Ask Ethan: How Old Are The Most Distant Stars We Can See?
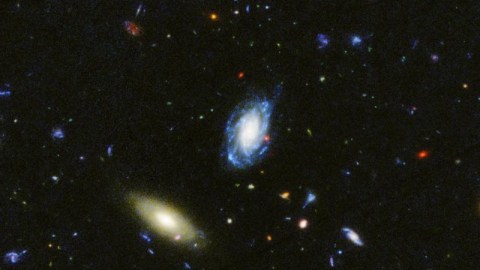
Some of them may have formed just 200 million years after the Big Bang.
When we look out at the Universe, we’re not seeing objects as they are today, but rather as they were when the light that reaches us today was emitted. The closest star to us, Proxima Centauri, is located 4.24 light-years away, and therefore appears to us as it was 4.24 years ago: when its light was emitted. For stars located even farther away, however, we also have to take into account the expansion of the Universe when we look back at them. And the stars we’re seeing were also formed some time ago: Proxima Centauri was born 4.85 billion years ago, making it even older than our Sun How do we put this together to determine the age of stars located all across the Universe? That’s what Sharika Hafeez wants to know, asking:
“I know that the Universe is 13.8 billion years old, and that the observable universe is 46.5 billion light years across. But what is the relationship between the two? When we observe a star, we can find out the distance it is from us, but how do we know its age?”
It’s a great question, and it requires us to put two very different pieces of information together. Here’s how we do it.
When we look at stars in the very nearby Universe, such as in our own galaxy or many of the nearest galaxies, we’re capable of measuring properties of the stars on an individual basis. Not only that, but one of the properties — the star’s current distance — is practically identical to the light-travel-time of the starlight. In other words, stars like Proxima Centauri, located 4.24 light-years away, will see their starlight arrive at our eyes after a journey through space of precisely 4.24 years.
However, those two pieces of information are only true for stars that are in the relatively nearby Universe. As we look to greater and greater distances, we can no longer resolve properties of stars individually, as our telescope resolution peters out before we’ve ever left our local supercluster. Additionally, once we leave the Local Group, we have to reckon with the fact that the fabric of space itself is expanding, not only stretching the wavelength of light (causing it to redshift), but leading to a discrepancy between the distance to an object (measured in light-years) and the light-travel-time to that same object (measured in years).
The first thing we have to realize is that when we look out at a distant object in the Universe, we’re looking back in time. Sure, if you look at a star that’s only a few light-years away, or maybe even a few thousand or hundreds of thousands of light-years away, it will take about the same number of “years” for that starlight to arrive at your eyes as the star is distant in terms of light-years. But once you venture to galaxies that are tens of millions of light-years distant, the expansion of the Universe starts to make a big difference.
The reason is this: light, once it leaves the source, will travel outward in all directions. The light that travels along your line-of-sight to that source will eventually arrive at your eyes (or your telescope’s eyes), but only after it travels through all the space in between you and the emitting source. It’s sort of like imagining that you have a bunch of raisins in a leavening loaf of bread; as the bread leavens, the dough expands and the raisins all get farther apart. The ones that start close by one another only expand a little bit relative to one another, but the ones that start farther away can wind up extremely far away by the time that a signal, such as light, completes its journey.
What this means — the fact that the Universe is expanding — is that the longer light takes to reach us, the larger the discrepancy is between the light-travel-time and the current distance to the object, in light-years. Because we know the combination of what the Universe is made out of (a mix of normal matter, dark matter, and dark energy) and how quickly the Universe is expanding today, we can perform the necessary calculations to determine how the Universe has expanded over its entire history.
This is a remarkably powerful technique, because it has so little wiggle-room. In the Universe today, so long as it’s governed by the laws of General Relativity, there’s an exact relationship between what the Universe is made out of and how quickly it will expand over time. By measuring the combination of distance to and redshift of a variety of cosmic objects to unprecedented precision, we were able to determine this combination, something that was later confirmed by both cosmic microwave background and large-scale structure measurements.
What this teaches us is that we can look back at an object, know how far back in time we’re looking, and also know how far away that object is from us today. For a few examples:
- Looking back at an object whose light requires 100 million years to reach us means we’re seeing an object that’s presently 101 million light-years away.
- When we look back at an object whose light takes 1 billion years to reach us, that object is now 1.035 billion light-years away.
- If light takes 3 billion years to reach us, it means the object is now 3.346 billion light-years away.
- Light that arrives after a 7 billion year journey comes from an object that’s now 9.28 billion light-years away.
- Light that takes 10 billion years to journey to us corresponds to an object now 15.8 billion light-years away.
- Light that requires 12 billion years to arrive at our eyes comes from an object now 22.6 billion light-years distant.
- And light from the most distant object ever detected, galaxy GN-z11, which took 13.4 billion years to arrive in the eyes of the Hubble Space Telescope, is now a whopping 32.1 billion light-years away.
When we measure a distant object, what we’re directly measuring is usually some version of its brightness and how much its light is redshifted, and that’s enough to determine both its current distance and its light-travel-time. When we measure light from an object that’s 32.1 billion light-years away, we’re seeing light as it was from 13.4 billion years ago: just 407 million years after the Big Bang.
But that’s not good enough to teach us how old the stars are in that galaxy; that only shows us how old the light is. To get the second part of the story — to know how old the stars are that are creating this distant light — what we’d ideally want to do is measure the exact properties of the individual stars. We can do this for stars in our own galaxy, and with the highest-resolution telescopes of all, we can identify individual stars up to about 50 or 60 million light-years away. Unfortunately, that barely takes us 0.1% of the way towards the edge of the observable Universe; beyond that point, we can no longer resolve individual stars.
When we’re able to measure individual stars, we can construct what’s known in astronomy as a color-magnitude diagram: we can plot how intrinsically luminous a star is against what its color/temperature is, which is incredibly useful. When stars first form, they make roughly a snaking diagonal line, where the brightest stars are also the bluest and hottest, while the fainter stars are redder and cooler. The youngest populations of stars have a wide mix of stars of all these different color/brightness combinations.
But as the stars age, the hottest, bluest, brightest stars burn through their fuel the fastest, and begin to die off. They die by evolving into red giants and/or supergiants, but this means that the stellar populations start to evolve as the stars age. So long as we can resolve individual stars — in open clusters, in globular clusters, and even in nearby galaxies outside the Milky Way — we can pin down precisely how old a stellar population is. When you combine that with the information we’ve gained about how old the light we’re receiving is, we can finally conclude how old a stellar population is.
But what do we do do when we can no longer observe the individual stars within a galaxy? Do we have any way of estimating the age of the stars inside based on the light we can observe, even if we cannot resolve the stars themselves?
We can use, instead, a proxy for this information that we no longer have, but at the cost of accuracy in translating the age of the stars inside. When we look out at a distant object, like an unresolved (or barely-resolved) galaxy, we can still measure the total starlight coming from those objects. We can still break that light up into different wavelengths and determine how much of the light — intrinsically, accounting for the redshifting that occurs due to the Universe’s expansion — is ultraviolet, blue, green, yellow, red, infrared, etc.
In other words, just by making accurate measurements of a distant galaxy’s color, we can come up with an estimate for how recently it last had a major episode of star-formation, giving us a figure for the ages of the stars within them.
The fact that we have to make these estimates, however, means that we introduce uncertainties. A galaxy that had multiple episodes of star formation over the span of hundreds of millions of years might give a very different figure from a galaxy that had a single major merger were it formed stars all at once. The errors can be as small as a few tens of millions of years, for galaxies that are extremely blue, to as large as 1-to-2 billion years, for galaxies that have a dearth of young, blue stars in them.
There are other methods than can be applied, such as surface brightness fluctuations (which depends on variable stars, which in turn depends on the age of the stars inside) but most of them fail to be useful beyond a certain distance. However, when we can obtain spectroscopic measurements, rather than just measuring the brightness through a variety of color channels (through photometric measurements), we can do a little bit better. By measuring the strength of various atomic and molecular transition — through absorption and emission lines — we can determine where a stellar population is in terms of its age since its most recent burst of star formation.
If you want to know how old the stars are that you’re looking at, there are two things you need to know.
- You need to know how old the light is that you’re looking at, which means you need to know how far away the object is in the context of our expanding Universe.
- You need to know how old the stars themselves are, dating back from the moment that you’re collecting their light.
When you can resolve individual stars, this is a pretty straightforward problem, but we can only resolve individual stars out to distances of around 50–60 million light-years. By contrast, the observable Universe goes out to some 46 billion light-years in all directions, which means we cannot use that method for the overwhelming majority of stars in the Universe. We can only use proxies — such as age estimates based on the colors of galaxies themselves — which introduce extra uncertainties. With a better understanding of stars and stellar evolution, as well as superior instruments and telescopes coming online in the near future, we are hopefully poised to better understand even the most distant, ancient objects of all.
Send in your Ask Ethan questions to startswithabang at gmail dot com!
Ethan Siegel is the author of Beyond the Galaxy and Treknology. You can pre-order his third book, currently in development: the Encyclopaedia Cosmologica.