Ask Ethan #35: Is there a limit to Lasers?
Or, in theory, can they produce an infinite amount of energy?
“The atoms become like a moth, seeking out the region of higher laser intensity.” –Steven Chu
Each week on our Ask Ethan series, we take one lucky question or suggestion from a reader just like you and look at the science behind whatever’s being asked. The topics we’ve taken on have ranged from theoretical physics to geophysics, from black holes to the expanding Universe, and from education to airplanes. This week’s question, however, dives into an arena we haven’t encountered in a long time: lasers! Let’s take a look at what our reader Murtaza had to say:
I asked this from my optics professor in University 5 years ago, but didn’t get any answer. We were studying lasers and the lasing cavity. My question was how many photons can be pumped into such a cavity? Is there a limit to the density of the photons? What happens when this limit is crossed?
As always, let’s start at the very beginning: an atom.
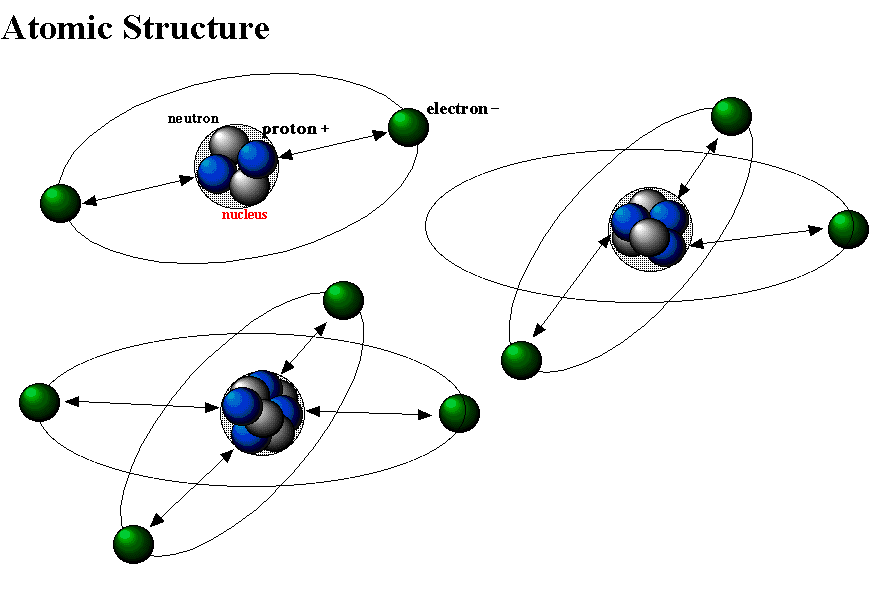
You might be familiar with an atom as a positively charged nucleus and a number of electrons orbiting it; simple as it is, this is a pretty good picture. These electrons typically exist in a number of finite configurations, only one of which is optimally the most stable: the ground state.
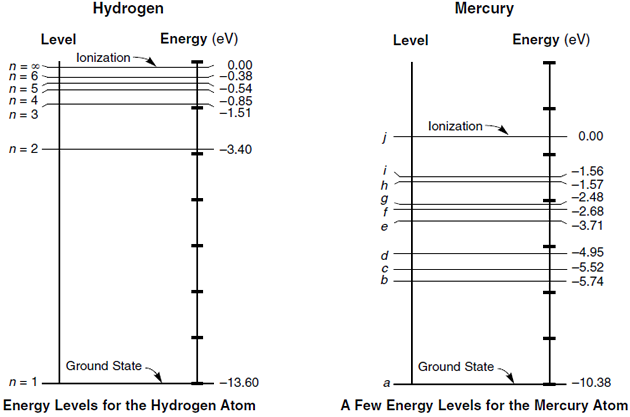
When you stimulate (i.e., add energy to) the atom in just the right way, its electron configuration can change, and it can enter a higher energy configuration: an excited state. All things being equal, that excited state will spontaneously decay to a lower energy state — either all-at-once to the ground state or in a chain — after a finite amount of time, emitting a photon of a very particular energy (or energies) when it does so.
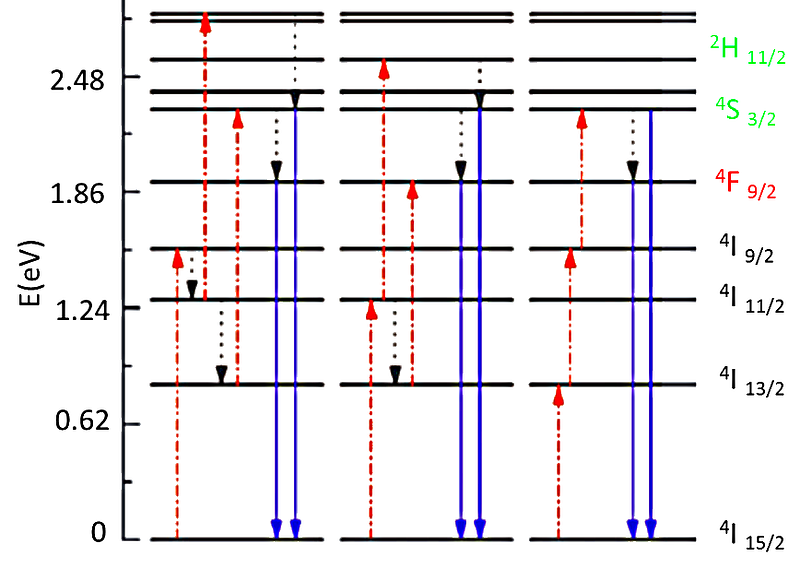
Now, that’s how it works for a single, free atom. Most of what exists in nature — at least on this world — isn’t a single atom, but consists of many atoms bound together in particular ways: the diversity of molecular compounds, crystals and gaseous configurations is simply mind-boggling. (Although it is finite!)
But each one still has a given number of electrons and energy states that they can occupy. If you can add energy to the system and excite one (or more) of the electrons, you can often coax it to emit radiation of a particular frequency. And if you stimulate your system in the correct, controlled fashion, you can get it to emit radiation of a uniform wavelength, frequency and direction pretty much every time. That’s what a LASER is.
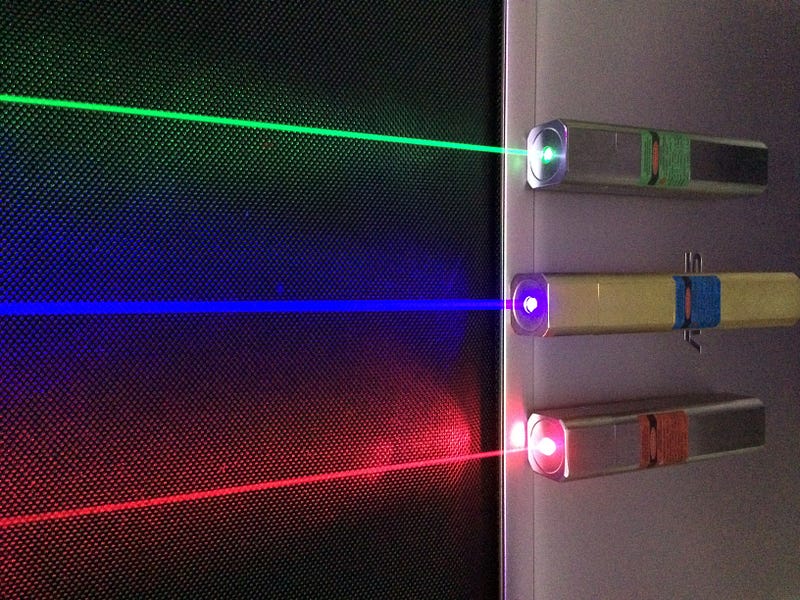
Technically, LASER is an acronym, standing for Light Amplification by Stimulated Emission of Radiation, although in truth, nothing is really being amplified. Instead, the electrons oscillate between either the excited-and-ground state or two different excited states, but for some unknown reason, no one wanted the acronym Light Oscillation by Stimulated Emission of Radiation. (I wonder why!)
The “spontaneous emission” part, however, is of paramount importance, and what makes a laser lase.
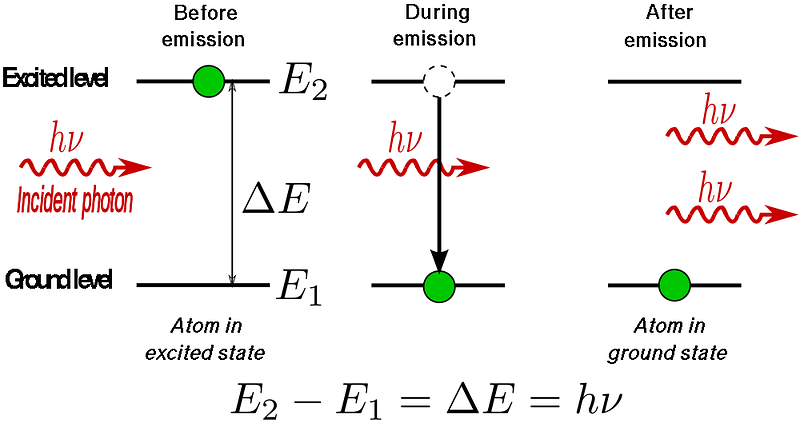
If you can produce either multiple atoms-or-molecules in the same excited state and stimulate their spontaneous jump to the ground state, they’ll emit the same energy photon. These transitions are extremely fast (but aren’t infinitely fast), and so there is a theoretical limit to how quickly you can make a single atom (or molecule) hop up to the excited state and spontaneously emit a photon; the system takes time to reset.
Normally, some type of gas, molecular compound or crystal is used inside a resonant-or-reflective cavity to create a laser, but those are not the only ways!
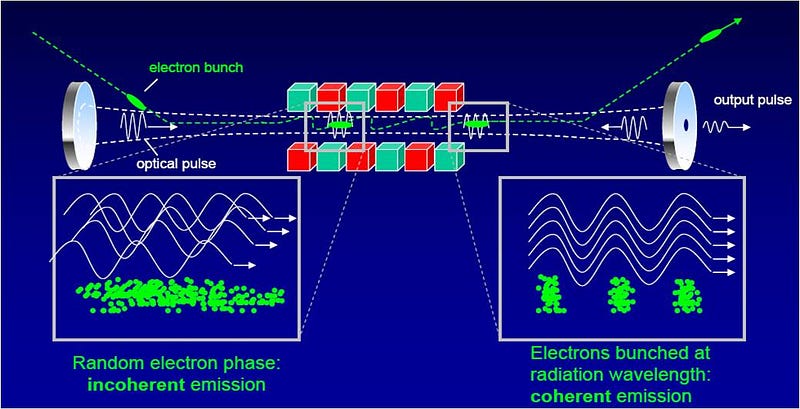
Free electrons can also be used to make lasers, as can semiconductors, optical fibers, and possibly even positronium: bound states of electrons and positrons. The wavelength that lasers can emit in range from extremely long radio waves to incredibly short X-rays, with gamma rays theoretically possible as well. We’ve even noticed this the laser process occurring naturally in space! Mostly occurring in coherently-moving clouds at microwave frequencies, some of these are actually energetic enough to become true visible-light lasers!
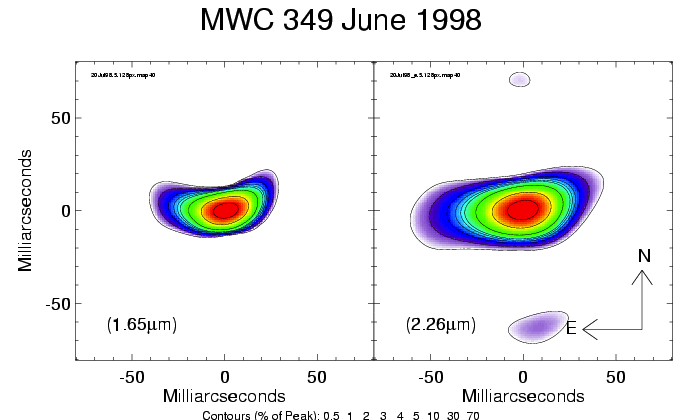
As new methods and techniques are developed, the amount of energy lasers produce has continued to rise over time, with intensities limited only by the practicalities of modern technology. You might wonder if there’s an intrinsic limit to the number of photons that could exist due to a laser (or laser-like process), since there is a limit to, say, the number of electrons you can cram into a given region of space.
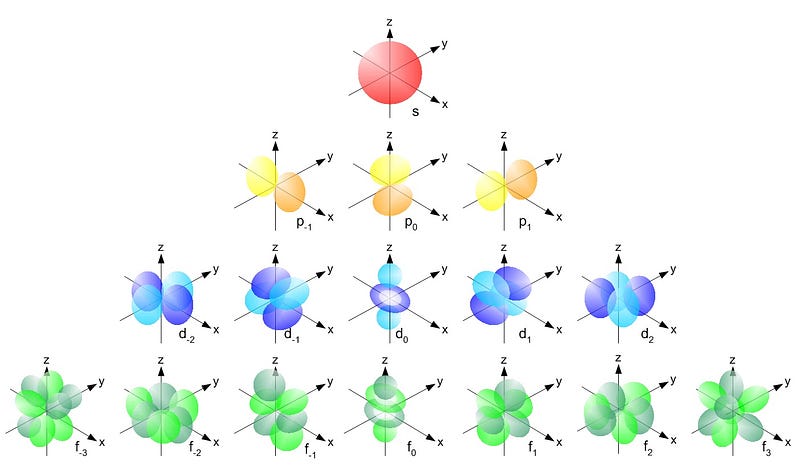
You see, in quantum mechanics, there’s a very important principle — the Pauli exclusion principle — that declares that no two quantum particles with exactly identical properties can exist in the same quantum state simultaneously. Only, I just lied to you; the Pauli exclusion principle only applied to particles like electrons or quarks, whose spin comes in half-integer increments: ±1/2, ±3/2, ±5/2, etc. For particles with integer spins: 0, ±1, ±2, etc., there is absolutely no limit to the number of identical particles that can occupy the same state!
At a fundamental level, this is why what we consider “normal matter” takes up space at all. But not everything is bound by that rule.
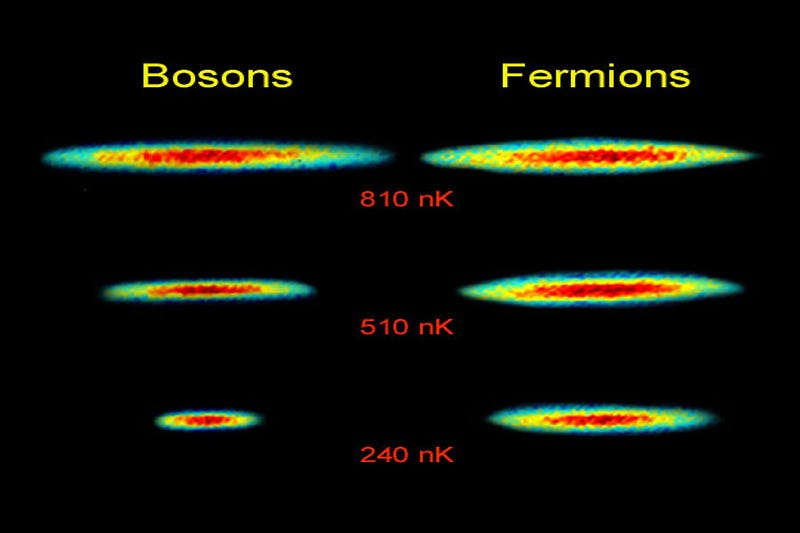
The photon, which is the particle produced by lasers of all varieties, has a spin of ±1, and hence you can theoretically pack an arbitrarily large number of them into as small a space as you want.
This is theoretically extremely important, because it means that, if we can figure out the right technology, there’s no limit to the magnitude of energy densities we can achieve!
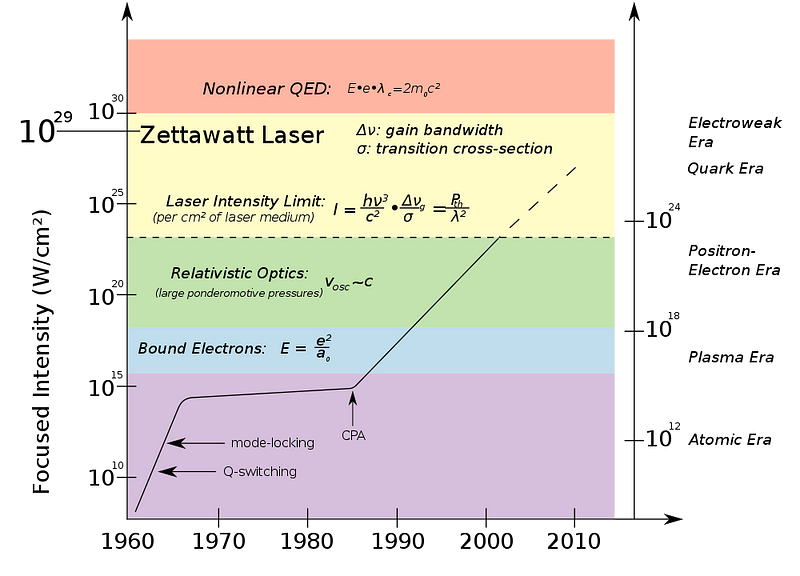
Yes, it’s true that practically, all lasers that operate with a cavity do have a maximum intensity they can reach, but that’s merely a practical limit of the materials used. In fact, if you could take a powerful enough laser and create a large enough mirrored cavity, it ought to be possible to — in theory, of course — make one of those mirrors capable of being slid inwards, evacuate the non-photons inside and compress the reflected light down to a high enough energy density so that it creates a black hole.
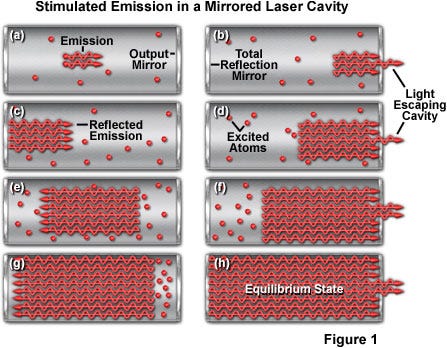
So practically, yes, there’s a limit. But theoretically, that limit is only a function of the materials we use; as we find better and better materials for constructing faster, higher energy and more intense lasers, the energy densities we can achieve continues to rise, with no upper limit in sight.
And that’s the science of lasers: with no limit on our horizon!
Update: After a conversation with Chad Orzel, it looks like although there’s no limit to the photon energy you can produce, you will at some point — above about 1 MeV in photon energy — start spontaneously producing matter-antimatter pairs of particles whenever your photon interacts with a reflective surface. So at extremely high photon energies, your laser light begins to resemble a matter-antimatter thermal bath rather than merely coherent light. So that will turn out to be a limiting factor after all! Sorry to those of you hoping for a black hole someday.
Have a question you’d like to see featured on Ask Ethan? Send yours in here! and if you have a comment, leave it at the Starts With A Bang forum on Scienceblogs.