Ask Ethan: Are Quantum Fields Real?

And do they fundamentally describe our entire Universe, or do we require something else?
The Universe we perceive and view, all around us, isn’t representative of what actually exists at a fundamental level. Instead of continuous, solid objects, matter is composed of indivisible quantum particles, held together through invisible forces that act across empty space. Both the particles themselves and the forces can be described by an underlying structure: quantum fields, which describe everything we know about all the particles and antiparticles of the Standard Model. But are these quantum fields real? And just what do they tell us? That’s what Patreon supporter Aaron Weiss wants to know, as he asks:
I would be very interested in a post about quantum fields. Are they generally/universally believed to be real and the most fundamental aspect of our universe or just a mathematical construct? I’ve read that there are 24 fundamental quantum fields: 12 fields for fermions and 12 for bosons. But I’ve also read about quantum fields for atoms, molecules, etc. How does that work? Does everything emerge from these 24 fields and their interactions?
Let’s start with what a quantum field actually is.
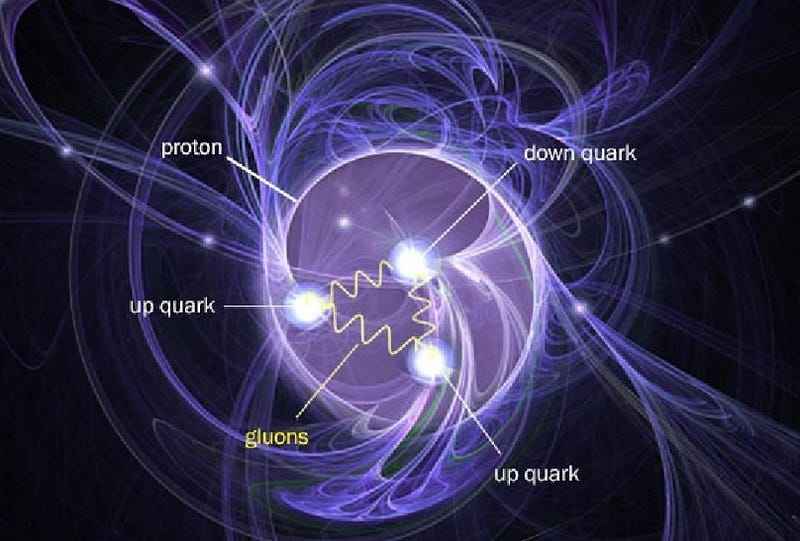
In physics, a field, in general, describes what some property of the Universe is everywhere in space. It has to have a magnitude: an amount that the field is present. It may or may not have a direction associated with it; some fields do, like electric fields, some don’t, like voltage fields. When all we had were classical fields, we stated that the fields must have some kind of source, like particles, which results in the fields existing all throughout space.
In quantum physics, though, this seemingly self-evident fact is no longer true. Whereas classical physics defines quantities like position and momentum as properties of a particle, and those properties would generate a corresponding field, quantum physics treats them differently. Instead of quantities, position and momentum (among other quantities) now become operators, which allow us to derive all the quantum weirdness you’ve heard so much about.
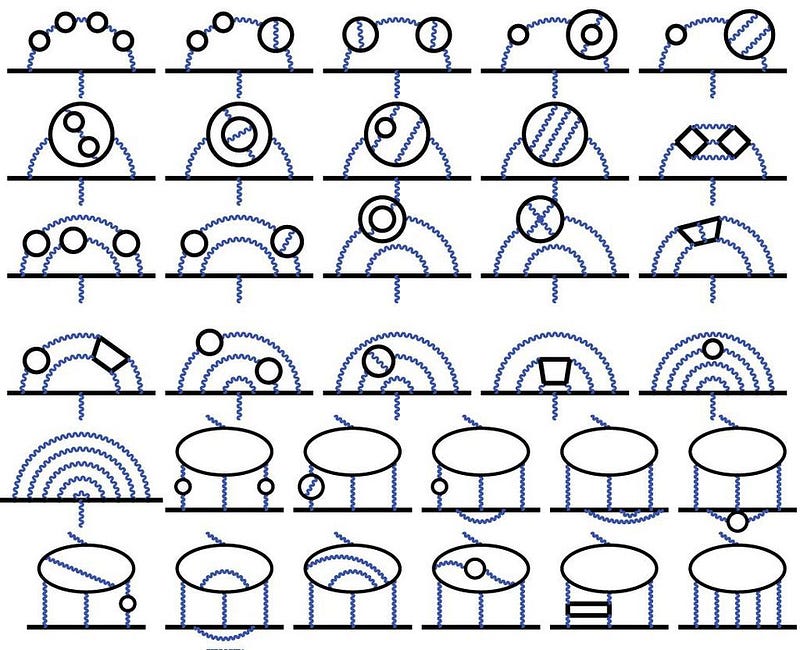
A quantity like an electron no longer has a well-defined position or momentum, but rather a wavefunction that describes the probability distribution of all possible positions and momenta.
You may have heard these words before, but have you ever thought about what that actually means?
It means that the electron isn’t a particle at all. It’s not something you can put your finger on and declare, “the electron is here, moving with this particular speed in this particular direction.” You can only state what the overall properties are, on average, of the space in which the electron exists.
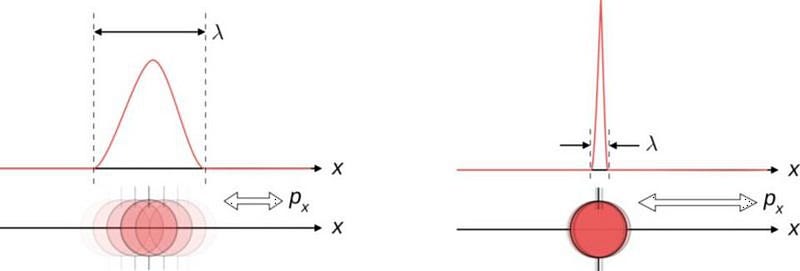
That doesn’t sound very particle-like, does it? In fact, that sounds more field-like: some property of the Universe everywhere in space. That’s because, in quantum field theory (QFT), quantum fields aren’t generated by matter. Instead, what we interpret as “matter” is itself a quantum field.
And these quantum fields, themselves, are made up of particles.
- The electromagnetic field? Made of particles called photons.
- The strong nuclear field, that holds protons and neutrons together? Made up of particles called gluons.
- The weak nuclear field, responsible for radioactive decays? Made of particles called W-and-Z bosons.
- Even the gravitational field, if we try and formulate a quantum version of gravity? Made of particles called gravitons.
Yes, even the gravitational waves that LIGO detected, as smooth and continuous as they appeared, should be made of individual quantum particles.
The reason we can use these terms of particles and fields interchangeably, in QFT, is because the quantum fields themselves encode all the information for everything. Have a particle and antiparticle annihilating? That’s described by equal-and-opposite excitations of a quantum field. Want to describe the spontaneous creation of particle-antiparticle pairs of particles? That’s also due to excitations of a quantum field.
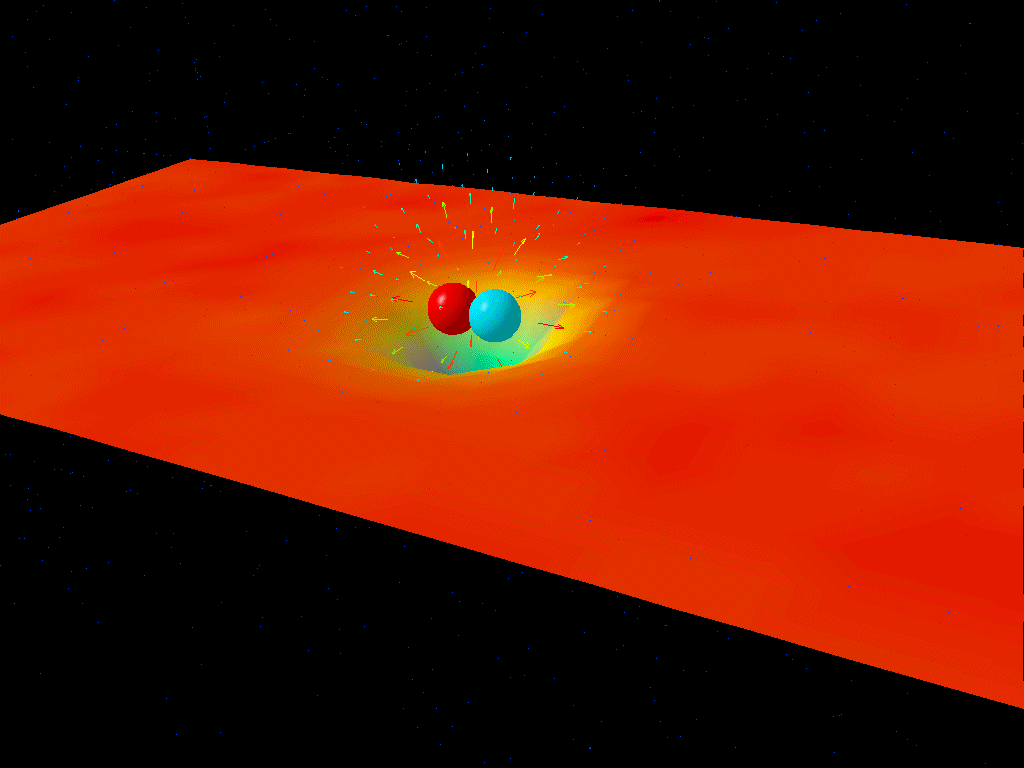
Even particles themselves, like electrons, are just excited states of a quantum field. Every particle in the Universe, as we understand it, is a ripple, or excitation, or bundle-of-energy, of the underlying quantum field. This is true for the quarks, the gluons, the Higgs boson, and for all the other particles of the Standard Model.
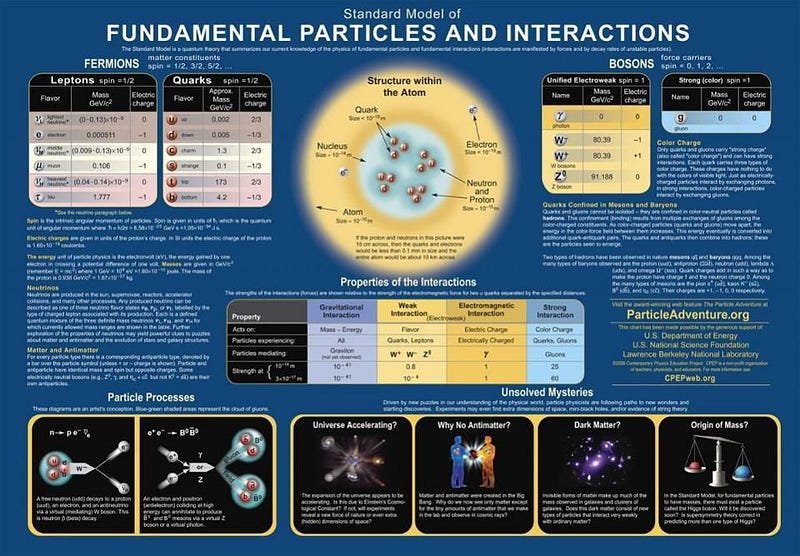
So how many fundamental quantum fields are there? Well, that depends on how you look at the theory. In the simplest QFT that describes our reality, the quantum electrodynamics of Julian Schwinger, Shinichiro Tomonaga and Richard Feynman, there are only two quantum fields: the electromagnetic field and the electron field. They interact; they transfer energy and momentum and angular momentum; excitations are created and destroyed. Every excitation that’s possible has a reverse excitation that’s also possible, which is why this theory implies the existence of positrons (antimatter counterparts of electrons). In addition, photons exist, too, as the particle equivalents of the electromagnetic field.
When we take all the forces that we understand, i.e., not including gravity, and write down the QFT version of them, we arrive at the predictions of the Standard Model.
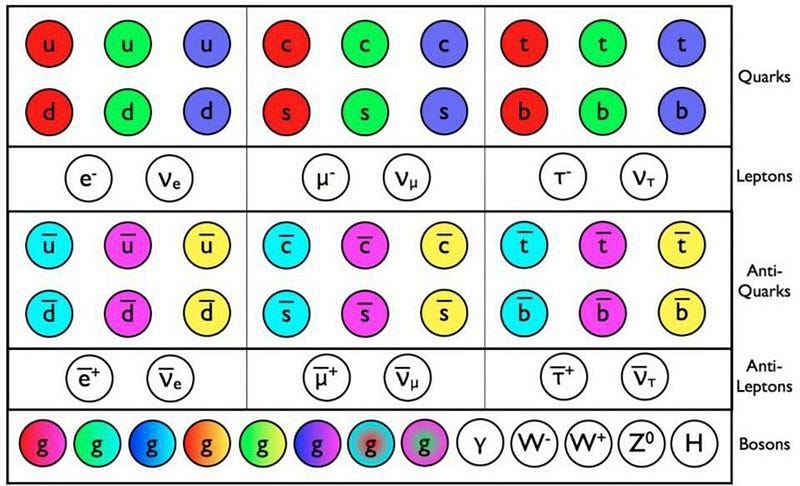
This is where the idea of 12 fermion fields and 12 boson fields come from. These fields are excitations of the underlying theories (the Standard Model) that describe the known Universe in its entirety, and include:
- The six (up, down, strange, charm, bottom, top) quarks, and their antiquark counterparts,
- The three charged (electron, muon, tau) and three neutral (electron neutrino, muon neutrino, tau neutrino) leptons, and their antimatter counterparts,
- The eight gluons (because of the eight possible color combinations),
- The two weak (W-and-Z) bosons,
- The one electromagnetic (photon) boson,
- And the Higgs boson.
The quarks and leptons are fermions, which is why they have antimatter counterparts, and the W boson comes in two equal-and-opposite varieties (positively and negatively charged), but all told, there are 24 unique, fundamental excitations of quantum fields possible. This is where the “24 fields” idea comes from.
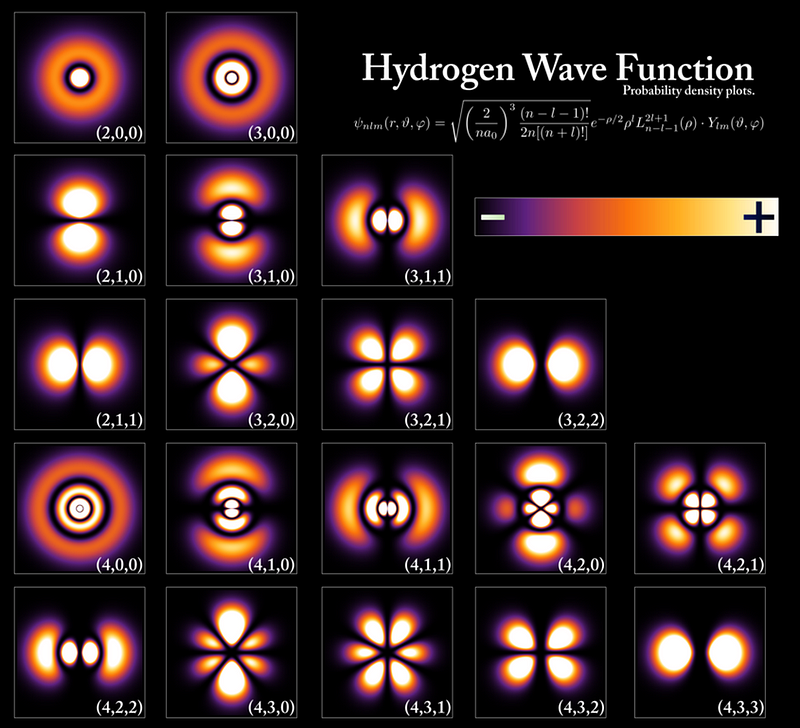
So what about complex systems, then, like protons, atoms, molecules, and more? You have to understand that just as the 24 fields are actually excitations of the underlying QFT that describes our physical reality, these complex systems are more than just combinations of these fields put together into some stable or quasi-stable bound state.
Instead, it’s more accurate to view the entire Universe as a complicated quantum field that, itself, contains all of physics. Quantum fields can describe an arbitrarily large number of particles that interact in all ways our theories can conceivably allow. And they do this not in some vacuum of empty space, but amidst a background of not-so-empty-space, which plays by the rules of QFT, too.
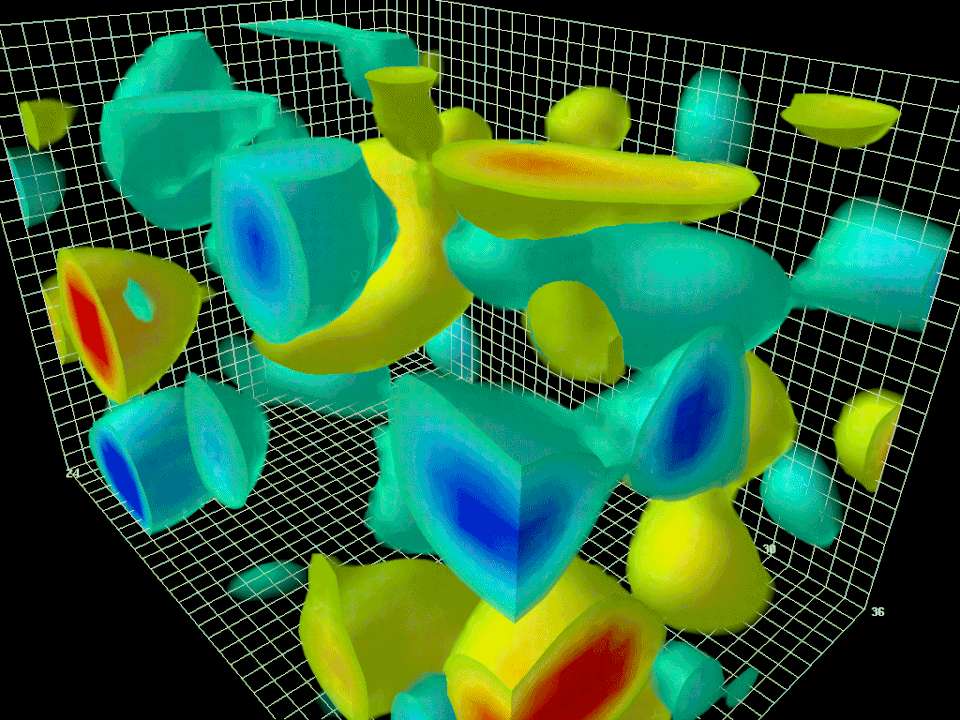
Particles, antiparticles, and all sorts of excitations of the fields are constantly being created-and-destroyed. Reality is fundamentally different from our classical picture of a smooth, continuous, well-defined Universe. Although it’s true that these quantum fields began as a mathematical construct, they describe our physical, observable reality more accurately than any other theory we’ve concocted. They allow us to make incredibly precise predictions about what the results of any experiment involving the quanta of the Standard Model will yield: predictions that have been borne out by every experiment sensitive enough to test them.
The Universe may not be an intuitive place, but as far as any physical theory can call itself reflective of reality, QFT has no equal in terms of its power. As long as physics remains an experimental science, this will be the standard that any candidate theory will have to supersede.
Send in your Ask Ethan questions to startswithabang at gmail dot com!
Ethan Siegel is the author of Beyond the Galaxy and Treknology. You can pre-order his third book, currently in development: the Encyclopaedia Cosmologica.