A Cosmic First: Ultra-High Energy Neutrinos Found, From Blazing Galaxies Across The Universe

In 1987, we detected neutrinos from another galaxy in a supernova. After a 30 year wait, we’ve found something even better.
One of the great mysteries in science is determining not only what’s out there, but what creates the signals we detect here on Earth. For over a century, we’ve known that zipping through the Universe are cosmic rays: high energy particles originating from far beyond our galaxy. While some sources for these particles have been identified, the overwhelming majority of them, including the ones that are most energetic, remain a mystery.
As of today, all of that has changed. The IceCube collaboration, on September 22, 2017, detected an ultra-high-energy neutrino that arrived at the South Pole, and was able to identify its source. When a series of gamma-ray telescopes looked at that same position, they not only saw a signal, they identified a blazar, which happened to be flaring at that very moment. At last, humanity has discovered at least one source that creates these ultra-energetic cosmic particles.
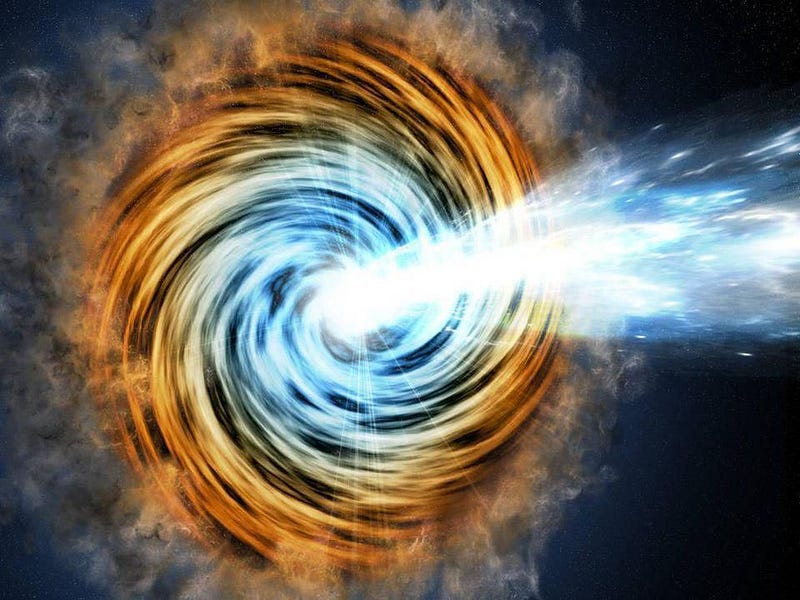
The Universe, everywhere we look, is full of things to look at and interact with. Matter clumps together into galaxies, stars, planets, and even people. Radiation streams through the Universe, covering the entirety of the electromagnetic spectrum. And in every cubic centimeter of space, hundreds of ghostly, tiny-massed particles known as neutrinos can be found.
At least, they could be found, if they interacted with any appreciable frequency with the normal matter we know how to manipulate. Instead, a neutrino would have to pass through a light year of lead to have a 50/50 shot of colliding with a particle in there. For decades after its proposal in 1930, we were unable to detect the neutrino.
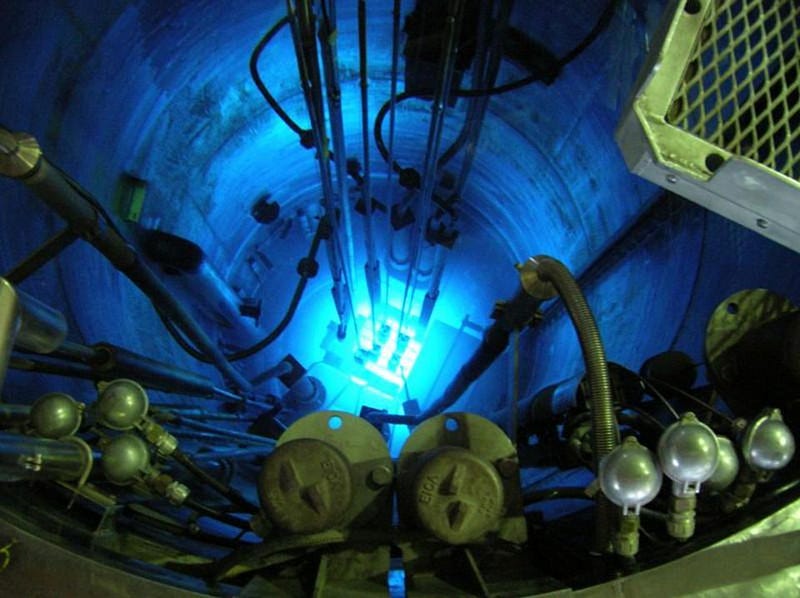
In 1956, we first detected them by setting up detectors right outside of nuclear reactors, mere feet away from where neutrinos are produced. In the 1960s, we built large enough detectors — underground, shielded from other contaminating particles — to find the neutrinos produced by the Sun and by cosmic ray collisions with the atmosphere.
Then, in 1987, it was only serendipity that gave us a supernova so close to home that we could detect neutrinos from it. Experiments running for entirely unrelated purposes detected the neutrinos from SN 1987A, ushering in the era of multi-messenger astronomy. Neutrinos, as far as we could tell, traveled across the Universe at energies indistinguishable from the speed of light.
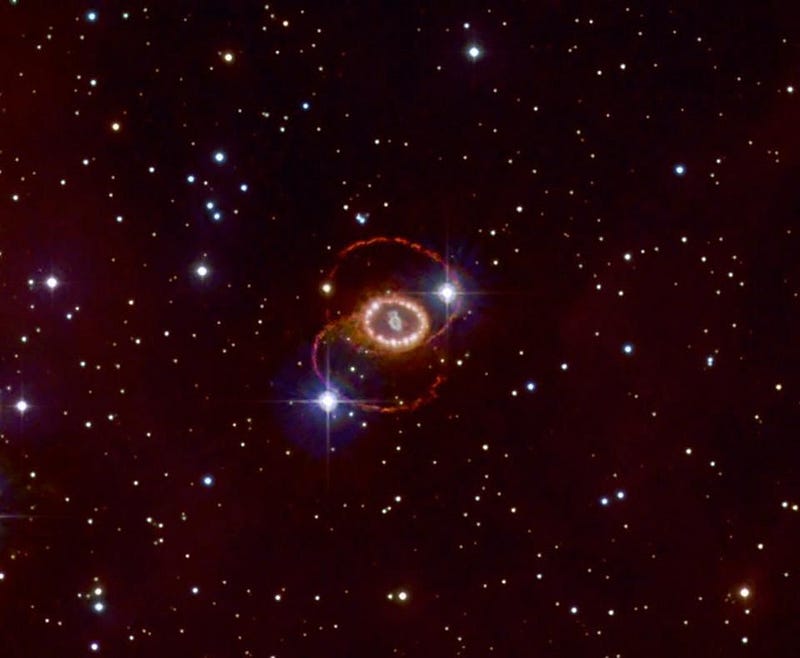
For some 30 years, the neutrinos from that supernova were the only neutrinos that we had ever confirmed to be from outside of our own Solar System, much less our home galaxy. But that doesn’t mean we weren’t receiving more distant neutrinos; it simply meant that we couldn’t robustly identify them with any known source in the sky. Although neutrinos interact only very weakly with matter, they’re more likely to interact if they’re higher in energy.
That’s where the IceCube neutrino observatory comes in.
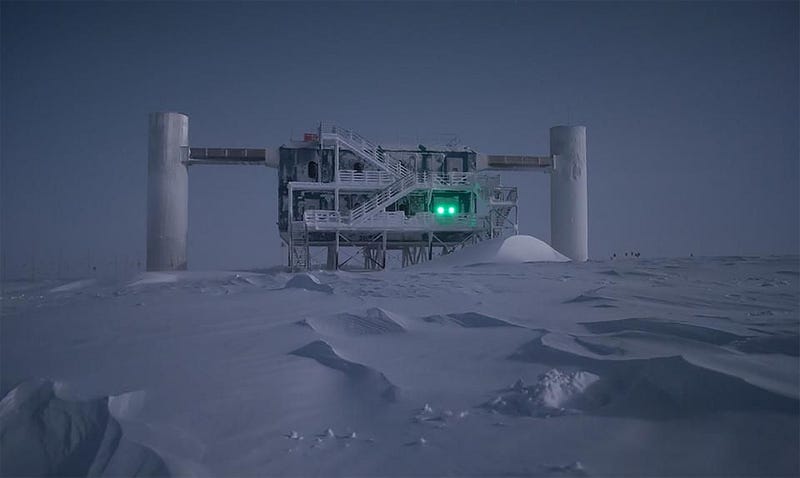
Deep within the South Pole ice, IceCube encloses a cubic kilometer of solid material, searching for these nearly-massless neutrinos. When neutrinos pass through the Earth, there’s a chance of having an interaction with a particle in there. An interaction will lead to a shower of particles, which should leave unmistakable signatures in the detector.
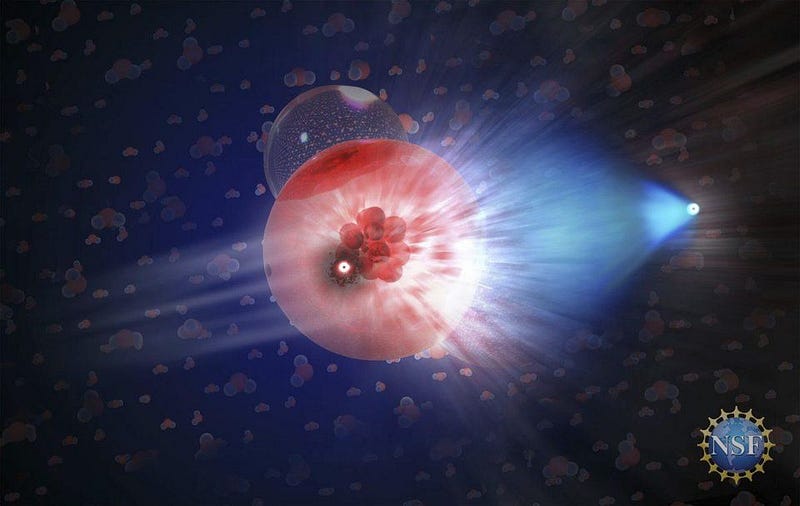
In the six years that IceCube has been running, they’ve detected more than 80 high-energy cosmic neutrinos with energies over 100 TeV: more than ten times the highest energies achieved by any particles at the LHC. Some of them have even crested the PeV scale, achieving energies thousands of times greater than what’s needed to create even the heaviest of the known fundamental particles.
Yet despite all these neutrinos of cosmic origin that have arrived on Earth, we haven’t yet ever matched them up with a source on the sky that offers a definitive location. Detecting these neutrinos is a tremendous feat, but unless we can correlate them with an actual, observed object in the Universe — for example, that’s also observable in some form of electromagnetic light — we have no clue as to what creates them.

Theorists have had no problem coming up with ideas, including:
- hypernovae, the most superluminous of all the supernovae,
- gamma ray bursts,
- flaring black holes,
- or quasars, the largest, active black holes in the Universe.
But it would take evidence to decide.
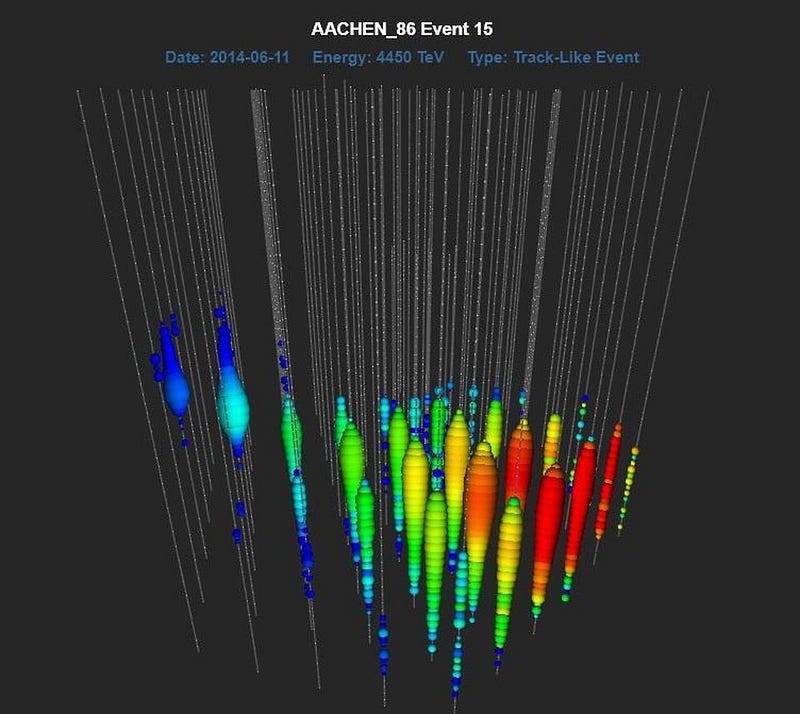
IceCube has been tracking and issuing releases with every ultra-high-energy neutrino they’ve found. On September 22 of 2017, another such event was seen: IceCube-170922A. In the release that went out, they stated the following:
On 22 Sep, 2017 IceCube detected a track-like, very-high-energy event with a high probability of being of astrophysical origin. The event was identified by the Extremely High Energy (EHE) track event selection. The IceCube detector was in a normal operating state. EHE events typically have a neutrino interaction vertex that is outside the detector, produce a muon that traverses the detector volume, and have a high light level (a proxy for energy).
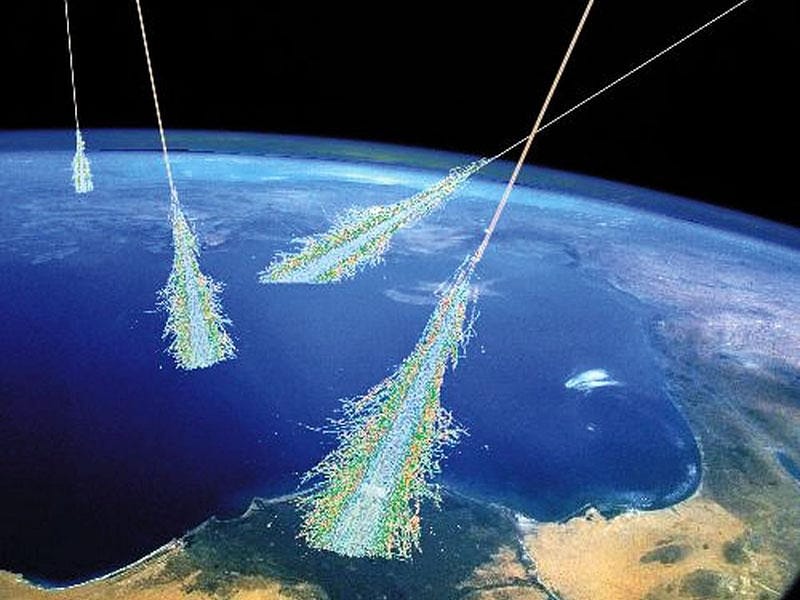
This endeavor is interesting not just for neutrinos, but for cosmic rays in general. Despite the fact that we’ve seen millions of cosmic rays of high energies for more than a century, we do not understand where most of them originate. This is true for protons, nuclei, and neutrinos created both at the source and via cascades/showers in the atmosphere.
That’s why it’s fascinating that, along with the alert, IceCube also gave coordinates for where this neutrino should have originated on the sky, at the following position:
- RA: 77.43 deg (-0.80 deg/+1.30 deg 90% PSF containment) J2000
- Dec: 5.72 deg (-0.40 deg/+0.70 deg 90% PSF containment) J2000
And that led observers, attempting to perform follow-up observations across the electromagnetic spectrum, to this object.

This is a blazar: a supermassive black hole that’s currently in the active state, feeding on matter and accelerating it to tremendous speeds. Blazars are just like quasars, but with one important difference. While quasars can be oriented in any direction, a blazar will always have one of its jets pointed directly at Earth. They’re called blazars because they “blaze” right at you.
This particular blazar is known as TXS 0506+056, and when a slew of observatories, including NASA’s Fermi observatory and the ground-based MAGIC telescope in the Canary Islands, detected gamma-rays coming from it immediately.
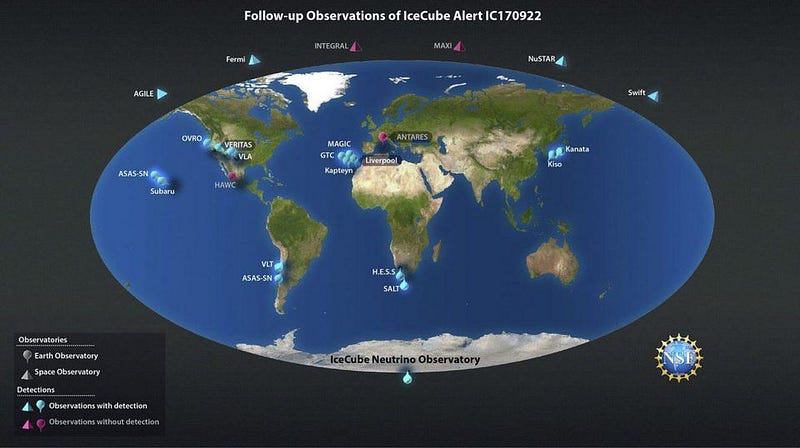
Not only that, but when the neutrinos arrived, the blazar was found to be in a flaring state, corresponding to the most active outflows such an object experiences. Since outflows peak and ebb, researchers affiliated with IceCube went through a decade’s worth of records prior to the September 22, 2017 flare, and searched for any neutrino events that would originate from the position of TXS 0506+056.
The immediate find? Neutrinos arrived from this object in multiple bursts, spanning many years. By combining neutrino observations with electromagnetic ones, we’ve robustly been able to establish that high-energy neutrinos are produced by blazars, and that we have the capability to detect them, even from such a great distance. TXS 0506+056, if you were curious, is located some 4 billion light years away.
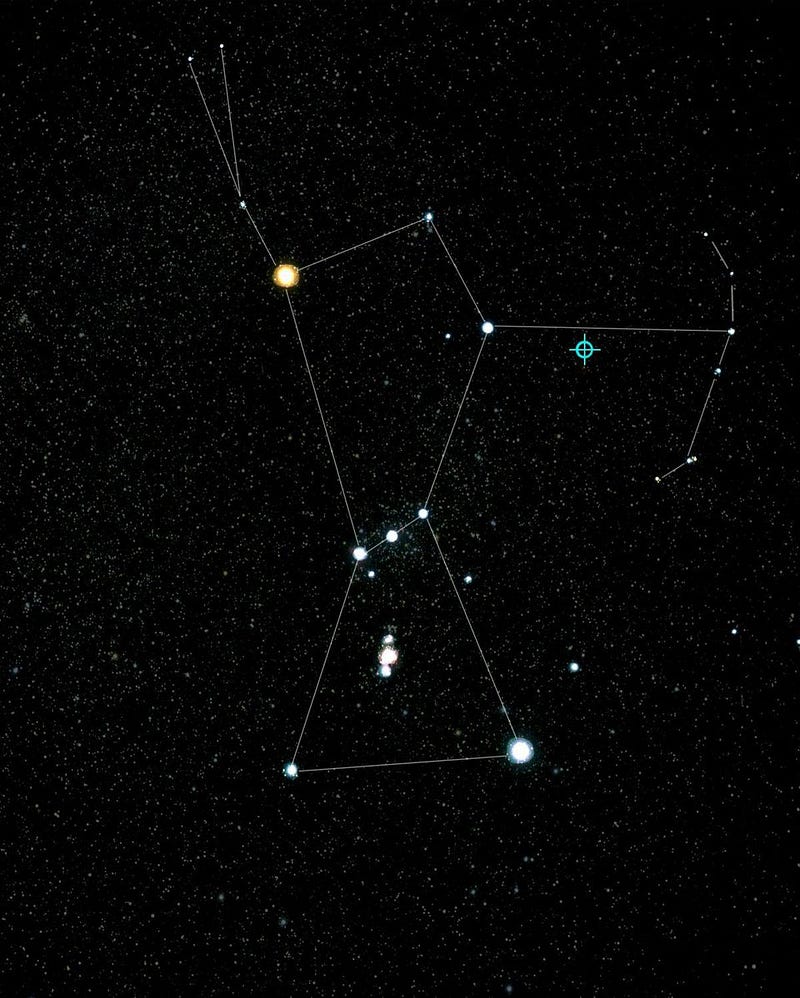
A tremendous amount can be learned just from this one multi-messenger observation.
- Blazars have been demonstrated to be at least one source of cosmic rays.
- To produce neutrinos, you need decaying pions, and those are produced by accelerated protons.
- This provides the first definitive evidence of proton acceleration by black holes.
- This also demonstrates that the blazar TXS 0506+056 is one of the most luminous sources in the Universe.
- Finally, from the accompanying gamma rays, we can be certain that cosmic neutrinos and cosmic rays, at least sometimes, have a common origin.
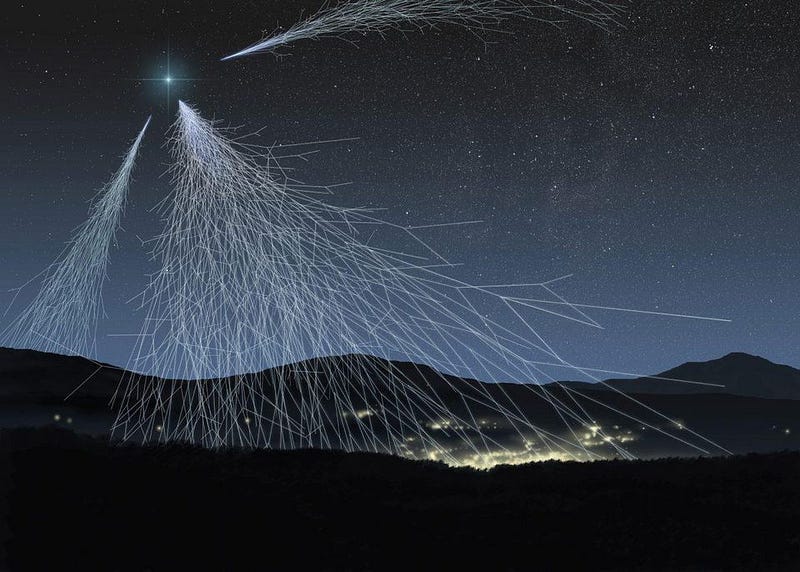
According to Frances Halzen, principal investigator of the IceCube neutrino observatory,
It is interesting that there was a general consensus in the astrophysics community that blazars were unlikely to be sources of cosmic rays, and here we are… The ability to marshal telescopes globally to make a discovery using a variety of wavelengths and coupled with a neutrino detector like IceCube marks a milestone in what scientists call “multi-messenger astronomy.”
The era of multi-messenger astronomy is officially here, and now we have three completely independent and complementary ways of looking at the sky: with light, with neutrinos, and with gravitational waves. We’ve learned that blazars, once considered an unlikely candidate for generating high-energy neutrinos and cosmic rays, in fact create both.
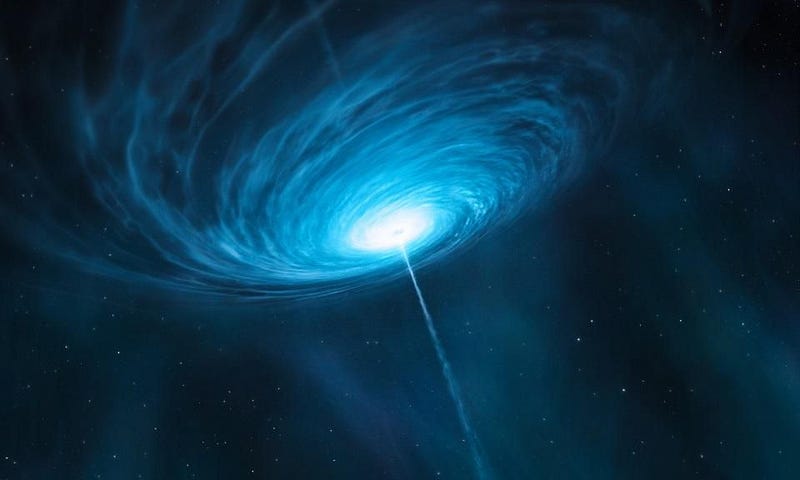
A new scientific field, that of high-energy neutrino astronomy, officially launches with this discovery. Neutrinos are no longer a by-product of other interactions, nor a cosmic curiosity that barely extends beyond our Solar System. Instead, we can use them as a fundamental probe of the Universe and of the basic laws of physics itself. One of the major goals in building IceCube was to identify the sources of high-energy cosmic neutrinos. With the identification of the blazar TXS 0506+056 as the source for both these neutrinos and of gamma rays, that’s one cosmic dream that’s at last been achieved.
Ethan Siegel is the author of Beyond the Galaxy and Treknology. You can pre-order his third book, currently in development: the Encyclopaedia Cosmologica.