Ask Ethan: How Prepared Are We For The Next Giant Solar Flare?
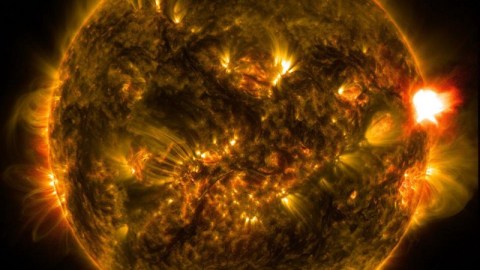
Not nearly well enough. And we should all be concerned.
In 1859, the science of solar physics truly began with the largest eruption in recorded history: the Carrington event. Prior to this time, many people had observed the Sun: counting and monitoring sunspots, watching the Sun’s differential rotation rate, and making a potential link between sunspot activity, the Earth’s magnetic field, and observations of Earth’s aurora. But when astronomers Richard Carrington and Richard Hodgson noticed an enormous “white light flare” on the Sun on September 1, 1859, we realized that the Sun and the Earth were connected as never before. Just 17 hours later, Earth experienced the largest geomagnetic storm ever recorded, and the worldwide reports of its effects are now legendary. Knowing that these events happen regularly, are we now prepared for the inevitable? That’s what Erich Rathkamp wants to know, asking:
“a CME the size of the 1859 Carrington Event would, if not prepared for, effectively level the power grid of the United States… Can we actually provide a full day’s worth of warning? Is a sufficient warning period actually significant enough to allow us to survive a Carrington class [event?] …if a Carrington class event were to be detected tomorrow, would we actually be able to survive it effectively?”
When it comes to looming natural disasters, the best thing we can do is to make sure we’re prepared. Here’s what the Sun has in store for us.
Normally, the Sun is a fairly quiet entity, outputting the same continuous amount of power to within 99.9% precision. It rotates about its axis, with a period of 25 days at its equator and 33 days at its poles, and also emits a steady stream of particles: the solar wind. Its central core reaches a maximum temperature of ~15 million K, but the limb of its photosphere is a relatively cool ~6,000 K, and that’s what radiates the energy we receive.
In addition, there’s a tenuous, very hot plasma separated from the photosphere: the Sun’s corona, which is hundreds of thousands of kelvin, and the Sun’s chaotic, irregular magnetic field frequently connects the two. Occasionally, however, the Sun develops sunspots, which are relatively cool regions on its photosphere. There are magnetic connections between the Sun, the corona, and even the other bodies in the Solar System, like Earth. Originating from a variety of processes, solar flares, coronal mass ejections, and other magnetic reconnection events can occur, sending out a stream of energetic particles in a particular direction.
Under normal circumstances, these particle streams are:
- relatively slow-moving and low in energy, taking about 3 days to reach Earth’s distance from the Sun,
- tend to miss the Earth, since they are fairly localized in space and the odds of striking Earth’s precise location are low,
- and even if they do hit the Earth, our planet’s magnetic field tends to funnel them harmlessly away, perhaps except for down around the poles, where they can create the beautiful and spectacular aurorae.
Importantly, the particles themselves pose no danger to biological organisms on Earth’s surface, like us. But that doesn’t mean we’re immune to any ill effects that might ensue.
If everything lines up in exactly the wrong way, the outcome can be disastrous. If a solar flare causes a coronal mass ejection, and if that coronal mass ejection is high in energy, and if the particles from it head directly for Earth, and — one more thing — if the magnetic field of the ejected material and the magnetic field of Earth are anti-aligned, that’s a recipe for maximum damage to our planet: infrastructure, electronics, and a whole lot more. That’s almost certainly what happened 162 years ago, when the now-infamous Carrington event occurred.
At about noon on September 1, 1859, Richard Carrington was tracking a large, irregular sunspot on the face of the Sun, when all of a sudden a brilliant flare occurred over it. Carrington described the flare as intensely bright, and as migrating from the left to the right of the sunspot over the span of about 5 minutes. Then, just as suddenly as the flare appeared, it disappeared entirely.
Some 18 hours later — about 3 to 4 times the speed of a typical solar flare — the largest geomagnetic storm in recorded history occurred. Aurorae were seen around the world; miners in the United States were awoken by the bright lights, thinking it was dawn. In places where it was night, the aurorae were bright enough that newspapers could be read by its light. The “green curtain” of aurorae could be seen at many equatorial latitudes: Cuba, Hawaii, Mexico, and Colombia all reported them. And, most disconcertingly, our early electric systems, like the telegraph, experienced their own induced currents, causing shocks, starting fires, and tapping wildly, even when the systems themselves were disconnected entirely.
The physics behind this is both simple and, if you think about it, terrifying. The charged particles that are emitted from the Sun and strike the Earth’s atmosphere aren’t themselves harmful, as the atmosphere has outstanding stopping power. But these particles, when they move in large numbers and at high speeds, will create their own magnetic fields, like any electrical current. If these magnetic fields are strong enough, they can substantially change the local magnetic field at Earth’s surface. And if you change the strength and/or direction of a magnetic field that passes through a loop or coil of wire, that changing magnetic field will induce an electric current.
I’ll say that again: if you have a loop or coil of wire where the magnetic field changes inside, it will create an induced electric current. Humanity knew about this law well prior to the Carrington event; Faraday discovered it back in 1831. But the world has changed an awful lot since Carrington’s day, as power grids, power stations and substations, power transport infrastructure, and even residential, commercial, and industrial electronics are all full of loops and coils of wire. The induced currents, if we were to experience a Carrington-like event today, would literally be astronomical.
The estimates for how much damage — if we do nothing to mitigate it — would occur. The power grids of most countries would be completely and effectively leveled. The top way to mitigate the effects of such a flare would be through increased grounding, so that the large currents that would otherwise flow through grid wires would instead flow directly into the Earth. Every time power companies attempt to do this, however, what winds up happening instead is that the conducting substance used for grounding (such as copper) is stolen for its material value.
As a result, we have under-grounded power stations and substations that would experience enormous induced currents, and that will typically lead to fires, followed by significant damage and destruction to our infrastructure. Not only are we talking about a multi-trillion dollar disaster (the damage to the United States alone has been estimated as high as $2.6 trillion), we’re talking about large swaths of the world’s population being left without power for extended periods of time: potentially for years. When you consider what happened in Texas just very recently when they got hit with freezing temperatures and many areas lost power, there’s the risk of an extremely large number of casualties; for many people, electricity is necessary to sustain their lives.
The Carrington event was not some massive outlier that only occurs once every few million years, either. Many solar flares have struck Earth, some of which have caused localized damage to the power grid. A 1972 set of solar storms caused a widespread disruption of electrical and telecommunications grids, satellite disruptions, and even caused the accidental detonation of naval mines in Vietnam. A 1989 geomagnetic storm caused a complete outage of Quebec’s electricity transmission system. And a 2005 solar storm knocked the GPS network offline. These events may have been damaging, but they were only warning shots compared to what nature inevitably has in store for us.
In 2012, the Sun finally — for the first time since we’ve developed the tools capable of monitoring it sufficiently — emitted a solar flare that was likely as energetic as the one that caused 1859’s Carrington event. It occurred on July 23, and that’s what saved us. The flare occurred in the same plane as Earth’s orbit, but missed us by the equivalent of nine days. Similar to the Carrington event, the particles reached Earth’s distance from the Sun in just 17 hours. Had Earth been in the way, the global damage done could have crested the $10 trillion mark, not to mention the immeasurable loss of life that would have ensued.
Yet most of us don’t think of solar storms the same way we think of hurricanes, tornadoes, earthquakes, tsunamis, or volcanic eruptions. In today’s modern, electronics-reliant world, however, we absolutely should be thinking of this in terms of disaster preparedness. With the new advent — as of only last year — of the Daniel K. Inouye Solar Telescope, we’re finally prepared to get some significant warning when a geomagnetic storm of disastrous proportions may occur.
This solar telescope behaves as a Sun-measuring magnetometer, capable of measuring the magnetic field on the Sun and in the solar corona, allowing us to know whether an Earth-directed coronal mass ejection has exactly the wrong magnetic field for our planet at the moment. If one is detected, we have a chance to take large-scale mitigations, which include:
- having power companies cut off the currents in their electrical grids, which takes gradual ramping-down on the timeframe of approximately ~24 hours to do responsibly,
- to disconnect and (if possible) ground stations and substations, so that large induced currents don’t flow into homes, businesses, and industrial buildings, creating fires,
- and to issue recommendations for residents at home on how to safely cope: unplugging all of your appliances and electronics, disconnecting certain wires and systems, etc.
The fastest solar flare ever to travel from the Sun to the Earth made the journey in just 14.6 hours, meaning that we’d ideally like our response time to be faster than that. The greatest danger, however, comes in being completely unprepared, which is fairly close to the current state of affairs. We have the beginnings — with not only the Inouye telescope, but the Parker Solar Probe and our Sun-monitoring satellites located at the L1 Lagrange point in space — of the infrastructure necessary to detect and measure these events, but the requisite mitigations are not in place at all.
In a worst-case scenario, the flare would arrive during a cold snap affecting the Northern Hemisphere during its winter. It would knock power offline for the majority of the developed world, leaving billions without heat or power. The storage and distribution of food and water might be knocked out, leaving billions to fend for themselves. Our satellite systems could be knocked offline as well; any system that relies on computerized maneuvers to avoid collisions could instead start a catastrophic chain-reaction of satellite impacts in low-Earth orbit. If we fail to prepare, a single event could set us back decades as a civilization.
So what do we do to prepare? It starts with early detection: ground-based and space-based observations of the Sun and of the particles traveling from the Sun to Earth. That would, ideally, mean a network of heliophysics observatories on Earth, at the L1 Lagrange point in space, and in close proximity to the Sun itself. We should prepare power grids for shutdowns and disconnections that take fewer than ~14 hours to execute, and increase grounding at stations and substations. We should create mandatory “safe mode” orbits for satellites, so that electronics disruptions won’t be catastrophic, and create emergency plans for citizens in the event that a Carrington-level flare occurs and heads towards Earth.
In a very real sense, the danger is definitely coming; it’s only a question of when. If we do nothing to prepare, when “the big one” hits, we can look forward to trillions of dollars worth of infrastructure damage and, quite possibly, an enormous number of deaths. But if we can prepare our power grid, distribution system, and global citizens to be ready for the inevitable, we truly have the capacity to effectively survive even a Carrington-type event. We just need to make the effort and the investment in prevention. Otherwise, we’ll be paying for it many times over, for years or even decades to come.
Send in your Ask Ethan questions to startswithabang at gmail dot com!
Starts With A Bang is written by Ethan Siegel, Ph.D., author of Beyond The Galaxy, and Treknology: The Science of Star Trek from Tricorders to Warp Drive.