Ask Ethan #48: Where does cosmic rotation come from?
From atoms to solar systems to galaxies, everything seems to have rotation and revolution. Where does that come from?
Image credit: Fermilab / DOE / Dark Energy camera; Dark Energy Survey.
“In my better sense of mind, I know that I’m far from alone and far from the worst, and the earth keeps spinning. Everything keeps moving, with or without me.” –Phil Anselmo
We’ve finally reached the end of an extremely busy, information-packed week here on Starts With A Bang, and yet you all somehow found time to continue to submit your questions and suggestions for our weekly Ask Ethan column. This week’s selection comes from a relatively new reader, Eric, who wants to know the following:
So, here is a question I’ve wondered about since I was a boy (I’m 44 now). All around us, from the micro to the macro, we see things revolving around other things: electrons around nuclei, moons around planets, planets around stars, stars around galaxy cores (I think). Do galaxies revolve, or orbit, around something? If so, can you speculate as to what it may be? After reading Ask Ethan #45, I now wonder if universes also revolve around something. Is there any way to know?
This is actually multiple questions in one, so let’s start at the beginning: the very beginning!
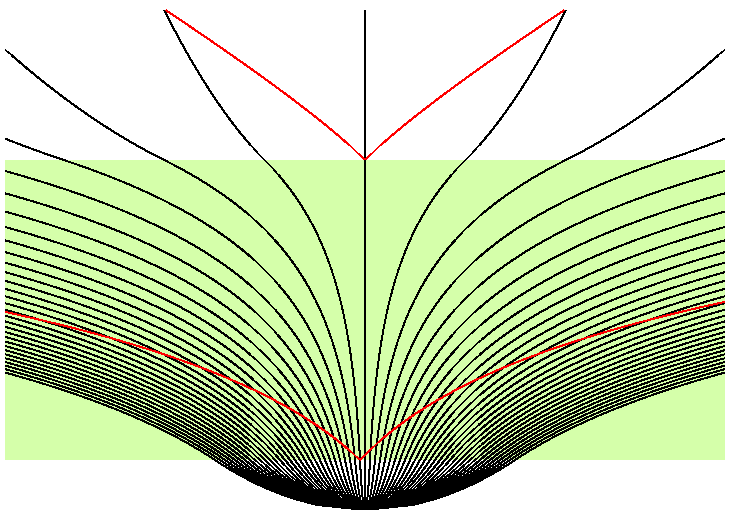
Before our Universe was filled with matter, radiation, neutrinos, dark matter or any of the particles that we currently find in it, it was in a rapidly expanding state, where the only energy found in our spacetime was the energy intrinsic to space itself. This was the period of cosmic inflation that gave rise to the Big Bang that we identify with the birth of what we call our Universe. During this time, as far as we can tell, there were quantum fluctuations produced, but they couldn’t interact with one another, as the expansion of space was too rapid to permit interactions mediated “only” at the speed-of-light. As far as we can tell, the expansion was the same everywhere and in all directions, with no particular preferred axis of any type.
But when inflation ended, that intrinsic-to-space energy was converted into matter, antimatter and radiation, and those quantum fluctuations gave rise to overdense and underdense regions in a rapidly expanding Universe.
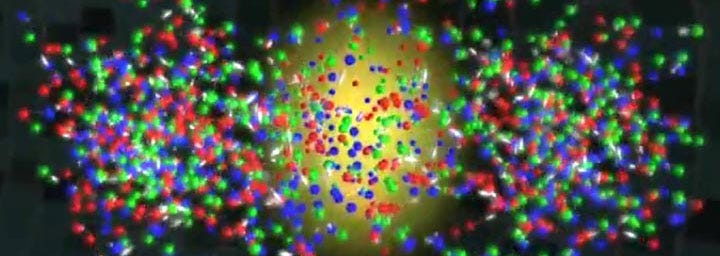
This is what we identify as the Big Bang. From the outset, all of the fundamental particles are born with an intrinsic angular momentum: a property known as spin that cannot be separated from the particle itself. Every electron, quark and neutrino has a spin of either ±½, while every gluon or photon has a spin of ±1. Gravitons, assuming that gravity is quantized the way we believe it to be, has a spin of ±2; only the Higgs Boson, out of all the fundamental particles, has a spin that’s intrinsically zero.
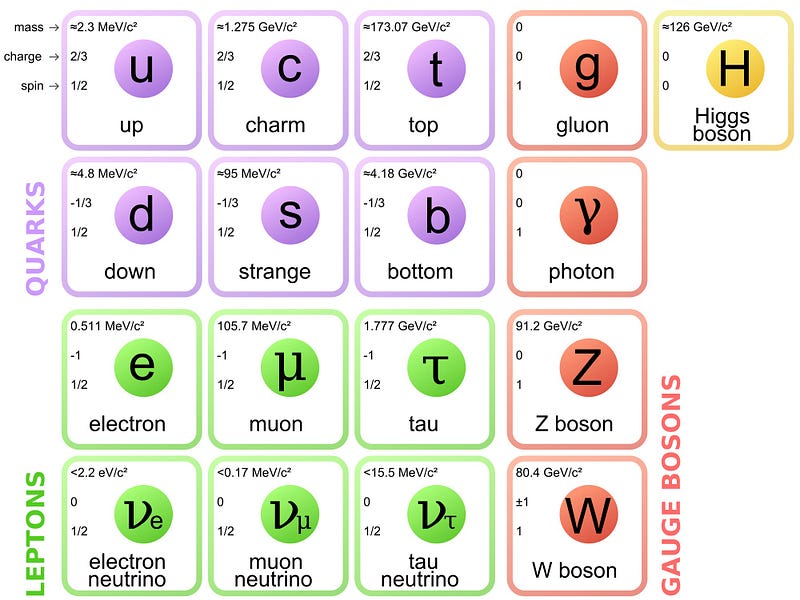
When these particles are first created, they have not yet had the opportunity to interact with one another. It’s fair to say that to the best of our understanding, the Universe isn’t born with any one particle orbiting any other. But particles are born with intrinsic kinetic energies and in locations with variable densities. As they collide and gravitationally interact, the overdense regions gravitationally attract more and more matter-and-energy, while the underdense regions grow even sparser, giving up their matter-and-energy to the relatively denser regions nearby.
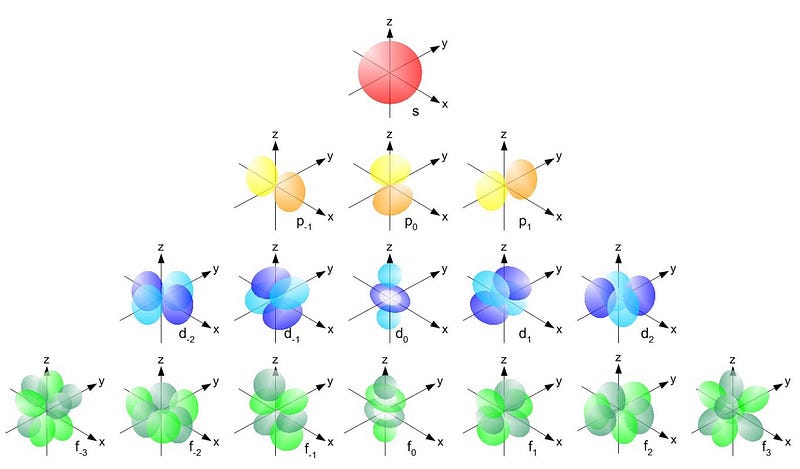
As the Universe cools, quarks condense into atomic nuclei, which have their own intrinsic angular momenta as governed by the laws of nuclear and particle physics. Similarly, when the Universe cools enough that neutral atoms can form, it isn’t quite a “planetary orbit” model like you might think of a la the Bohr atom, but rather occupy specific quantum states, all with their own intrinsic spin and orbital angular momenta, as illustrated above.
By time the Universe forms these neutral atoms, the gravitational differences between an underdense and an overdense region have been magnified many times over from what the Universe was born with.
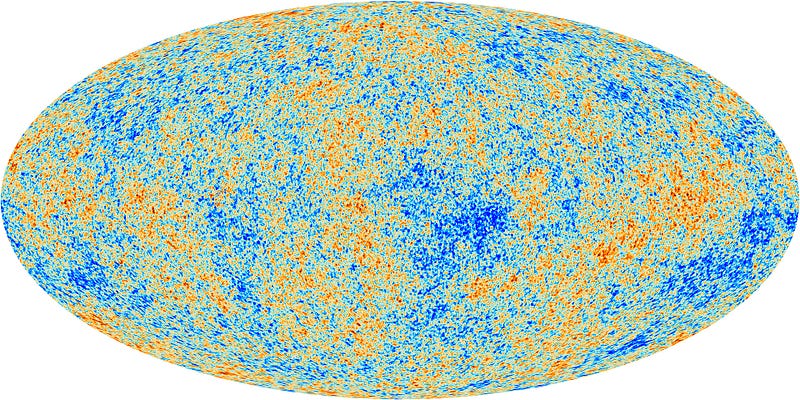
Even when the Universe is very young, we’ve got gravitationally unique regions — regions that will grow into stars, galaxies, clusters and more — that move relative to one another and exert a gravitational force on each other. Unless two of these gravitational sources have the extraordinarily unlikely properties of both being perfectly spherical and also moving with a velocity that’s only along the imaginary line connecting them, they’re going to exert a very special type of force on one another: a tidal torque.
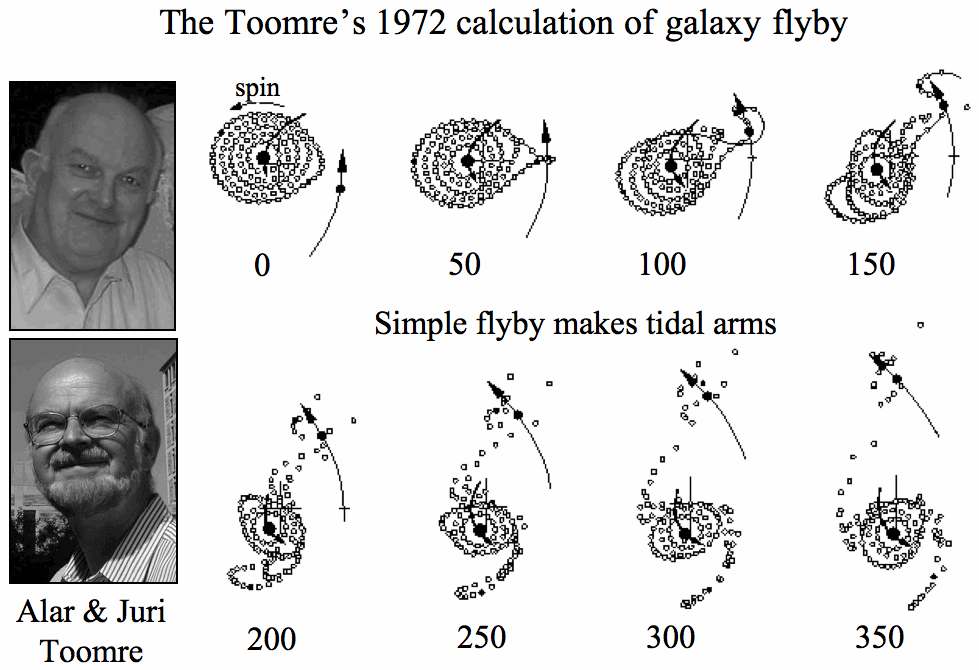
Every bit of matter-and-energy that moves relatively non-aligned to any other bit of matter-and-energy causes a gravitational interaction that creates a torque, the same way that pushing up-or-down on a wrench causes a nut to turn.
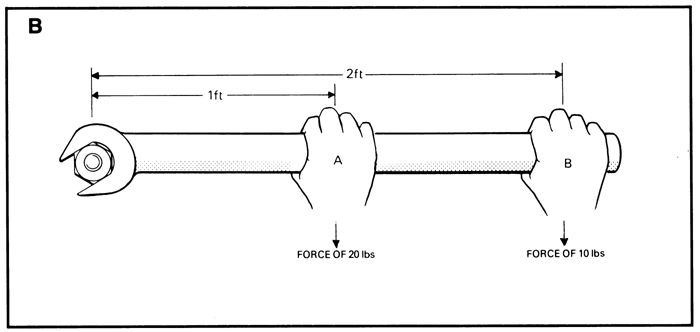
These torques occur on scales both large-and-small, and affect every bit of matter we know of, all the way down to individual atoms interacting with one another. As time goes on and gravitational collapse begins to happen, these small amounts of angular momentum — 50% of which ought to be clockwise and 50% of which should be counterclockwise — are enough to cause these huge, massive collections to rotate very slowly.
But certain things in physics are special because they’re conserved quantities. You’re probably familiar with the conservation of energy: the statement that energy cannot be created or destroyed. A little less well known is the conservation of momentum, which also cannot be created or destroyed. But what most people don’t realize is that angular momentum is also one of these quantities. You can see this applied, however, if you observe a spinning figure skater pull his-or-her arms and legs in close to their body.
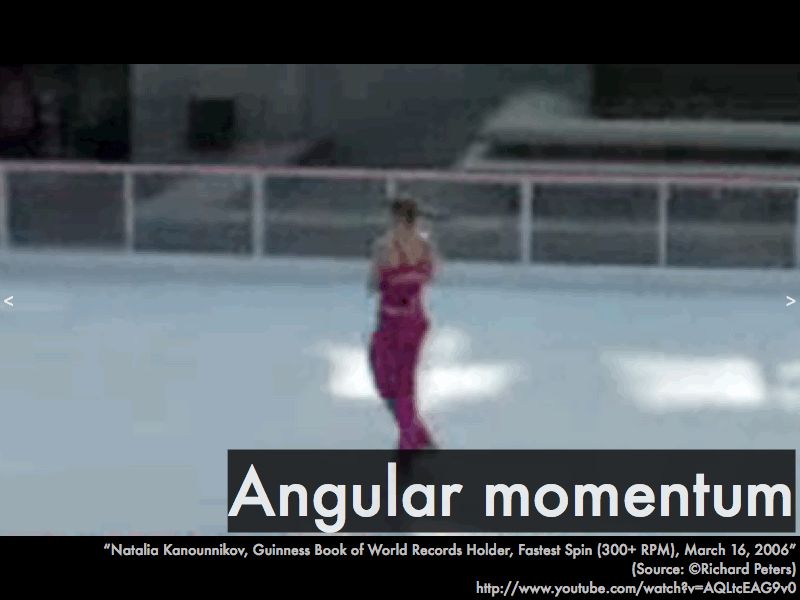
By changing what’s known as their moment of inertia (bringing their mass distribution closer to their axis-of-rotation), the conservation of angular momentum dictates that their angular velocity (or rotational speed) must increase in order to compensate. Our Sun, for example, rotates with a period of a little under one month. If, however, we were to collapse it down to a white dwarf — an object about the size of the Earth — its angular velocity would have to increase so substantially that it would rotate once every thirty-six minutes!
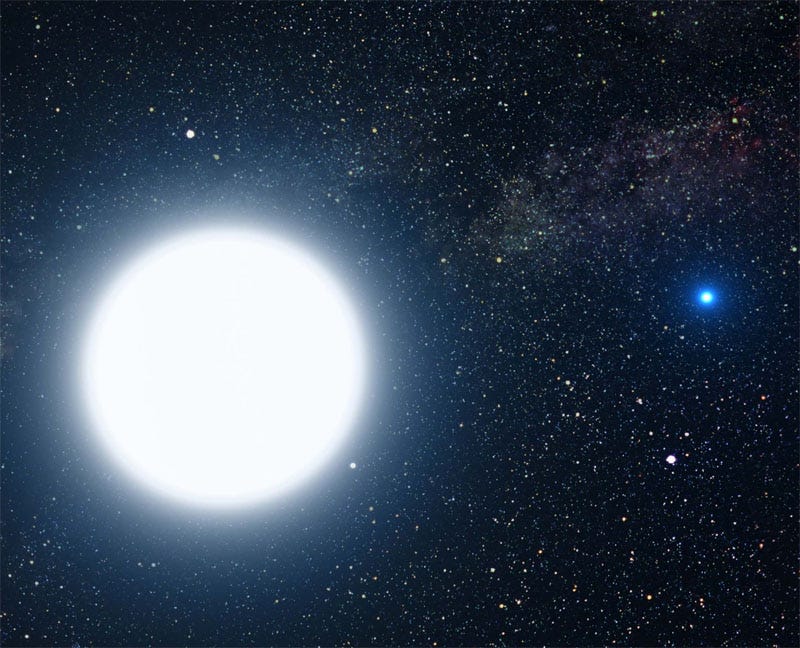
When it comes to star systems, individual planets-and-moons, or galaxies as a whole, the fact that we see more than a single dense, stationary object is evidence that every known system in the Universe has experienced these tidal interactions, and has a non-zero amount of angular momentum with respect to the other objects in the Universe.
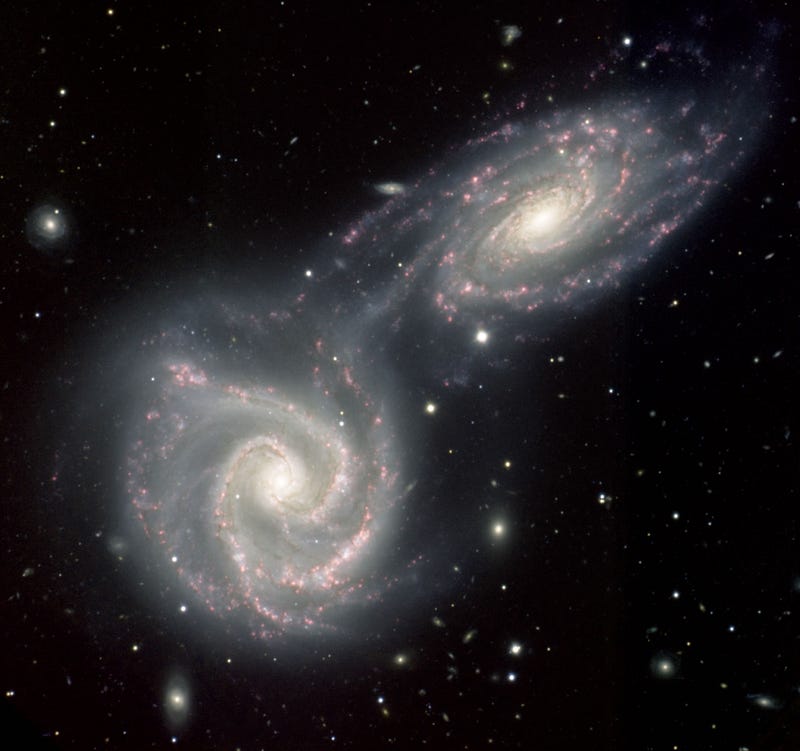
In other words, even though there often is a black hole at the center of galaxies, its presence is not at all what’s responsible for the galaxy’s rotation: the galaxy would continue to rotate and the stars would continue to revolve around it even in the total absence of one! In fact, we see many spiral galaxies without discernible central black holes at all, and they do just fine.
Gravitation, the inevitable physics of torques, and the conservation of angular momentum are why everything rotates.
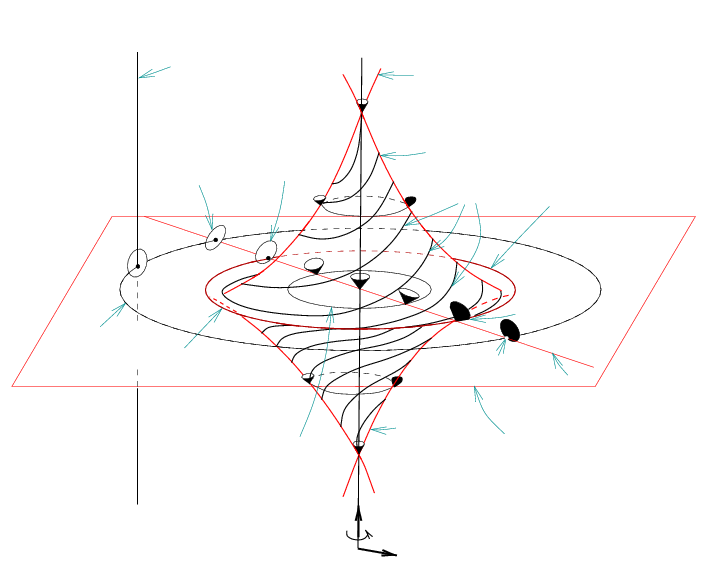
On the other hand, what if we consider the entire Universe as a whole? We think that the Universe doesn’t have any overall rotation, because gravitation hasn’t had (and will never have) the opportunity to interact on scales larger than our observable Universe is today, but all we have are constraints at this point. The Universe could have, in principle, a certain amount of angular momentum that it was born with overall, and that would give us an even greater mystery to dig into!
Thanks for a great question, Eric, and if you’d like to submit your questions and suggestions to have a shot at being the next Ask Ethan, send them in. Until next week, have a great one!
Leave your comments at the Starts With A Bang forum on Scienceblogs!