Ask Ethan #46: What is a Quantum Observation?
The observer changes everything, but what does that mean?
“You can observe a lot by just watching.” –Yogi Berra
In our everyday lives, things happen the way they happen, and whether we watch or not doesn’t appear to make a difference one way or the other. Sure, we may feel anxiety if others are watching us, but that has more to do with our internal state-of-mind than any intrinsic physical difference. But the quantum world is different! You kept sending in your questions and suggestions for Ask Ethan, and the one I chose this week came from Robert Coolman, who asks:
What is “observation”? [Two] examples the more I think about, the less I understand: Young’s Experiment and Bell’s Theorem. The more I think about it, the more I realize I have no idea what “observation” actually is. Can you help me understand this?
Let’s start by giving you these two classic examples of quantum weirdness.
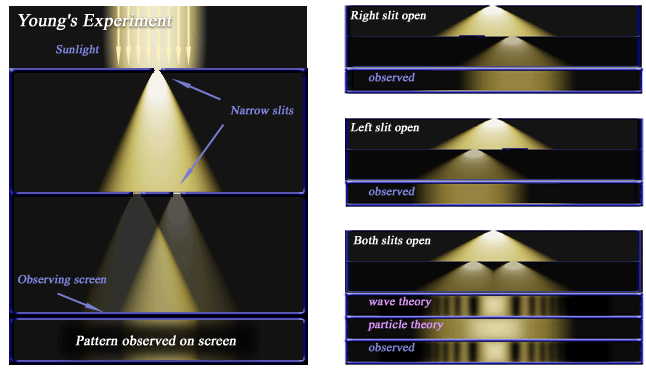
First off, there’s Young’s Experiment. It was known for a long time that individual particles — things like pebbles, if you like — behave differently from waves, such as water. If you have a screen with two slits in it and you throw a handful of pebbles (or bullets, or other macroscopic particles) at them, most of the pebbles will be blocked by the screen. But where the slits are, a few of the pebbles will get through. What you’d expect, and in fact what happens, is that you’ll get a bunch of pebbles that go through the slit on the left and a bunch more that go through the slit on the right.
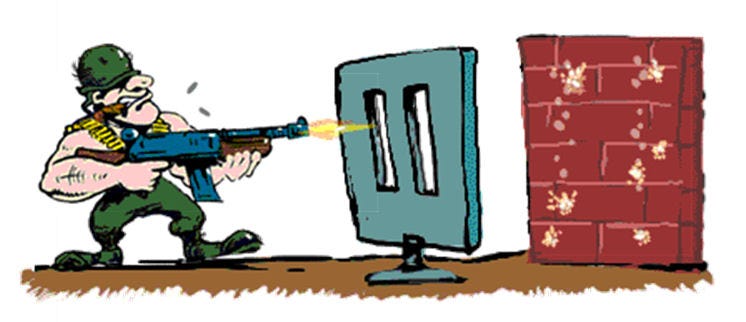
You’d get two piles of pebbles that make roughly a bell curve, each pile corresponding to one of the two slits. And as you’d expect, this happens whether you look at the pebbles as you’re throwing them or not. You throw the pebbles, you get this pattern: done deal.
On the other hand, what if you had a tank of water and were capable of making waves on one end? You could place a screen with two slits in it, so that only those two slits allow water waves through. This creates two sources for the waves — or ripples — to emanate and propagate from.
And, as you’d expect, you’ll get an interference pattern at the other end, with peaks (high points) and troughs (low points), as well as points in between where you simply get the average water height with no ripples at all. This is the wave phenomenon of interference, which can be constructive where the peaks-and-troughs add together, and destructive where the peak of one source and the trough from the other cancel each other out.
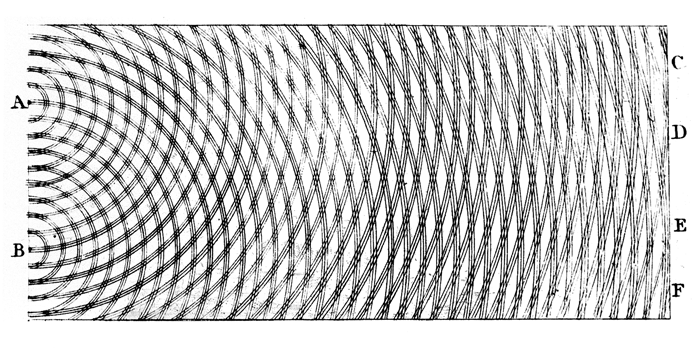
Young’s experiment, in its original incarnation, was performed way back in a series of experiments between 1799 and 1801, and shone light through two slits in an effort to determine whether it behaved like a particle or a wave. This is now a standard experiment that undergraduates perform in their introductory physics labs, and if you perform it yourself, you’d see patterns like this:
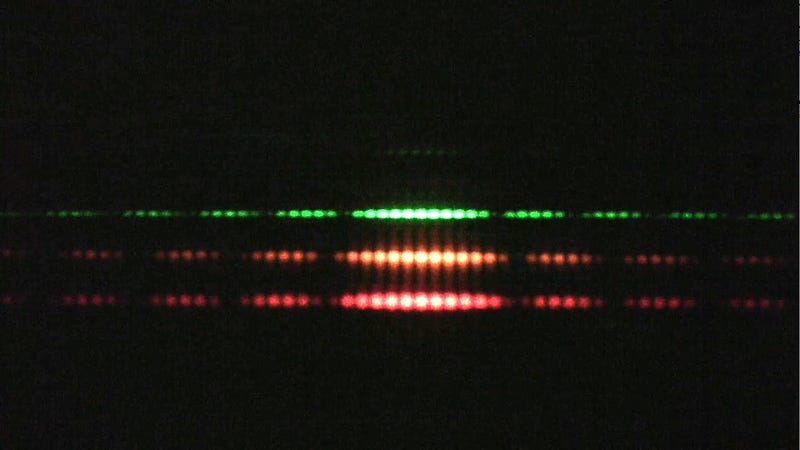
Clearly, there’s interference happening. Well, in the early 1900s, the discovery of the photoelectric effect — consistent with the idea that light was quantized into photons of distinct energies — seemed to indicate that light was a particle, not a wave, and yet it definitely made this wave-like interference pattern when shone through a double slit.
Well, things were about to get much weirder. In the 1920s, physicists had the bright idea to perform this same experiment, except using electrons instead of photons. What would happen when you fired a stream of electrons (something you could obtain by taking a radioactive source that underwent β decay) at a double slit, with a screen behind it? What type of pattern would you see?
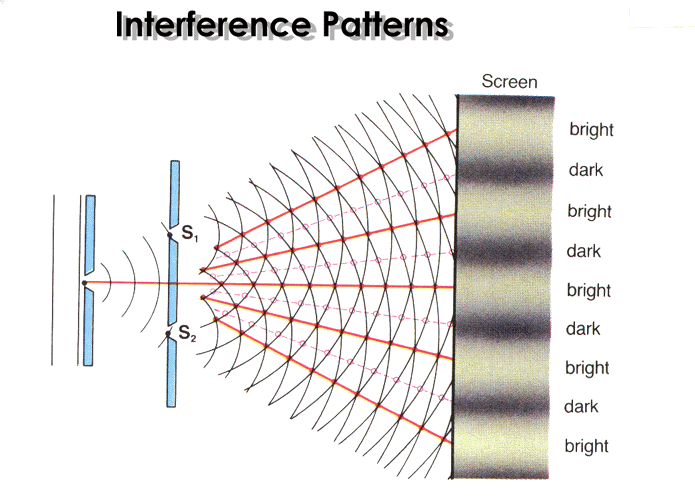
Bizarrely enough, a source of electrons gave you an interference pattern!
“Okay, wait a minute,” said everyone. “Somehow, these electrons must be interfering with the other electrons from the radioactive decays. So let’s send them through one-at-a-time, and have a look at what appears on the screen.”
So they did that experiment, and kept an eye out for what the pattern would look like after each and every electron that passed through. This is what they saw.
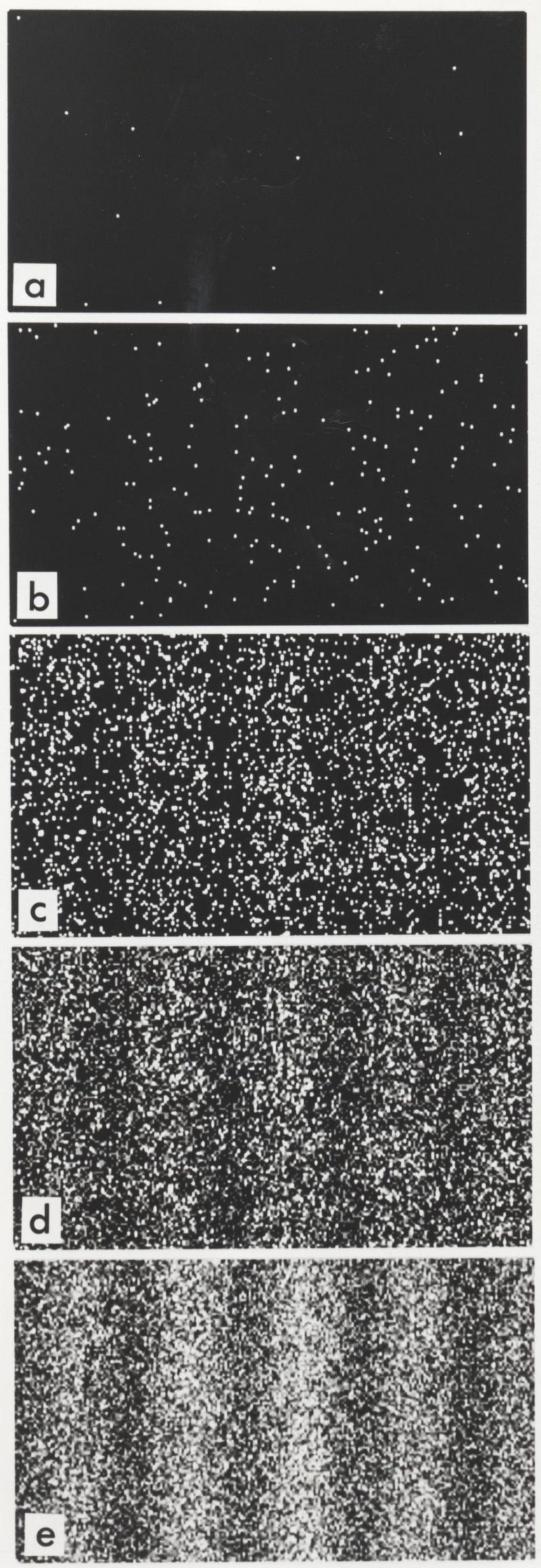
Somehow, each electron was interfering with itself as it passed through the slits! So this led physicists to the question of how this was happening; after all, if electrons are particles, they should be passing through one slit or the other, just like pebbles or bullets.
So which one was it? They set up a “gate” (where you shine photons to interact with whatever passes through the slit) to find out which slit each electron passed through, and found, sure enough, that it was always one slit or the other. But when they looked at the pattern that emerged, they found the particle pattern, not the wave pattern. In other words, it looked like the electron somehow knows whether you’re looking at it or not!
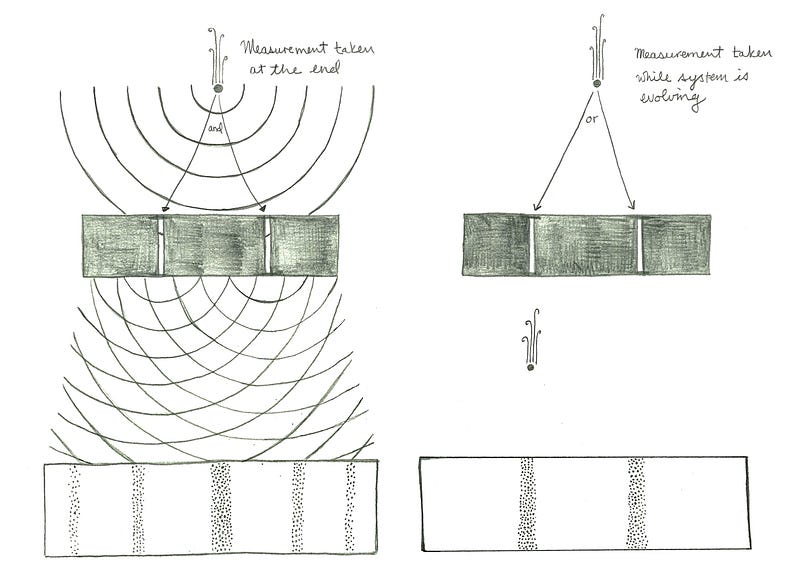
Or, as physicists sometimes frame it, the act of observation changes the outcome. This might seem peculiar, but this is actually what happens in pretty much all quantum systems set up like this: things evolve like they’re in a wave-like superposition of all possible outcomes until you make the key “observation,” which forces the system to give you one real answer.
The other example Robert refers to is quantum entanglement.
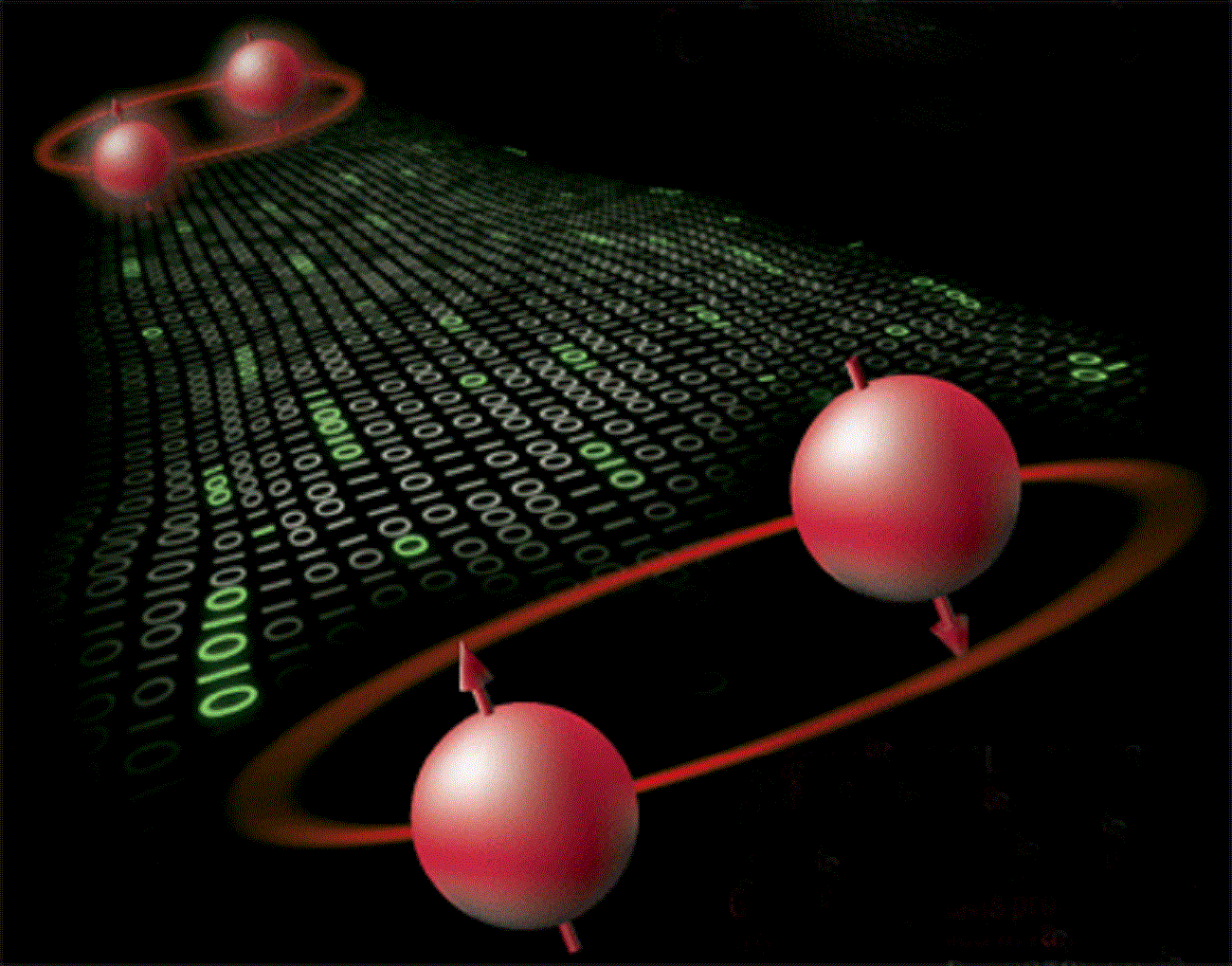
Many particles can be created in an entangled state: where you know that, for example, one needs to have a positive spin and one needs a negative spin (e.g., ±½ for electrons, ±1 for photons, etc.), but you don’t know which is which. In fact, until you make a measurement, you have to treat each particle like it’s a superposition of the positive state and the negative state. But once you “observe” the property of one of them, you immediately know the corresponding property of the other.
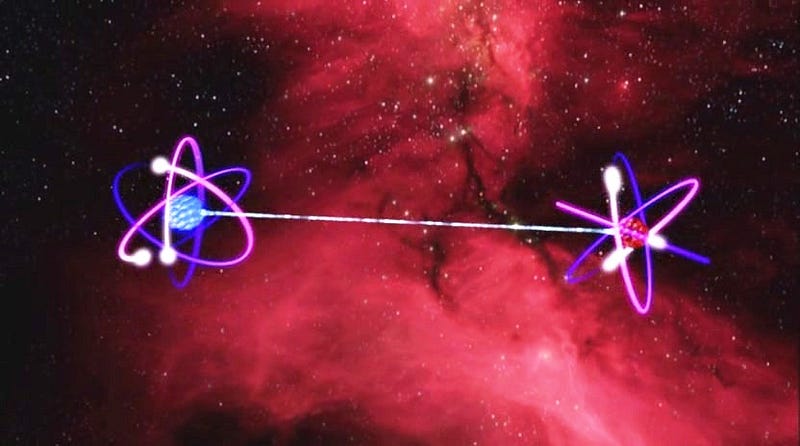
This is weird, because just like the electron passing through the slit, particles behave differently when they’re in a superposition of states versus when they’re forced to be in one “pure” state. You can, in theory, entangle two particles here, move the other one a light-year away, “observe” the first one (and immediately know its spin), and you will immediately know the spin of the other one; you won’t have to wait a year for the speed-of-light to transmit that signal.
Now, if that sounds spooky to you, that’s because it is. No less a person than Einstein was troubled by it, and the resolution (by Bell, which is why it’s called Bell’s theorem) is that quantum entanglement is what we call a non-local phenomenon.
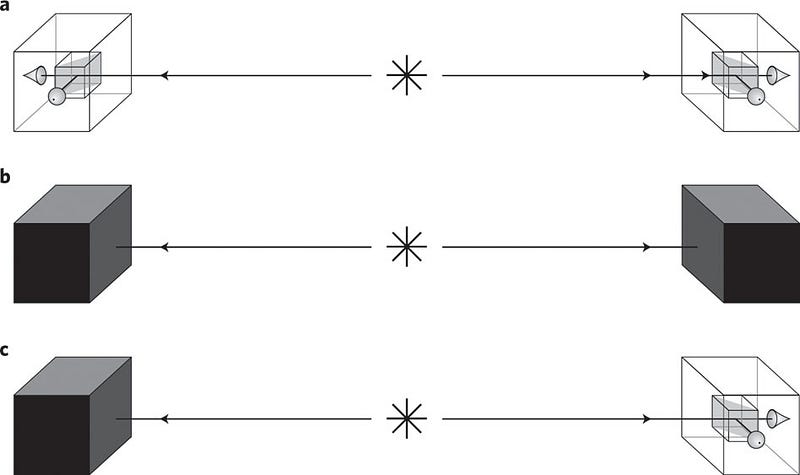
To be fair, the person with the particle a light-year away won’t notice anything weird about their particle once you measure yours; it’s only once you bring your particle together with theirs (or the information from it, both of which are limited by the speed of light) can you observe the states of both particles.
So, after all that, we’re ready for the meat of Robert’s question: what is an observation?
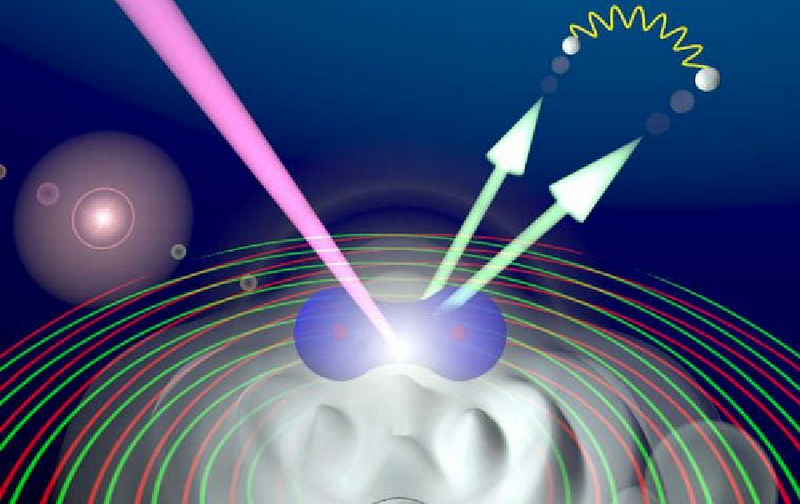
Contrary to what you may believe based on everything you just read, it has nothing to do with you, the observer. All this talk about measuring and observing has hidden the real truth here: in order to make these observations, we’ve needed to make a quantum particle interact with the particle we’re trying to observe. And if we want to make these particular measurements, we need that interaction to take place above a certain energy threshold!
It has nothing to do with you or the “act of observing,” and instead everything to do with whether you interact with sufficient energy to “make an observation,” or — in non-anthropomorphized terms — to constrain the particle into one particular quantum state or another.
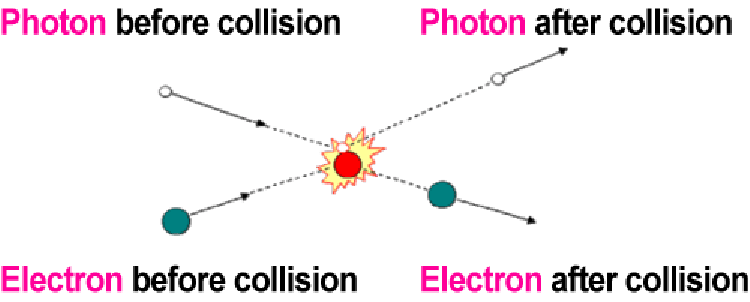
For an electron passing through a slit, that means forcing an interaction with a photon that can constrain its position well-enough to be definitively through one slit. For a photon of either spin +1 or -1, that means making a measurement sensitive to its polarization, which means having an interaction that is sensitive to the type of electromagnetic field the photon creates.
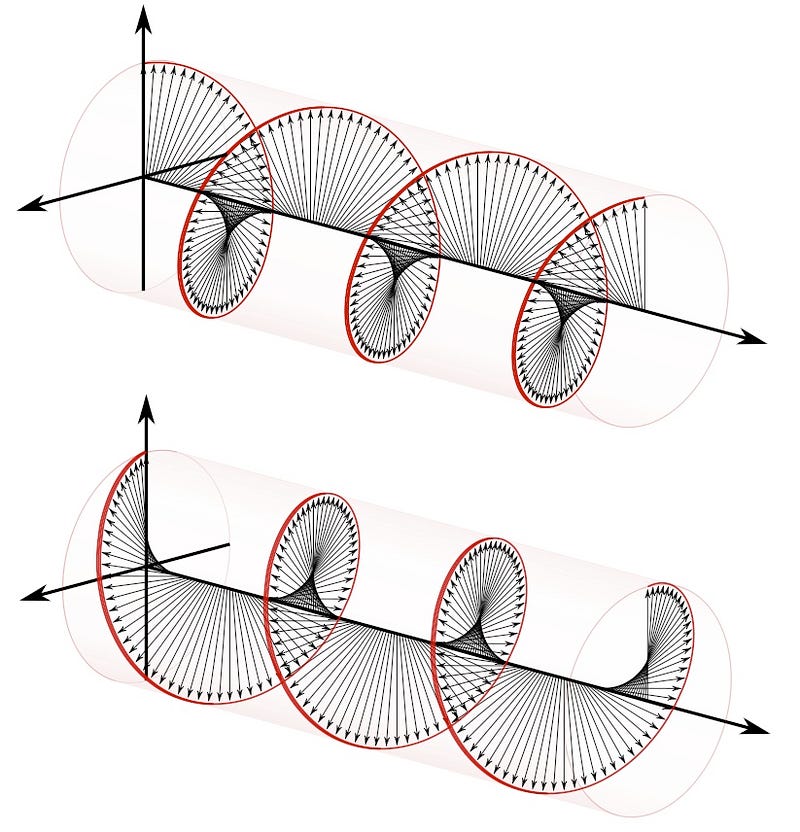
So if you want the TL;DR version: an observation is a quantum interaction that is sufficient to determine the quantum state of a system.
But what a different Universe the quantum one is from our own, macroscopic experience! I hope you enjoyed it, and if you have a question or suggestion for the next Ask Ethan column, don’t be afraid to ask. The next one could be yours!
Leave your comments at the Starts With A Bang forum on Scienceblogs!