Ask Ethan: How Can We See 46.1 Billion Light-Years Away In A 13.8 Billion Year Old Universe?
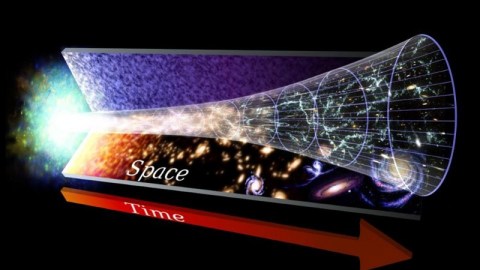
In General Relativity, the fabric of space doesn’t remain static over time. Everything else depends on the details we measure.
If there’s one thing we’ve experimentally determined to be a constant in the Universe, it’s the speed of light in a vacuum, c. No matter where, when, or in which direction light travels, it moves at 299,792,458 meters-per-second, traveling a distance of 1 light-year (about 9 trillion km) every year. It’s been 13.8 billion years since the Big Bang, which might lead you to expect that the farthest objects we can possibly see are 13.8 billion light-years away. But not only isn’t that true, the farthest distance we can see is more than three times as remote: 46.1 billion light-years. How can we see so far away? That’s what Anton Scheepers and Jere Singleton want to know, asking:
If the age of the universe is 13.8 billion years, how can we detect any signal that is more than 13.8 billion light-years away?
It’s a good question, and one that you need a little bit of physics to answer.
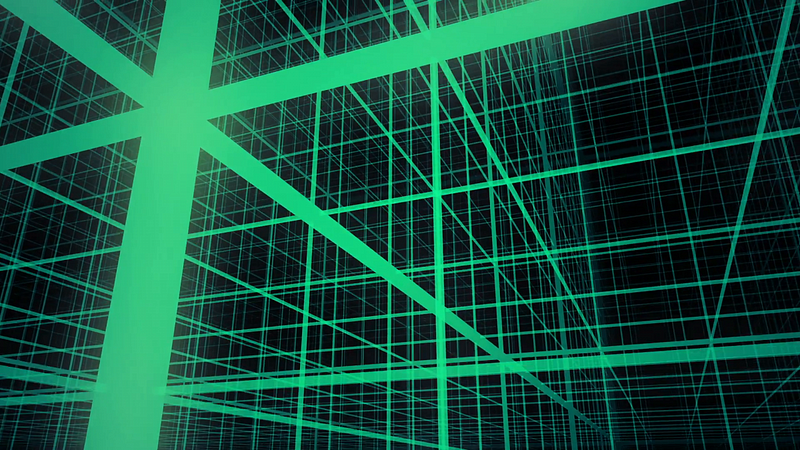
We can start by imagining a Universe where the most distant objects we could see really were 13.8 billion light-years away. For that to be the case, you’d have to have a Universe where:
- objects remained at the same, fixed distance from one another over time,
- where the fabric of space remained static and neither expanded nor contracted over time,
- and where light propagated through the Universe in a straight line between any two points, never being diverted or affected by the effects of matter, energy, spatial curvature, or anything else.
If you imagine your Universe to be a three-dimensional grid — with an x, y, and z axis — where space itself is fixed and unchanging, this would actually be possible. Objects would emit light in the distant past, that light would travel through the Universe until it arrived at our eyes, and we’d receive it the same number of “years” later as the number of “light-years” the light traveled.
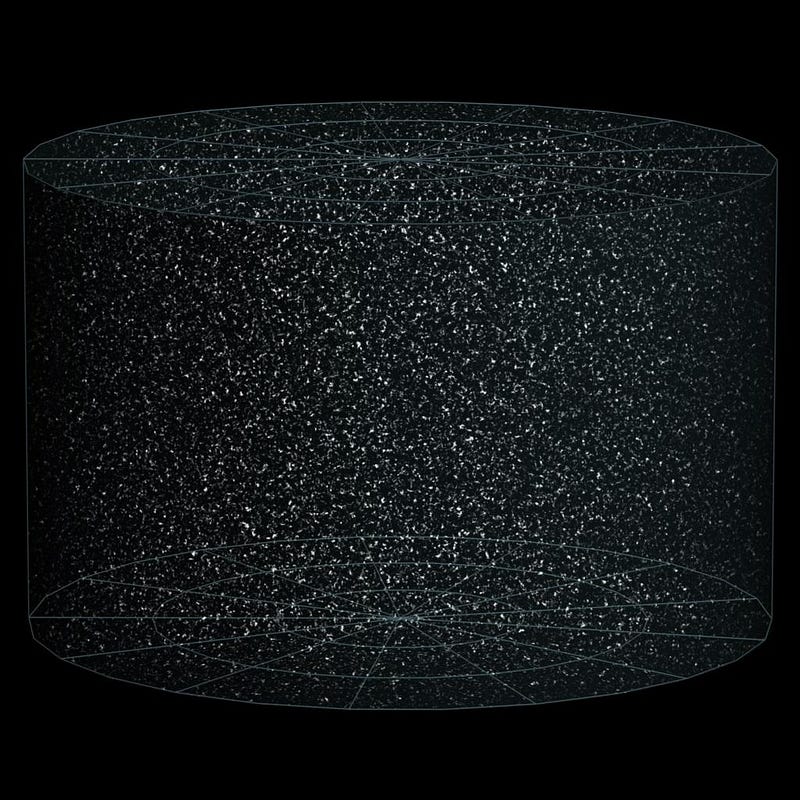
Unfortunately for us, all three of those assumptions are incorrect. For starters, objects don’t remain at a constant, fixed distance from one another, but rather are free to move through the space that they occupy. The mutual gravitational effects of all the massive and energy-containing objects in the Universe cause them to move around and accelerate, clumping masses together into structures like galaxies and clusters of galaxies, while other regions become devoid of matter.
These forces can get extremely complex, kicking stars and gas out of galaxies, creating ultra-fast hypervelocity objects, and creating all sorts of accelerations. The light that we perceive will be redshifted or blueshifted dependent on our relative velocity to the object we’re observing, and the light-travel time won’t necessarily be the same as the actual present-day distance between any two objects.
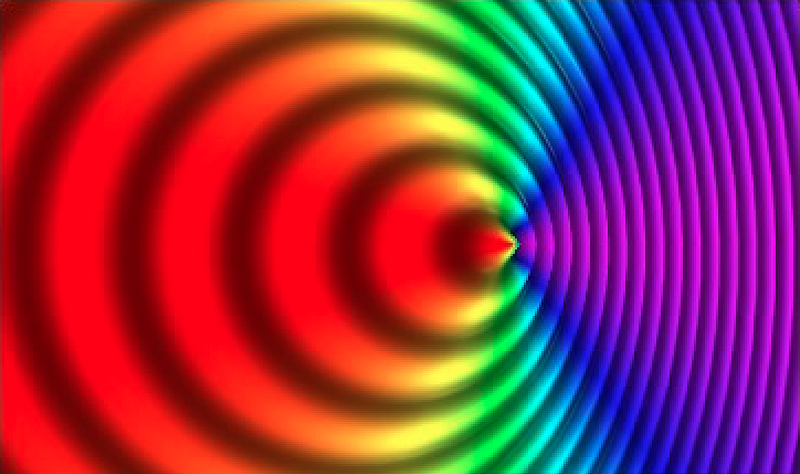
This last point is very important, because even in a Universe where space is static, fixed, and unchanging, objects could still move through it. We can even imagine an extreme case: an object that was located 13.8 billion light-years away some 13.8 billion years ago, but was moving away from us at a velocity very close to the speed of light.
That light will still propagate towards us at the speed of light, traversing 13.8 billion light-years in a timespan of 13.8 billion years. But when that light arrives at the present day, the object can be up to twice as far away: up to 27.6 billion light-years away if it moved away from us arbitrarily close to the speed of light. Even if the fabric of space didn’t change over time, there are plenty of objects we can see today that could be farther away than 13.8 billion light-years.
The only catch is that their light could travel for 13.8 billion light-years at most; how the objects move after emitting that light is irrelevant.
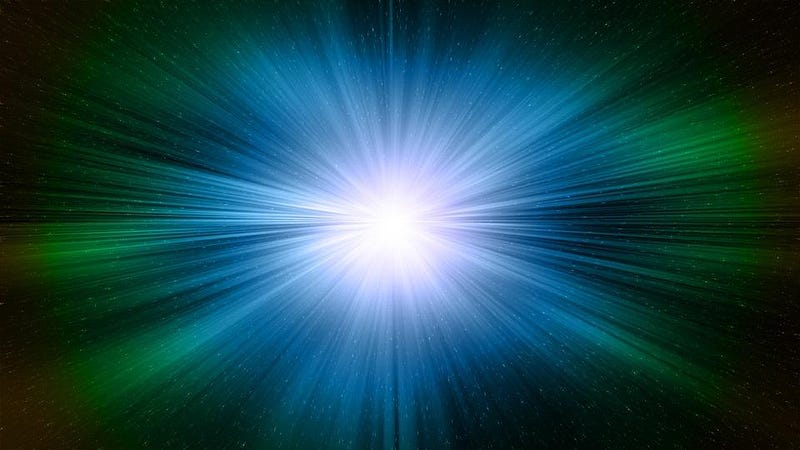
But the fabric of space isn’t constant, either. This was the big revelation of Einstein that led him to formulate the General theory of Relativity: that neither space nor time were static or fixed, but instead formed a fabric known as spacetime, whose properties were dependent on the matter and energy present within the Universe.
If you were to take a Universe that was, on average, filled relatively evenly with some form of matter or energy — irrespective of whether it were normal matter, dark matter, photons, neutrinos, gravitational waves, black holes, dark energy, cosmic strings, or any combination thereof — you would find that the fabric of space itself is unstable: it cannot remain static and unchanging. Instead, it must either expand or contract; the great cosmic distances between objects must change over time.
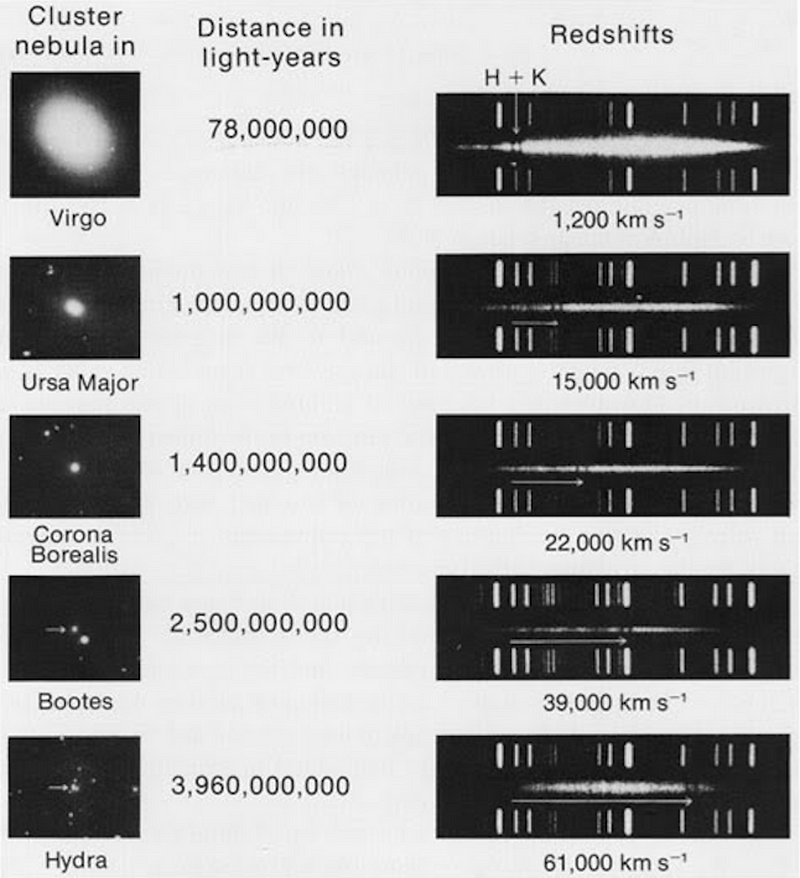
Beginning in the 1910s and 1920s, observations began to confirm this picture. We discovered that the spiral and elliptical nebulae in the sky were galaxies beyond our own; we measured the distance to them; we discovered that the farther away they were, the greater their light was redshifted.
In the context of Einstein’s General Relativity, this led to a surefire conclusion: the Universe was expanding.
This is even more profound than people typically realize. The fabric of space itself does not remain constant over time, but rather expands, pushing objects that aren’t gravitationally bound together apart from one another. It’s as if individual galaxies and groups/clusters of galaxies were raisins embedded in a sea of invisible (space-like) dough, and that as the dough leavened, the raisins were pushed apart. The space between these objects expands, and that causes individual objects to appear to recede from one another.
This has enormous implications for the meaning behind our observations. When we observe a distant object, we don’t just see the light that it emitted, nor do we merely see the light shifted by the relative velocity of the source and the observer. Instead, we see how the expanding Universe has affected that light from the cumulative effects of the expanding space that occurred at every point along its journey.
If we want to probe the absolute limits of how far back we’re able to see, we’d look for light that was emitted as close to 13.8 billion years ago as possible, that was just arriving at our eyes today. We’d calculate, based on the light we see now:
- how much time the light has been traveling for,
- how the Universe has expanded between then and now,
- what all the different forms of energy present in the Universe must be to account for it,
- and how far away the object must be today, given everything we know about the expanding Universe.
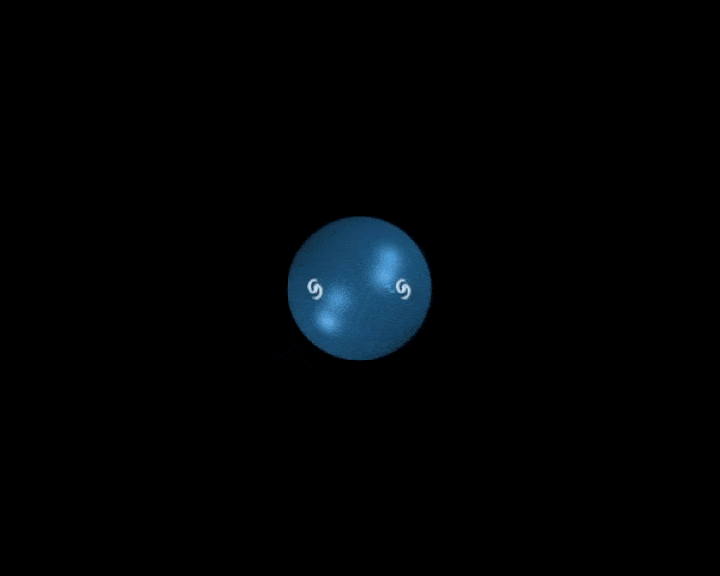
We haven’t just done this for a handful of objects at this point, but for literally millions of them, ranging in distance from our own cosmic backyard out to objects more than 30 billion light-years away.
How can the objects be more than 30 billion light-years away, you ask?
It’s because the space between any two points — like us and the object we’re observing — expands with time. The farthest object we’ve ever seen has had its light travel towards us for 13.4 billion years; we’re seeing it as it was just 407 million years after the Big Bang, or 3% of the Universe’s present age. The light we observe is redshifted by about a factor of 12, as the observed light’s wavelength is 1210% as long as it was compared to when it was emitted. And after that 13.4 billion year journey, that object is now some 32.1 billion light-years away, consistent with an expanding Universe.
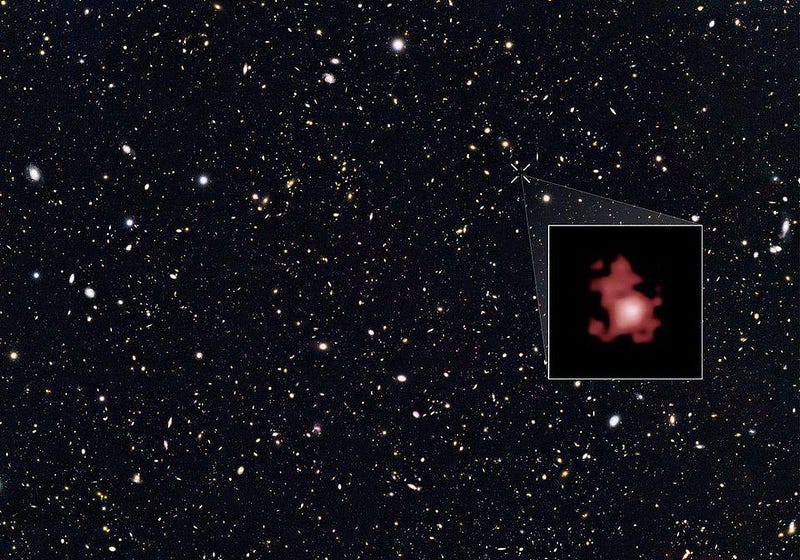
Based on the full suite of observations we’ve taken — measuring not just redshifts and distances of objects but also the leftover glow from the Big Bang (the cosmic microwave background), the clustering of galaxies and features in the large-scale structure of the Universe, gravitational lenses, colliding clusters of galaxies, the abundances of the light elements created before any stars were formed, etc. — we can determine what the Universe is made of, and in what ratios.
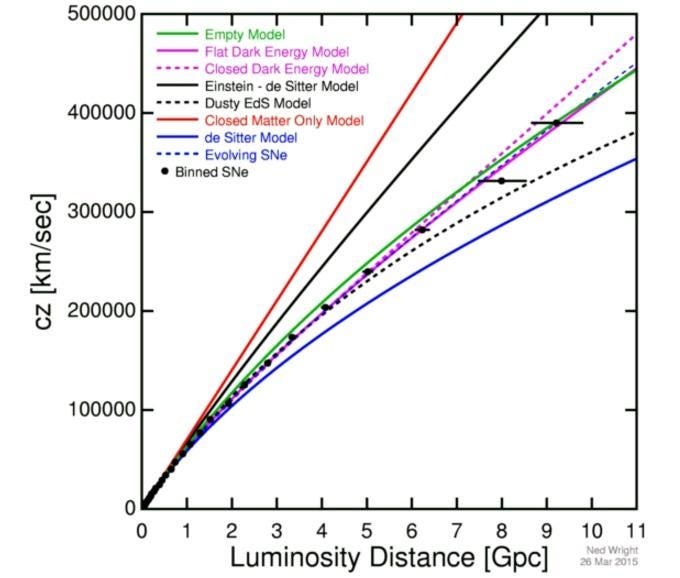
Today, our best estimates are that we live in a Universe made up of:
- 0.01% radiation in the form of photons,
- 0.1% neutrinos, which have a small but non-zero mass,
- 4.9% normal matter, made of protons, neutrons and electrons,
- 27% dark matter,
- and 68% dark energy.
This fits all the data we have, and leads to a unique expansion history dating from the moment of the Big Bang. From this, we can extract one unique value for the size of the visible Universe: 46.1 billion light-years in all directions.
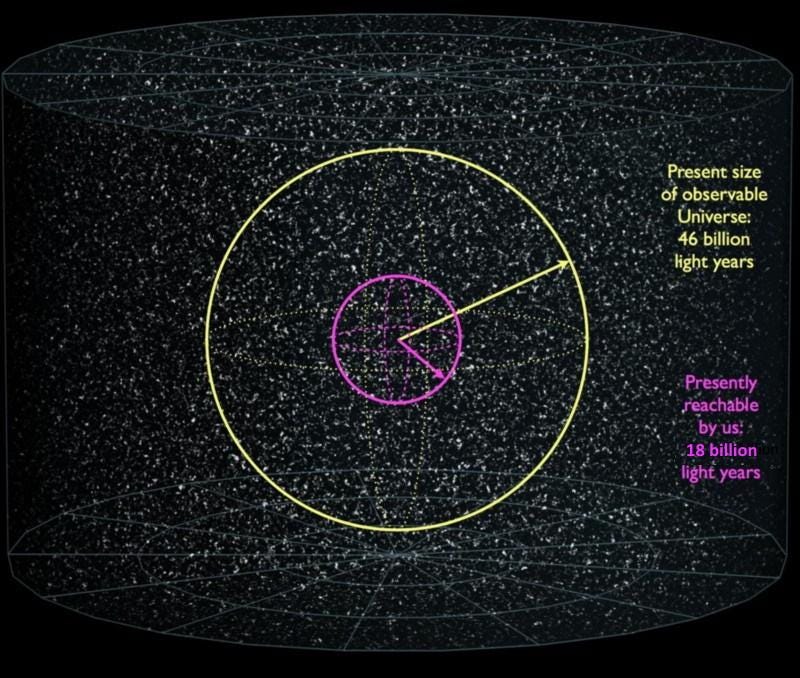
If the limit of what we could see in a 13.8 billion year old Universe were truly 13.8 billion light-years, it would be extraordinary evidence that both General Relativity was wrong and that objects could not move from one location to a more distant location in the Universe over time. The observational evidence overwhelming indicates that objects do move, that General Relativity is correct, and that the Universe is expanding and dominated by a mix of dark matter and dark energy.
When you take the full suite of what’s known into account, we discover a Universe that began with a hot Big Bang some 13.8 billion years ago, has been expanding ever since, and whose most distant light can come to us from an object presently located 46.1 billion light-years away. The space between ourselves and the distant, unbound objects we observe continues to expand at a rate of 6.5 light-years per year at the most distant cosmic frontier. As time goes on, the distant reaches of the Universe will further recede from our grasp.
Send in your Ask Ethan questions to startswithabang at gmail dot com!
Ethan Siegel is the author of Beyond the Galaxy and Treknology. You can pre-order his third book, currently in development: the Encyclopaedia Cosmologica.