Ask Ethan: How Does The Event Horizon Telescope Act Like One Giant Mirror?
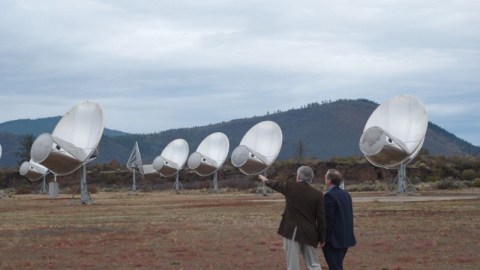
It’s made up of scores of telescopes at many different sites across the world. But it acts like one giant telescope. Here’s how.
If you want to observe the Universe more deeply and at higher resolution than ever before, there’s one tactic that everyone agrees is ideal: build as big a telescope as possible. But the highest resolution image we’ve ever constructed in astronomy doesn’t come from the biggest telescope, but rather from an enormous array of modestly-sized telescopes: the Event Horizon Telescope. How is that possible? That’s what our Ask Ethan questioner for this week, Dieter, wants to know, stating:
I’m having difficulty understanding why the EHT array is considered as ONE telescope (which has the diameter of the earth).
When you consider the EHT as ONE radio telescope, I do understand that the angular resolution is very high due to the wavelength of the incoming signal and earth’s diameter. I also understand that time syncing is critical.
But it would help very much to explain why the diameter of the EHT is considered as ONE telescope, considering there are about 10 individual telescopes in the array.
Constructing an image of the black hole at the center of M87 is one of the most remarkable achievements we’ve ever made. Here’s what made it possible.
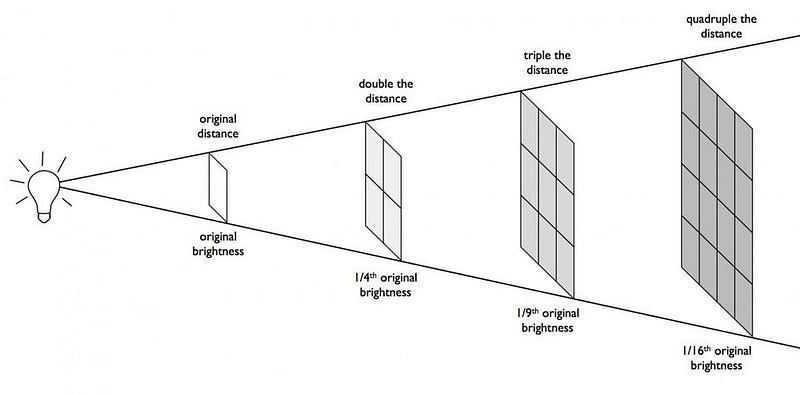
The first thing you need to understand is how light works. When you have any light-emitting object in the Universe, the light it emits will spread out in a sphere upon leaving the source. If all you had was a photo-detector that was a single point, you could still detect that distant, light-emitting object.
But you wouldn’t be able to resolve it.
When light (i.e., a photon) strikes your point-like detector, you can register that the light arrived; you can measure the light’s energy and wavelength; you can know what direction the light came from. But you wouldn’t be able to know anything about that object’s physical properties. You wouldn’t know its size, shape, physical extent, or whether different parts were different colors or brightnesses. This is because you’re only receiving information at a single point.
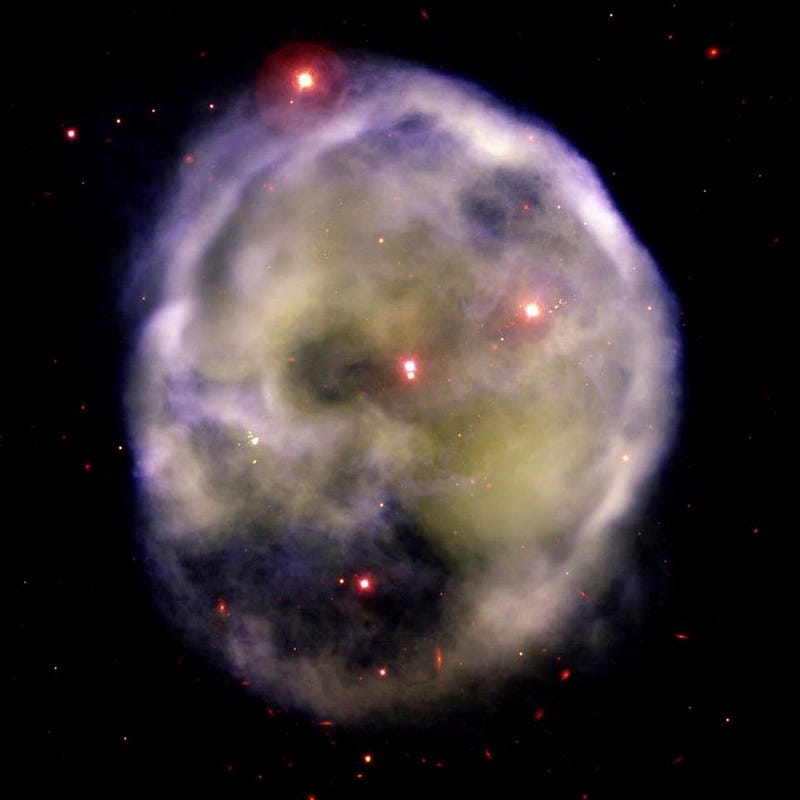
What would it take to know whether you were looking at a single point of light, such as a star like our Sun, or multiple points of light, like you’d find in a binary star system? For that, you’d need to receive light at multiple points. Instead of a point-like detector, you could have a dish-like detector, like the primary mirror on a reflecting telescope.
When the light comes in, it’s not striking a point anymore, but rather an area. The light that had spread out in a sphere now gets reflected off of the mirror and focused to a point. And light that comes from two different sources, even if they’re close together, will be focused to two different locations.
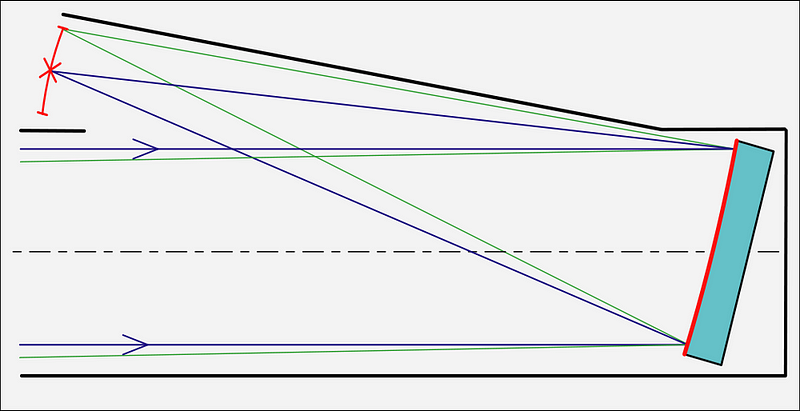
If your telescope mirror is large enough compared to the separation of the two objects, and your optics are good enough, you’ll be able to resolve them. If you build your apparatus right, you’ll be able to tell that there are multiple objects. The two sources of light will appear to be distinct from one another. Technically, there’s a relationship between three quantities:
- the angular resolution you can achieve,
- the diameter of your mirror,
- and the wavelength of light you’re looking in.
If your sources are closer together, or your telescope mirror is smaller, or you look using a longer wavelength of light, it becomes more and more challenging to resolve whatever you’re looking at. It makes it harder to resolve whether there are multiple objects or not, or whether the object you’re viewing has bright-and-dark features. If your resolution is insufficient, everything appears as nothing more than a blurry, unresolved single spot.
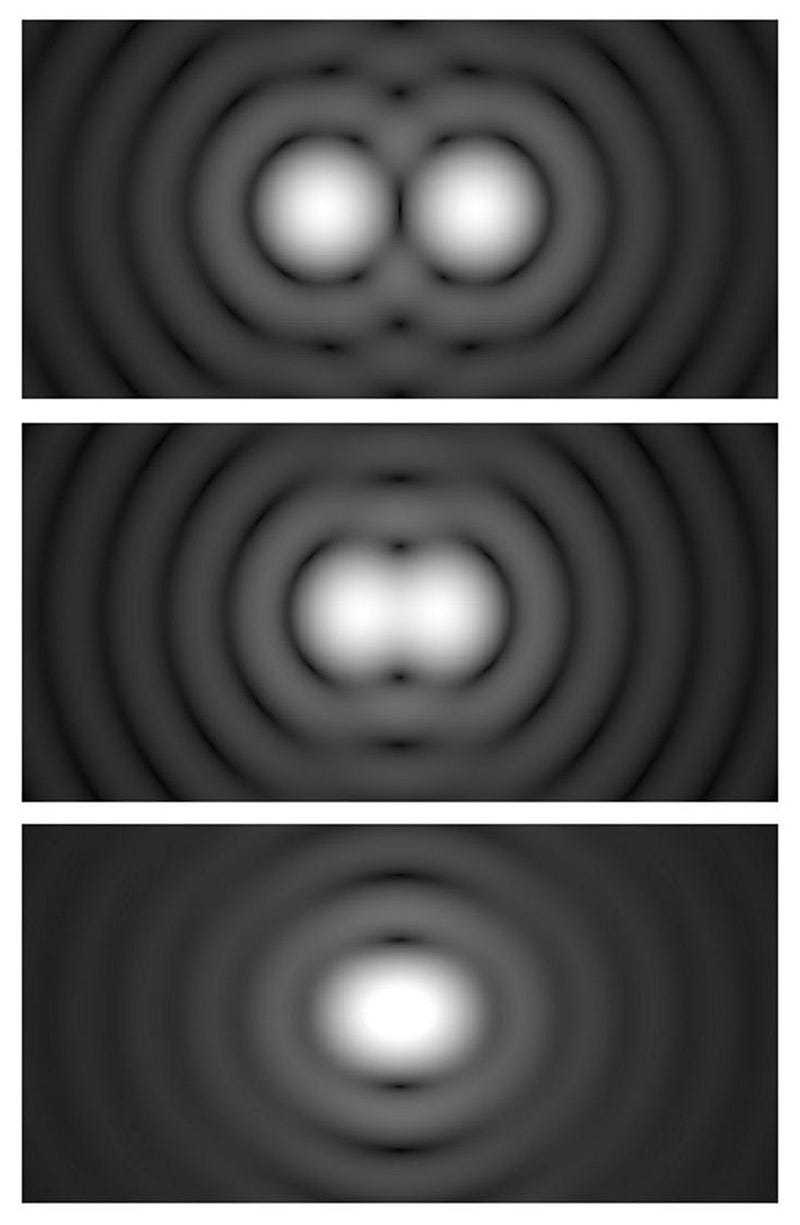
So that’s the basics of how any large, single-dish telescope works. The light comes in from the source, with every point in space — even different points originating from the same object — emitting its own light with its own unique properties. The resolution is determined by the number of wavelengths of light that can fit across our primary mirror.
If our detectors are sensitive enough, we’ll be able to resolve all sorts of features on an object. Hot-and-cold regions of a star, like sunspots, can appear. We can make out features like volcanoes, geysers, icecaps and basins on planets and moons. And the extent of light-emitting gas or plasma, along with their temperatures and densities, can be imaged as well. It’s a fantastic achievement that only depends on the physical and optical properties of your telescope.
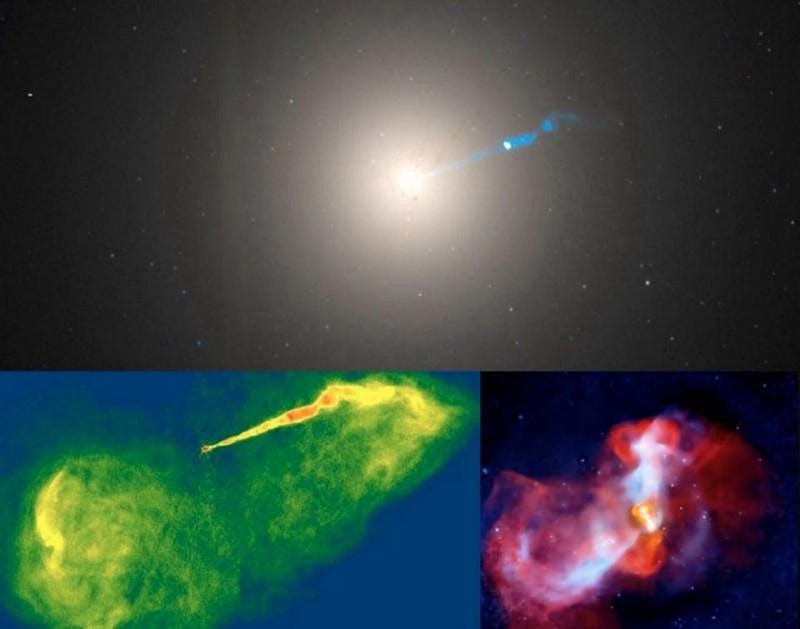
But maybe you don’t need the entire telescope. Building a giant telescope is expensive and resource intensive, and it actually serves two purposes to build them so large.
- The larger your telescope, the better your resolution, based on the number of wavelengths of light that fit across your primary mirror.
- The larger your telescope’s collecting area, the more light you can gather, which means you can observe fainter objects and finer details than you could with a lower-area telescope.
If you took your large telescope mirror and started darkening out some spots — like you were applying a mask to your mirror — you’d no longer be able to receive light from those locations. As a result, the brightness limits on what you could see would decrease, in proportion to the surface area (light-gathering area) of your telescope. But the resolution would still be equal to the separation between the various portions of the mirror.
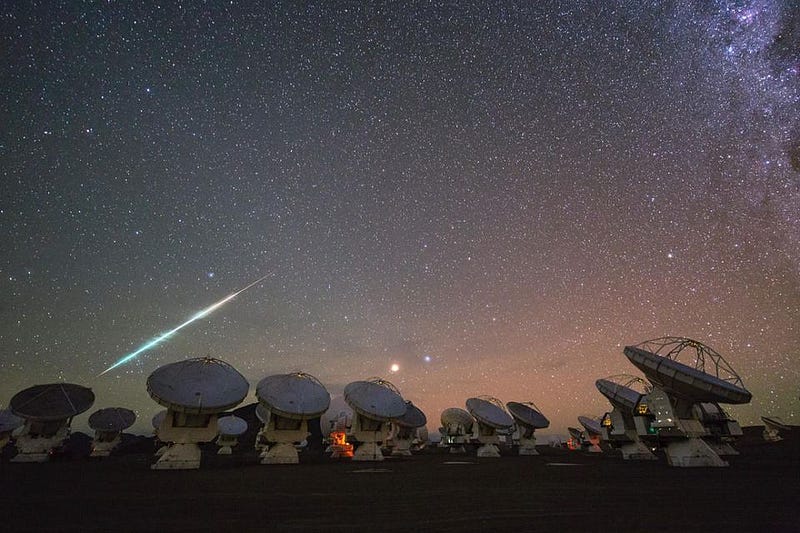
This is the principle on which arrays of telescopes are based. There are many sources out there, particularly in the radio portion of the spectrum, that are extremely bright, so you don’t need all that collecting area that comes with building an enormous, single dish.
Instead, you can build an array of dishes. Because the light from a distant source will spread out, you want to collect light over as large an area as possible. You don’t need to invest all your resources in constructing an enormous dish with supreme light-gathering power, but you still need that same superior resolution. And that’s where the idea of using a giant array of radio telescopes comes from. With a linked array of telescopes all over the world, we can resolve some of the radio-brightest but smallest angular-size objects out there.
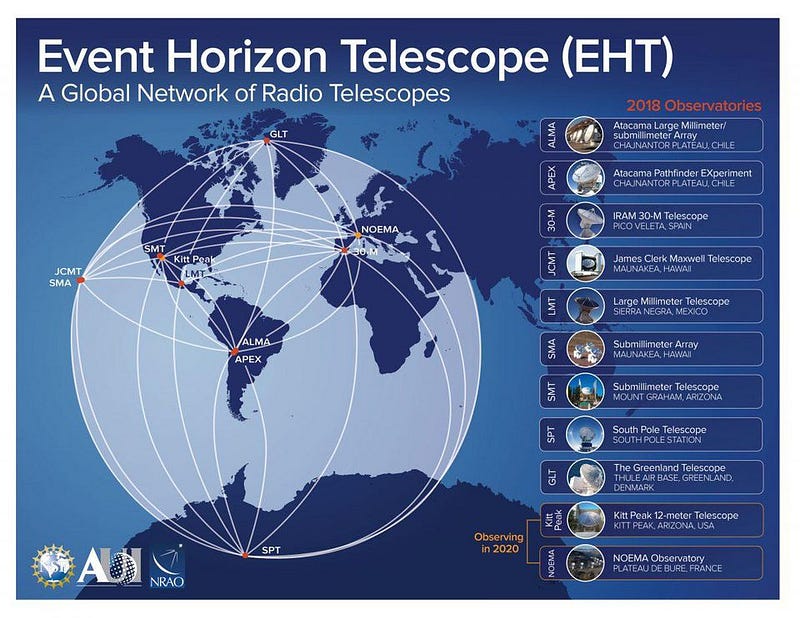
Functionally, there is no difference between thinking about the following two scenarios.
- The Event Horizon Telescope is a single mirror with a lot of masking tape over portions of it. The light gets collected and focused from all these disparate locations across the Earth into a single point, and then synthesized together into an image that reveals the differing brightnesses and properties of your target in space, up to your maximal resolution.
- The Event Horizon Telescope is itself an array of many different individual telescopes and individual telescope arrays. The light gets collected, timestamped with an atomic clock (for syncing purposes), and recorded as data at each individual site. That data is then stitched-and-processed together appropriately to create an image that reveals the brightnesses and properties of whatever you’re looking at in space.
The only difference is in the techniques you have to use to make it happen, but that’s why we have the science of VLBI: very long-baseline interferometry.
You might immediately start thinking of wild ideas, like launching a radio telescope into deep space and using that, networked with the telescopes on Earth, to extend your baseline. It’s a great plan, but you must understand that there’s a reason we didn’t just build the Event Horizon Telescope with two well-separated sites: we want that incredible resolution in all directions.
We want to get full two-dimensional coverage of the sky, which means ideally we’d have our telescopes arranged in a large ring to get those enormous separations. That’s not feasible, of course, on a world with continents and oceans and cities and nations and other borders, boundaries and constraints. But with eight independent sites across the world (seven of which were useful for the M87 image), we were able to do incredibly well.
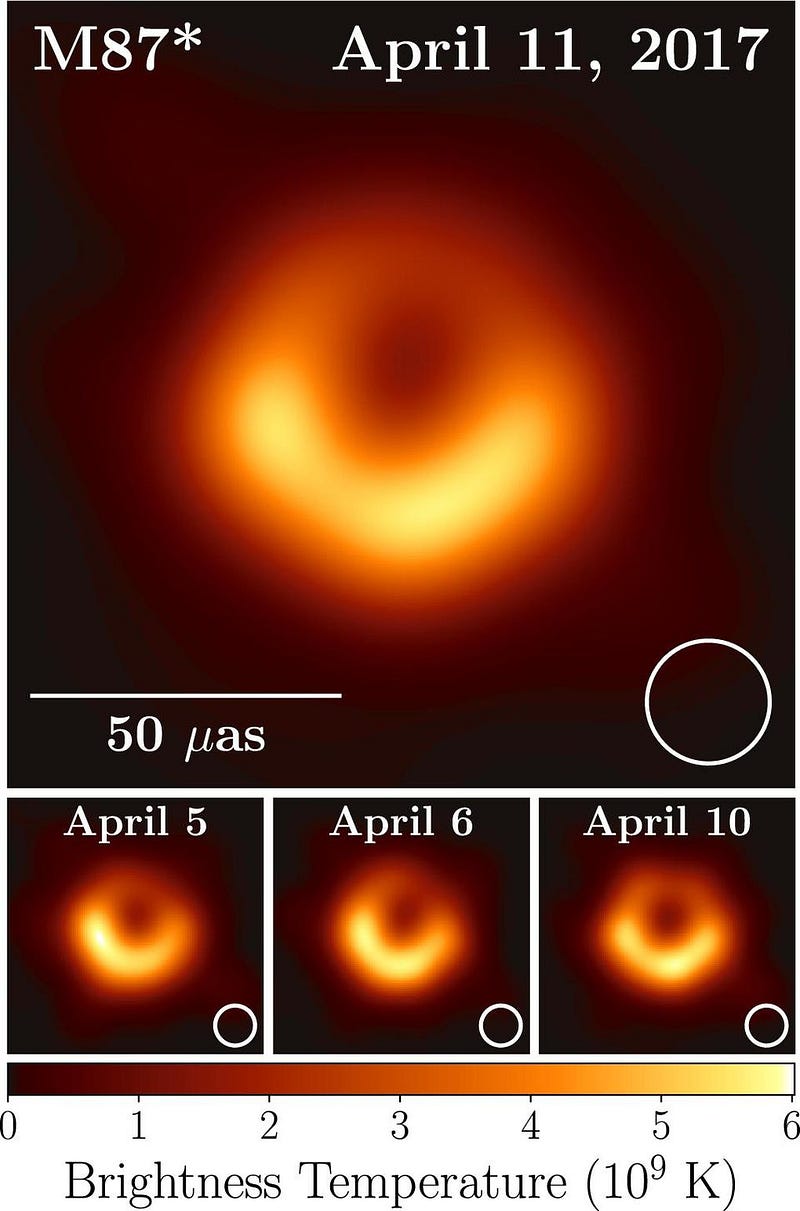
Right now, the Event Horizon Telescope is limited to Earth, limited to the dishes that are presently networked together, and limited by the particular wavelengths it can measure. If it could be modified to observe at shorter wavelengths, and could overcome the atmospheric opacity at those wavelengths, we could achieve higher resolutions with the same equipment. In principle, we might be able to see features three-to-five times as sharp without needing a single new dish.
By making these simultaneous observations all across the world, the Event Horizon Telescope really does behave as a single telescope. It only has the light-gathering power of the individual dishes added together, but can achieve the resolution of the distance between the dishes in the direction that the dishes are separated.
By spanning the diameter of Earth with many different telescopes (or telescope arrays) simultaneously, we were able to obtain the data necessary to resolve the event horizon.
The Event Horizon Telescope behaves like a single telescope because of the incredible advances in the techniques we use and the increases in computational power and novel algorithms that enable us to synthesize this data into a single image. It’s not an easy feat, and took a team of over 100 scientists working for many years to make it happen.
But optically, the principles are the same as using a single mirror. We have light coming in from different spots on a single source, all spreading out, and all arriving at the various telescopes in the array. It’s just as though they’re arriving at different locations along an extremely large mirror. The key is in how we synthesize that data together, and use it to reconstruct an image of what’s actually occurring.
Now that the Event Horizon Telescope team has successfully done exactly that, it’s time to set our sights on the next target: learning as much as we can about every black hole we’re capable of viewing. Like all of you, I can hardly wait.
Send in your Ask Ethan questions to startswithabang at gmail dot com!
Ethan Siegel is the author of Beyond the Galaxy and Treknology. You can pre-order his third book, currently in development: the Encyclopaedia Cosmologica.