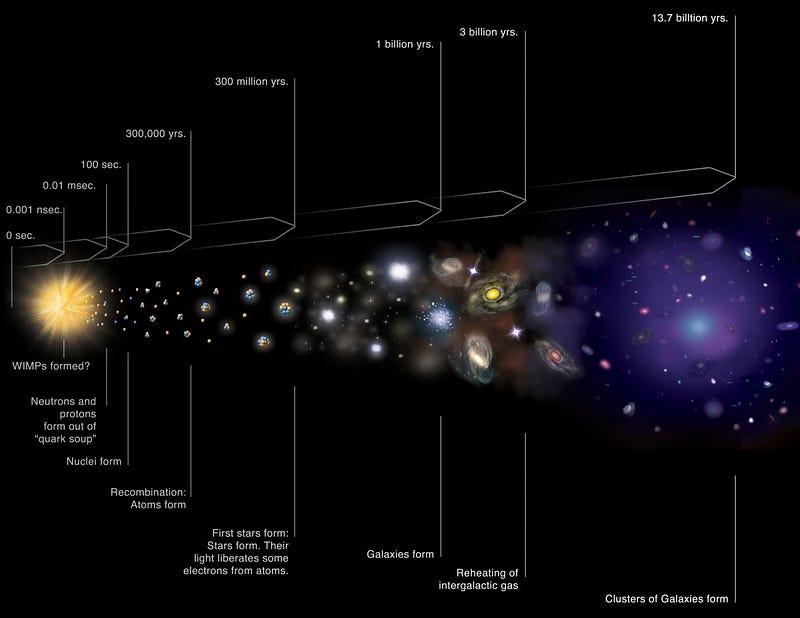
The simplest way to measure how the Universe is expanding is to look at objects we understand — things like individual stars, rotating galaxies, supernovae, etc. — and measure both their apparent brightness and their redshift. If we know how intrinsically bright something is, which we do for well-understood objects, and measure how bright it appears, we can infer how far away it has to be, the same way we can know the distance to a 60 Watt light bulb by measuring its brightness. (Astronomers call these objects “standard candles,” since the idea predates light bulbs by multiple generations.) Since the Universe is expanding, measuring the redshift and distance both allows us to observe how space is expanding today, and by going to greater and greater distances, we can observe how that expansion rate has changed over time.
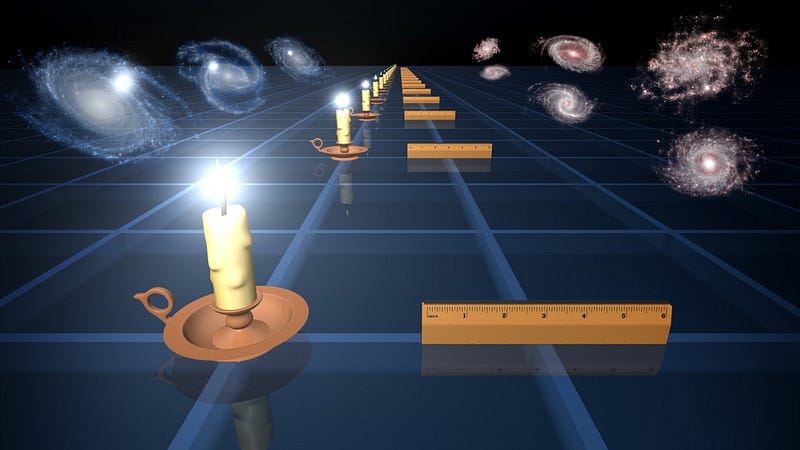
This concept is the same for many different classes of objects: Cepheid variable stars, fluctuations on the surfaces of spiral galaxies, evolving red giant stars, rotating spiral galaxies, and type Ia supernova, the last of which can reach the greatest distances of all. A combination of all of these methods was used in the 1990s and early 2000s to determine what the Hubble expansion rate of the Universe was to a tremendous accuracy: 72 ± 7 km/s/Mpc, a tremendous advance from previous studies which vacillated between values of 50 and 100. (The Hubble space telescope, which made these measurements, was so named because of its intent to measure the Hubble rate!)
But since that time, we’ve refined our measurements and reduced our errors even further, uncovering a new problem: different types of measurements give different values for the expansion rate.
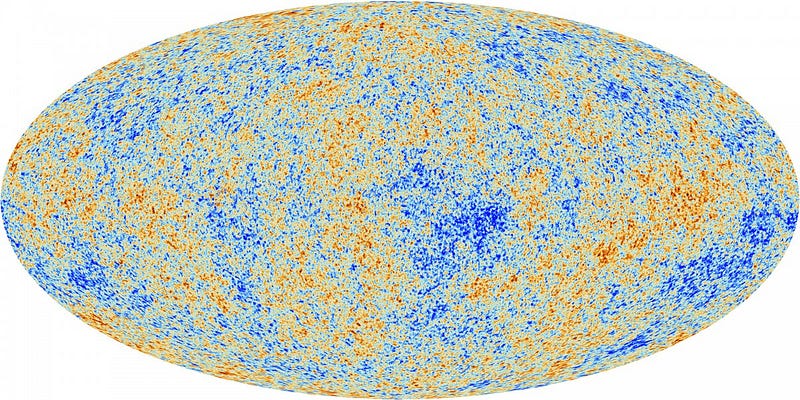
One way to measure the expansion history of the Universe is to look at the Cosmic Microwave Background: the Big Bang’s leftover glow. The fluctuations in it as well as some overall global properties enable us to reconstruct what the expansion rate is from this measurement alone. From the Planck satellite, we obtain a value of 67 ± 2 km/s/Mpc, which is consistent with prior measurements but more precise. From the clustering of galaxies on the largest scales (baryon acoustic oscillations), as measured by the Sloan Digital Sky Survey and others, we get a value of 68 ± 1 km/s/Mpc. These two large-scale measurements seem to give a value that’s consistent with prior data and also with one another. But if we take a look at Cepheid data and supernova data, where we have Cepheid variable stars and type Ia supernovae in the same galaxy, we get an equally precise — but inconsistent — value: 73 ± 2 km/s/Mpc.
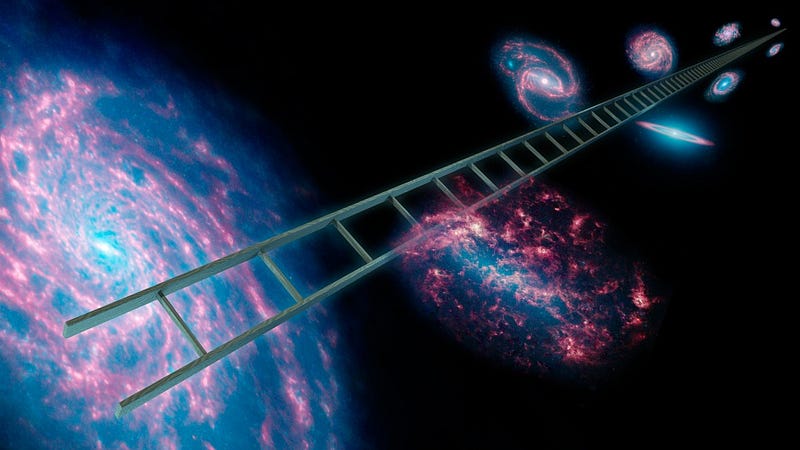
This is what the recent hullaballoo is all about. Some people propose wild theoretical alternatives, like evolving dark energy, to explain it, while others question the core assumptions of cosmology. But it’s quite possible — perhaps even probable — that there’s no problem at all. Because not included in these errors are systematic errors, or uncertainties inherent to the measuring process. The Cepheid and supernova data allow us to construct a cosmic distance ladder, where each more distant “rung” of the expanding Universe is built upon a closer, pre-existing rung. If we’ve made an error early on:
- in the parallax measurement of the nearest Cepheids,
- in the “standard-ness” of each of these objects,
- in the brightness-distance relationship of any rung along the way,
- in the intrinsic, assumed brightness of these standard candles,
- or about the environments in which these phenomena are found,
that error will propagate to all our future conclusions. Despite the small uncertainty from this distance ladder, it’s worth pointing out that they have four different, independent ways of calibrating the Hubble rate, and each of these ways gives a different value, ranging between 71.82 and 75.91, each with an uncertainty of approximately 3.
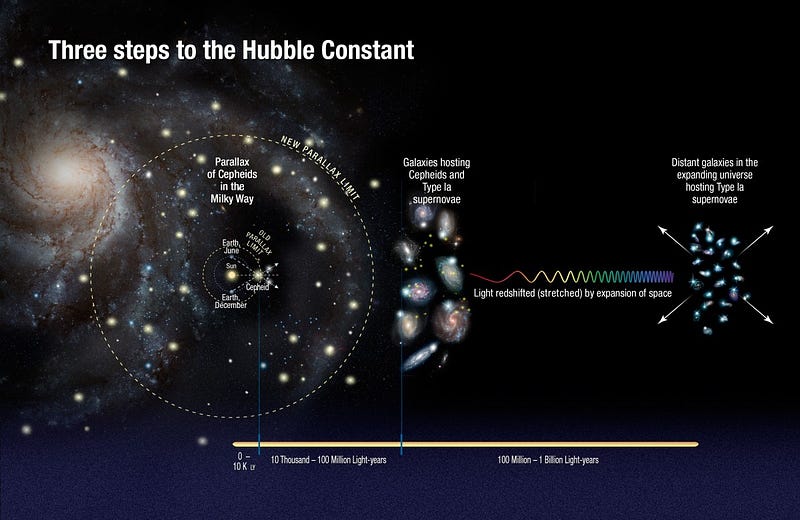
The hope is that upcoming parallax measurements greatly improve these uncertainties, and also help us understand the systematic errors that are likely at the heart of these differences. While it might be fun to speculate wildly, these new pieces of evidence for tension in the Hubble expansion rate most likely point towards an opportunity for us to better understand the astrophysical phenomena that lead to these values, and to hopefully, eventually converge on a single value for the expansion rate that’s consistent across all methods. Even if the value jumps to 73, remains at around 70 or falls all the way down to 67, that has the potential to change our parameters by a few percent, but not our conclusions. Perhaps the Universe is 13.5 billion years old instead of 13.8; perhaps it’s made of 65% dark energy instead of 70%; perhaps there’s still room for a Big Rip some 40 billion years from now. But the big picture of the Universe remains the same, even if this tension is real. The key, as always, is discovering the underlying cause, and learning whatever it is the Universe has to teach us in the end.
Send in your questions for Ask Ethan to startswithabang at gmail dot com!
This post first appeared at Forbes, and is brought to you ad-free by our Patreon supporters. Comment on our forum, & buy our first book: Beyond The Galaxy!