Ask Ethan: Could Our Universe Have Collided With Another, Revealing The Multiverse?
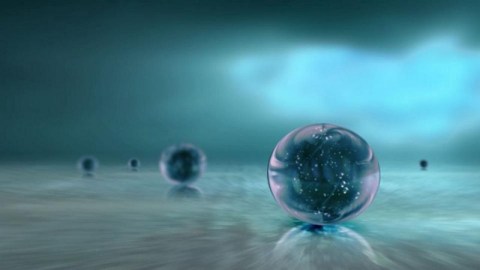
It’s a wild idea, but there’s a way to test it.
No matter how far we look out in the Universe, there’s always more “Universe” to see. Even at the extreme limits of what’s visible — 46 billion light-years in all directions, given the finite amount of time that’s passed since the Big Bang, the expanding Universe, and the finite speed of light — there’s no evidence of all sorts of weirdness we’ve imagined. There’s no edge to the Universe, no departures from large-scale uniformity, no evidence for a preferred direction, and no evidence of repeating patterns. Even so, it’s important to keep our minds open to all the possibilities that aren’t ruled out. After all, “absence of evidence does not imply evidence of absence.”
One of the more fantastic possibilities is that our Universe is just one of many that exist, all of them embedded in a larger Multiverse. If so, could our Universe have collided with another one, possibly leaving an imprint on our own? That’s what Kaden Chan wants us to explore, writing in to ask:
“I remember reading your article about how universes can’t collide due to the theory of cosmic inflation. Then I remember reading somewhere else about how another bubble universe could be created too close to another bubble universe and that could cause a collision. Any thoughts on this, would really like your opinion.”
If we want to know whether these Universes could collide or not, we have to go back to the theory behind it all: cosmic inflation. Let’s see what it says.
Back in the earliest stages of the hot Big Bang, the Universe was:
- incredibly dense, with densities exceeding that of even a neutron star’s core,
- incredibly hot, with energies reaching as high as trillions of times those achieved at CERN’s Large Hadron Collider,
- incredibly uniform, with overdense and underdense regions departing from the average density by only ~0.003%,
- filled with particles and antiparticles, all of which are so energetic they travel at speeds indistinguishable from the speed of light,
- and expanding extraordinarily rapidly.
We also know that there’s a very important relationship between the expansion rate and the sum total of all the various forms of matter and energy present in the Universe: if they balance perfectly, the Universe can expand without either recollapsing or expanding into an empty oblivion; if they don’t, an almost immediate recollapse or an “emptying” of the Universe will occur. We know, looking back from our 13.8 billion year after-the-Big-Bang perspective, that this early balance was indistinguishable from perfect.
Cosmic inflation was the first idea — an add-on to the Big Bang — to explain how this could occur. What inflation postulated was that the reason the total energy density and the expansion rate balanced so perfectly is that the Big Bang wasn’t the very beginning of everything, but rather was preceded by what’s known as an inflationary phase: where there’s no matter, antimatter, or radiation, but rather where the expansion of the Universe is determined by a form of energy inherent to space itself.
This creates an interesting scenario. You see, when your Universe is filled with something like matter, there are a fixed number of particles present: when the volume increases, the density of particles goes down. As a result, when the energy density lowers, the expansion rate lowers too: the two of them must balance. Similarly, with radiation, the density drops too, but even faster; radiation is not only made up of a fixed number of particles, but each one of those particles behaves like a wave, meaning that its wavelength lengthens as the Universe expands, causing the expansion rate to decrease even more quickly than in the matter-filled Universe case.
But for energy inherent to space, the energy density remains constant. Even as the Universe expands, space is still space. And since the energy density balances the expansion rate, the expansion rate doesn’t change if you’re dominated by energy inherent to space itself.
Hypothesizing this early, energy-inherent-to-space state is extremely compelling. If your Universe is expanding as though there’s energy inherent to space, the expansion rate won’t change over time, which means that the expansion will be exponential.
Exponential means the following:
- imagine you have a point located a certain distance away from you,
- and you allow a certain amount of time to pass, until that point’s distance doubles,
- if you allow that amount of time to pass once again, that distance once again doubles, so that it’s four times the original distance,
- and if that time interval passes once again, that distance doubles again, making it eight times the original distance,
- and that if 10 or 100 times that time interval passes, that distance becomes 2¹⁰ or 2¹⁰⁰ times the original distance,
enabling a Universe that inflates in this fashion to be stretched flat, emptied of all pre-existing matter and radiation, and giving it the same properties everywhere, since everything that now occupies our observable Universe emerged from this once-tiny region of inflating space.
It’s only the exponential nature of inflation that enables it to precede and set up the Big Bang; if it were filled with matter or radiation instead, the Universe’s expansion rate would drop as the density diluted, as shown below.
There are, of course, good reasons to believe that inflation occurred beyond explaining these otherwise unexplained puzzles. One is that an inflating Universe, assuming that inflation is a quantum field that experiences quantum fluctuations just like any other field in the Universe, has these quantum fluctuations stretched across the inflating Universe. When inflation ends, the energy inherent to space gets “dumped” into particles: matter, antimatter, radiation, etc., and a number of observable consequences ensue. They include:
- an almost-perfectly scale invariant spectrum of density fluctuations, where the fluctuations on the largest scales are slightly greater in magnitude, by a few percent, than the ones on smaller scales,
- where these fluctuations are 100% adiabatic (with constant entropy) and 0% iscocurvature (with constant spatial curvature),
- where fluctuations on scales larger than the cosmic horizon exist,
- and where there’s an observed maximum upper limit on how hot the Universe could have gotten in the earliest stages of the Big Bang, and that limit is significantly below the Planck energy scale.
All of these predictions have been confirmed, while the end of inflation corresponds to the onset of the hot Big Bang. Our cosmic origins have now been pushed back to before the Big Bang, to this inflationary state.
Of course, this is going to have other consequences as well: consequences that perhaps aren’t as easily observable as these phenomena we’ve been able to test and measure. Some of what inflation predicts, in fact, might forever be unobservable, due to one fact that’s so important it’s worth highlighting:
Inflation, due to the nature of exponential expansion, forever erases from our Universe any signature that was created before the final tiny-fraction-of-a-second of inflation itself.
It’s only those final instants of inflation, where the small region of space that would:
- expand while fluctuating,
- transition from expanding exponentially and being filled with energy-inherent-to-space to expanding like a matter-and-antimatter-and-radiation-filled Universe,
- and lead to a hot Big Bang for a region at least the size of a soccer ball, which was the minimum size for our Universe at the start of the hot Big Bang,
that we can observe. Anything that happened either before that event, or that happened outside of that particular soccer ball-sized region that became our Universe, cannot be observed.
So, according to the theory of inflation, what is it, exactly, that happens?
One of the simplest ways to visualize inflation is to imagine that you have a ball on top of a very flat hill, and that as time goes on, the ball rolls down the hill, on either one side or the other, into the awaiting valley below. Only, instead of this ball being a classical ball — with a well-defined, unambiguous position on the hill — this is a quantum ball, with a probability distribution of where it is that spreads out over time.
Now, here’s a key part of inflation that most people don’t recognize: the hill has to have a certain set of properties so that the ball rolls slowly enough to give us enough inflation to give rise to our Universe as we see it. This places significant and meaningful constraints on the allowed shapes that the hill could possibly have, and in particular, this fact is true: the hill needs to be flat enough that the ball rolls slowly.
What this enables, however, is the quantum field that determines the ball’s position to spread out, and you have to compare “how fast does the ball roll, on average” with “how fast does the ball, due to quantum effects, spread out in its possible locations along the hill?”
Here’s the fun, important lesson to learn: in virtually all models of inflation where the ball rolls slowly enough to get “enough” inflation to be consistent with our Universe, the “quantum spreading” happens faster than the rolling does, especially when you’re on the flat part of the hill.
This means, during inflation, that you’ll get some regions where the field “spreads” closer to the valley, and once you roll into the valley, inflation ends.
But you’ll also get regions where inflation doesn’t end at that time, and spreads back towards the flat part of the hill, where inflation continues for some additional time. In fact, working out the mathematics of this indicates quite strongly that:
- yes, there will always be some regions, during inflation, where the “ball” rolls into the valley,
- that where it does, inflation ends and we get a hot Big Bang,
- but in regions surrounding those “inflation ends” regions, inflation continues,
- and in those regions, space expands exponentially, rather than at the rapidly decreasing rate that occurs in regions where you get a hot Big Bang.
This is very, very important, because that’s precisely what gives rise to the Multiverse: this very property of space under the influence of cosmic inflation.
During inflation, the expansion is relentless: space everywhere expands at the same rapid, constant rate. You can imagine that this space is like a sea of water, and that the sea is expanding: more and more water appears as time goes on.
Then you can imagine that inflation ends in some regions, and these are like little tiny bubbles that form and grow, similar to how a pot of boiling water starts with tiny bubbles that nucleate and grow.
But unlike in a pot of boiling water, the “expanding” nature of the sea drives these individual bubbles apart; they grow, but the expanding sea between them grows relatively faster, ensuring that any two individual bubbles will never collide.
That’s the standard picture of cosmic inflation, along with how and why it creates a Multiverse. In fact, once inflation begins, there will always be regions between any two places where inflation ends that keep on inflating; in this sense, inflation is eternal. However, we can only observe the Universe where we exist within it, and that’s where a hot Big Bang occurred some 13.8 billion years ago. And to no one’s surprise, there’s no evidence of a bubble collision having occurred in the early Universe, as the imprint such an event would have left is nowhere to be seen.
The take home message is this: in order to get enough inflation to explain the Universe we observe, the field responsible for it needs to have certain properties. If it has those properties, we can not only explain everything that the Big Bang without inflation could, we also explained things that the Big Bang alone could not, plus make new predictions that have subsequently been tested and confirmed.
However, there’s another consequence that cannot realistically be avoided that comes along for the ride: a Multiverse, where many independent universes are created within it that never interact, overlap, or collide with one another. We have no evidence that the Multiverse exists, of course; the only variants that would have observable consequences involve “bruises” on our Universe, which are inconsistent with what we see. Everything still lines up with the simplest models of inflation, nearly 40 years after their initial proposal. Perhaps it’s time to stop paying attention to the doubting Thomases out there, and to instead embrace the Universe exactly as it shows itself to be.
Send in your Ask Ethan questions to startswithabang at gmail dot com!
Starts With A Bang is written by Ethan Siegel, Ph.D., author of Beyond The Galaxy, and Treknology: The Science of Star Trek from Tricorders to Warp Drive.