Ask Ethan: Are Gravitational Waves Themselves Affected By Gravity?
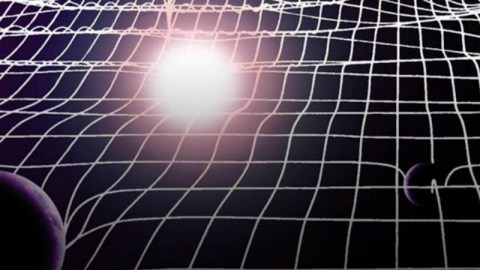
When you emit gravitational waves, they have to pass through the Universe. But do they gravitate, too?
When you travel through the Universe, it isn’t just a free ride through empty space. Although you might not think about it very often, there are forces arising from the presence of everything else, and those forces play a major role. The electric charges, the nuclear forces, and the gravitational distortion of spacetime itself — caused by all the masses and forms of energy present within the visible Universe — affect your motion. But what if you weren’t made of atoms; what if, instead, you were a gravitational wave? Would you still experience those forces in the same way? That’s the question of Patreon supporter Darren Redfern, who asks:
Are gravitational waves themselves subject to gravity? That is, if a gravitational wave were to pass by a galaxy cluster, would its form get distorted (even though the wave, itself, is a distortion of space-time)? One side of me says gravitational waves are a form of energy so therefore must be affected by gravity. The other side of me says “Nah — that just doesn’t make sense!”
The Universe isn’t obligated to make sense. But it does have rules that it’s obligated to follow. Let’s see what they say.
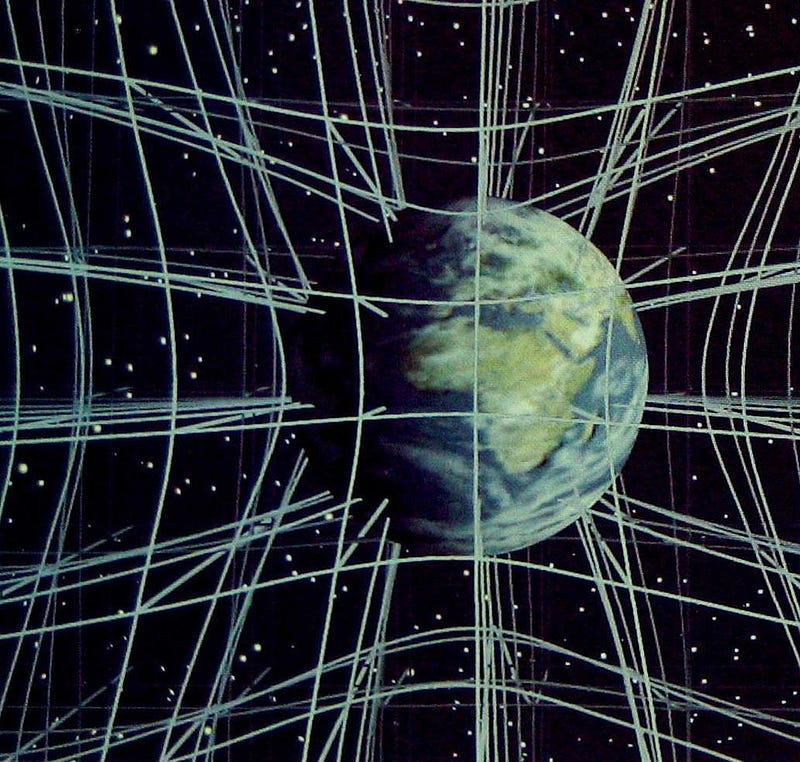
When it comes to General Relativity, the concept of gravity is perhaps simpler than any alternative that’s ever existed. The cardinal rule is this: matter and energy tells spacetime how to curve; the curved spacetime determines how matter and energy move. If you tell me what the particles, antiparticles, and other energy-containing entities are, I can, in principle, tell you how the fabric of the Universe is curved in response.
As the various masses and forms of energy move relative to one another — or as you yourself, active as an observer, move — spacetime will distort in response. At any instant in time, that curved spacetime will determine how you move and accelerate through the Universe. That’s how General Relativity works.
It’s a little counterintuitive, but it doesn’t actually matter what type of particle you are. Whether you’re matter or antimatter; whether you’re massive or massless; whether you’re a fundamental, indivisible particle or a composite one are all irrelevant. The fabric of the Universe is curved, and that curvature is what determines how everything moves through the Universe.
It seems like an open-and-shut case, then. When we look out at a distant galaxy cluster, we know that its mass distorts the fabric of space. When we view the light coming from distant objects either within or beyond that galaxy cluster, we know (and observe) that the light — even though it’s massless — follows the path determined by this curved space.
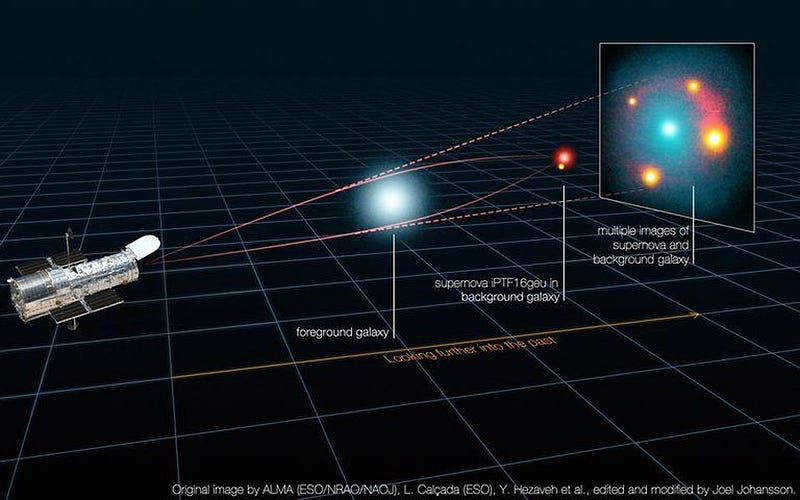
There’s every reason to expect that gravitational waves will behave similarly.
Isn’t there?
They do share a number of properties with photons, including:
- they’re massless,
- they travel at the speed of light,
- and, perhaps most importantly, they do carry energy.
This last part about carrying energy is very important, because that’s what responds to curved spacetime.
Like light, gravitational waves have a wavelength. Like light, they carry an energy that’s defined by their wavelength and intensity/amplitude. And, like light, its wavelength gets stretched as the Universe expands.
This last part allows us to move from the realm of the theoretical into the realm of the observational. We have observed a number of different gravitational waves thanks to LIGO: 11 at last count. These all correspond to merging, massive, compact objects, where even the closest one was over 100 million light years distant. With light-travel-times (or gravitational-wave-travel-times) this large, the expansion of the Universe is important, and when we measure the waves that passed through Earth, we can see that they were definitively stretched by the effects of the Universe’s expansion.
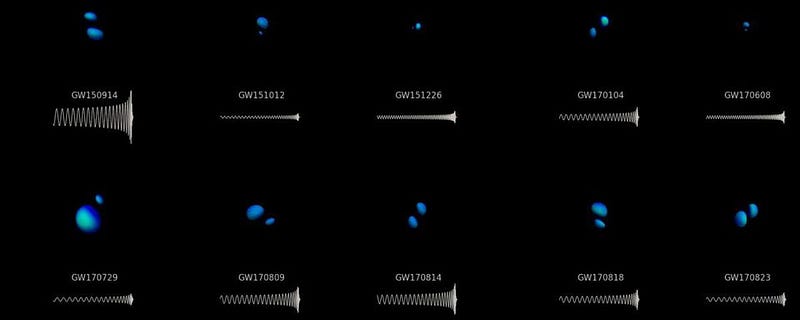
This tells us, unambiguously, that gravitational waves, as they travel through the Universe, are affected by the warping, curvature, and stretching of space.
There’s another piece of evidence, too. The kilonova event of 2017, where we observed the merging of two neutron stars in both gravitational waves and in electromagnetic light, had these two signals arrive nearly simultaneously: with less than a 2.0 second difference between them. Traveling from a distance of over 100 million light years (and given that there are over 30 million seconds in a year), we can state that the speed of light and the speed of gravity are equal to within better than 1 part in a quadrillion (10¹⁵).
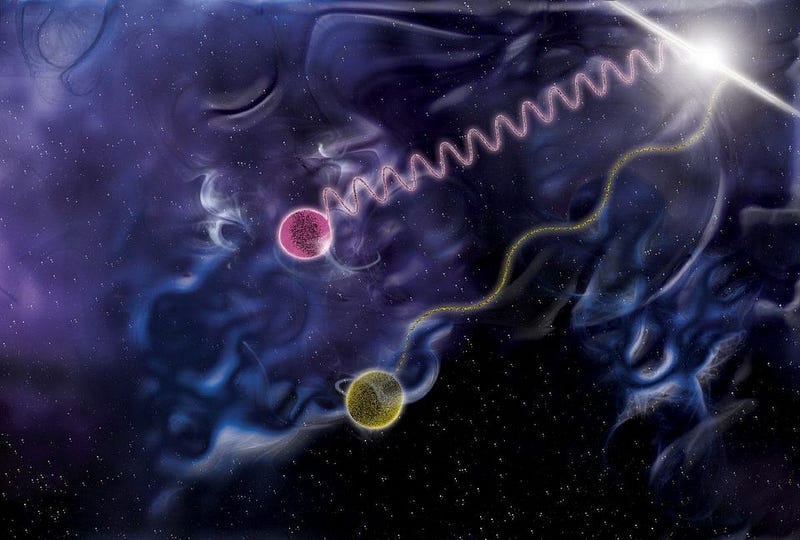
This tells us another important piece of the puzzle: whatever time delays take place for photons as they travel through the Universe owing to the curvature of space also occur for gravitational waves. Whenever you enter or leave an area where gravitation is strong, you have to follow the path set forth by the curvature of space. Around a massive galaxy, for example, like the one we observed the kilonova in, space is curved, and all massless particles have to climb out of that potential well.
The fact that photons and gravitational waves arrived simultaneously tell us that they had to experience the same effects as one another from the curved space they passed through.
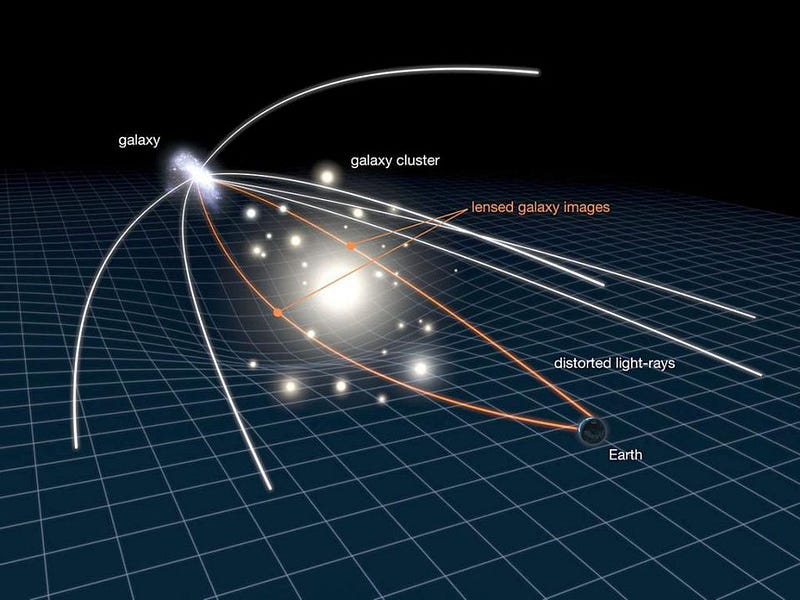
So gravitational waves, observationally:
- experience the stretching effects of the expansion of the Universe,
- follow the same paths as photons do (to the best of our ability to detect it),
- suffer the same time dilation and time delay effects as other massless particles,
- and experience the same changes in energy as they move into and out of regions of severe gravitational curvature.
This carries with it an implication that’s quite profound, although it might not be intuitive. At some level, we fully expect that there is a quantum theory of gravity governing the Universe, and that the graviton is the particle responsible for the gravitational interaction.
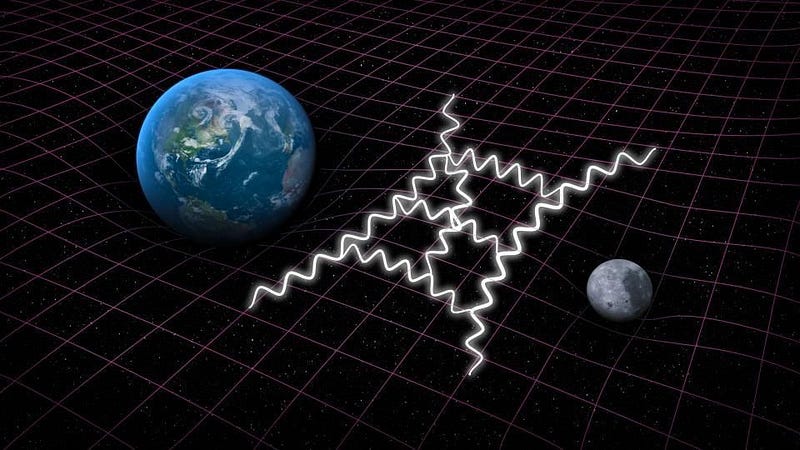
If gravitational waves experience gravity, that means that gravitons don’t just interact with the energy-carrying particles of the Standard Model, but there is a graviton-graviton interaction as well.
Two different gravitational waves, in Einstein’s relativity, should interfere when they meet. But they can’t simply pass right through one another; General Relativity itself is a nonlinear theory, meaning that the gravitational waves must interact and scatter off of each other at some level. This tells us there’s a subtle application to quantum gravity: there’s a chance of having a graviton-graviton scattering interaction.
Gravitons, the particles responsible for the gravitational force, don’t only mediate interactions between the particles of the Standard Model. There’s a chance that they can collide with one another, and what possibly happens when they do is a puzzle that only quantum gravity will be able to solve.
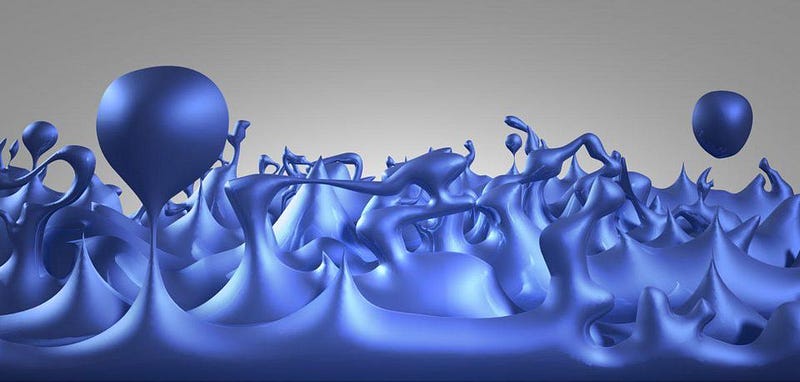
Although it might seem counterintuitive that gravitation would affect gravitational waves, this is one of those wonderful times where theory and observation line up perfectly. They demonstrate that gravitational waves must follow the curved paths set by the presence of mass and energy in the Universe; that they see their wavelengths stretch as the Universe expands; that they obey the rules of time dilation; that they follow the same paths that photons do, minus the interactions with matter.
This realization also carries with it some consequences for a quantum theory of gravity, which may constrain or even rule out some possible scenarios that would otherwise be incredibly interesting. In our quest to understand the Universe, gravitational wave astronomy truly is taking us to the next frontier!
Send in your Ask Ethan questions to startswithabang at gmail dot com!
Ethan Siegel is the author of Beyond the Galaxy and Treknology. You can pre-order his third book, currently in development: the Encyclopaedia Cosmologica.