Exclusive interview: answers to 20 questions from the James Webb Space Telescope team
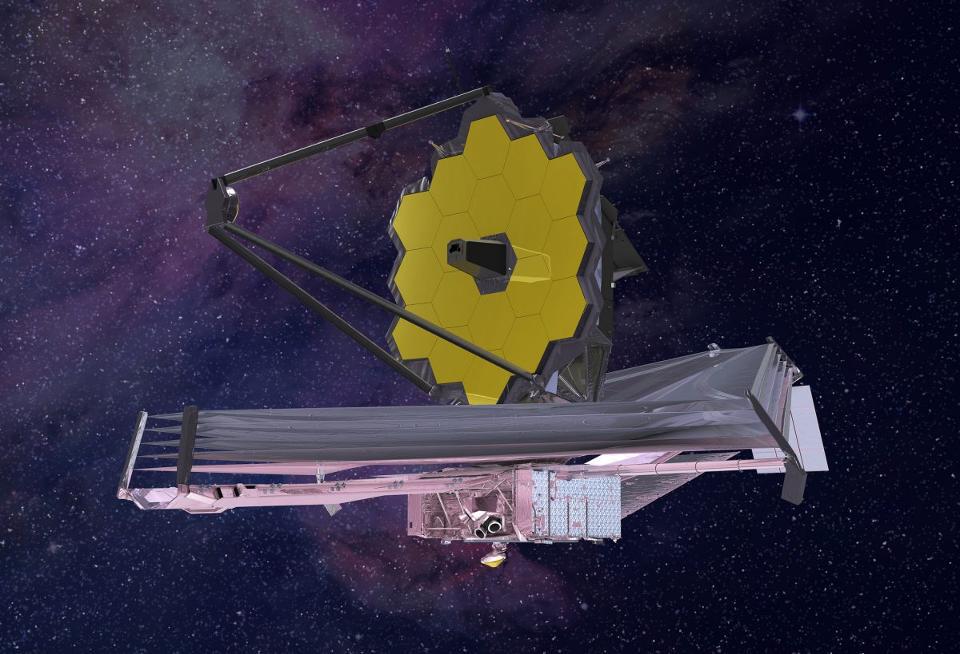
- With an incredibly successful launch, deployment, and journey to its final destination now complete, NASA’s James Webb Space Telescope is slated to begin science operations in mid-2022.
- Two main instruments aboard the telescope, the near-infrared NIRCam and the mid-infrared MIRI, will be the primary cameras that will unveil the Universe as never before.
- In an exclusive “20 questions” interview with nine members of those teams, an insider’s view of what awaits James Webb now awaits us all, too.
On Christmas Day, 2021, a new era in astronomy began as the James Webb Space Telescope rocketed into space. A perfect launch from an Ariane 5 rocket sent the telescope speeding away from Earth so that, after a month-long journey, it would come to rest some ~1,500,000 kilometers away. With all of its components now fully deployed, calibration, alignment, and testing of its various systems have commenced, setting us up to begin science operations as soon as June of 2022. Armed with capabilities that no other telescope can match, Webb is poised to begin a mission that could last as long as 20 years to revolutionize what we know about our place in the Universe.
We can expect an explosion of new knowledge across a wide variety of fields, including:
- unprecedented imagery of planets and moons in our own Solar System,
- understanding what’s in the atmosphere of the nearest Earth-sized exoplanets,
- never-before-seen views of the protoplanetary disks surrounding newborn and newly forming stars,
- the earliest and most distant galaxies ever seen,
- and quite possibly, for the first time, stars made out of material that’s been untouched since the earliest moments of the hot Big Bang.
But these scientific advances will only be possible because of the incredible capabilities of the novel instruments aboard the James Webb Space Telescope, and it’s the unsung heroes of astronomy — the instrument scientists who’ve spent years working to understand the very limits of their capabilities — who are indispensable in making it all possible.
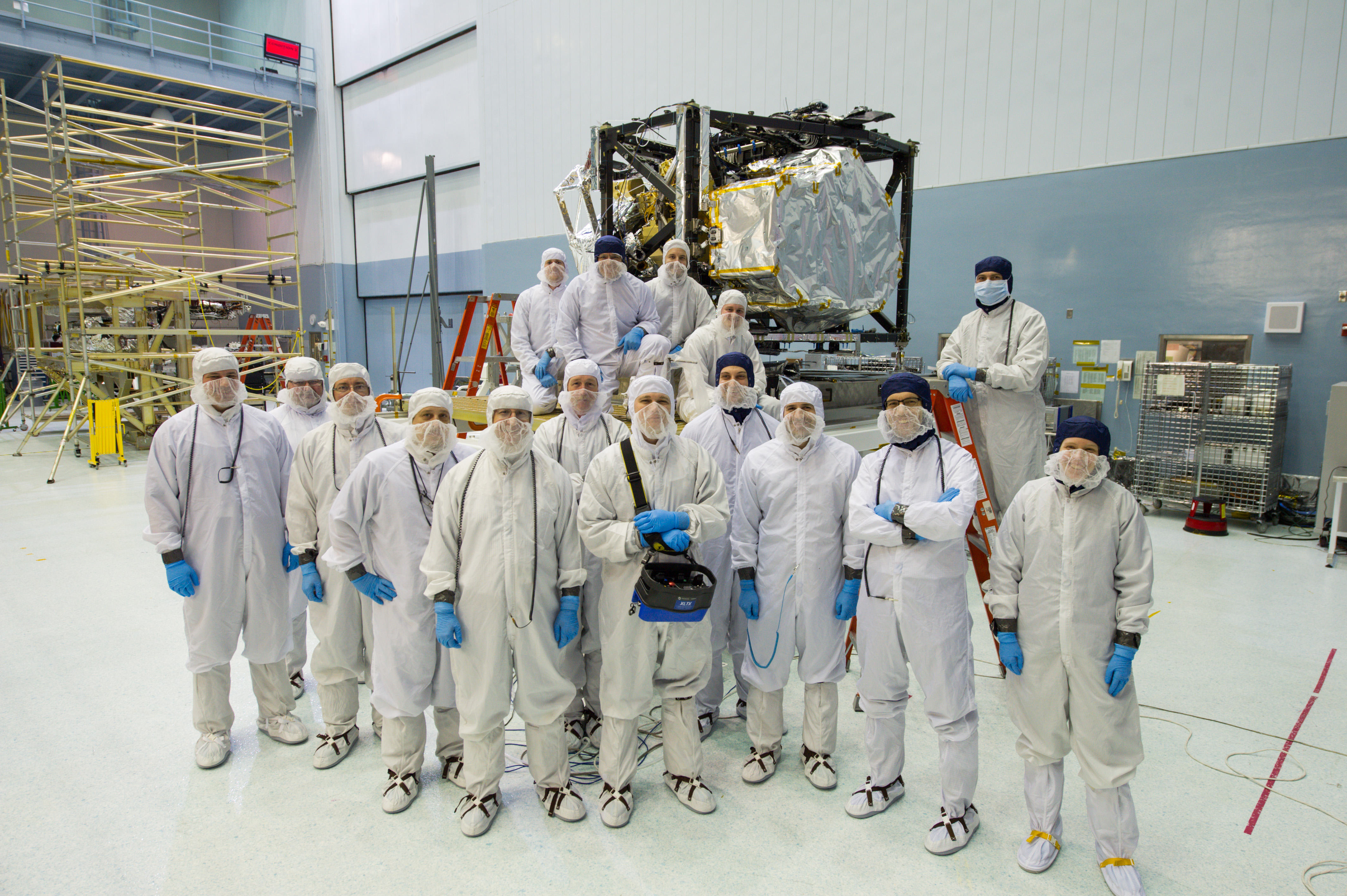
(Credit: NASA/Chris Gunn)
While the novel, 5-layer sunshield and the segmented gold mirror aboard the James Webb Space Telescope are the most striking features to look at, the capabilities of the instruments housed inside are at least as important. Designed to measure the Universe primarily in the infrared — in longer wavelengths of light than the human eye can see — each of the four instruments contained within the Integrated Science Instrument Module have their own unique capabilities, but also their own limitations.
- The Near-Infrared Camera, or NIRCam, is the primary imaging camera aboard the James Webb Space Telescope, and it is ideal for seeing through the interstellar dust that blocks most of the light that’s visible to human eyes.
- The Near-Infrared Spectrograph, or NIRSpec, is specialized for measuring the cosmic “fingerprint” of atoms and molecules that are present in any astrophysical object.
- The Mid-Infrared Instrument, or MIRI, contains both a camera and a spectrograph, and can reveal planets, comets, asteroids, warm interstellar dust, and even protoplanetary disks around newly forming stars. It probes the longest-wavelength light that Webb can see: up to 28 microns, or some 40 times longer than the maximum wavelength human eyes can see.
- And the Fine Guidance Sensor/Near Infrared Imager and Slitless Spectrograph, or FGS/NIRISS, helps point the telescope and will detect, characterize, and measure the atmospheres of exoplanets.
What are these instruments all about? Who works on them? And what will they help us accomplish once science operations begin?
Thanks to nine professional astronomers, all presently assistant research professors, working on the various instrument teams — Everett Schlawin, Jarron Leisenring, Stacey Alberts, Andras Gaspar, Irene Shivaei, Thomas Beatty, Christina Williams, Schuyler Wolff, and Kevin Hainline — here are in-depth answers to 20 of the most probing questions we can ask about the James Webb Space Telescope (JWST) and its science instruments.
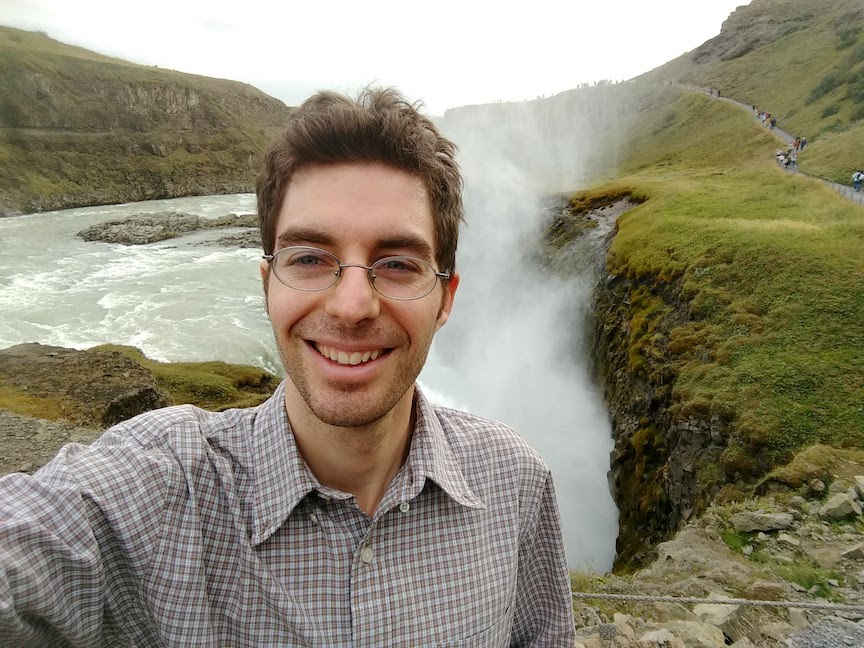
Q1.) What is it that an instrument team does for an observatory like the James Webb Space Telescope?
Answer (Everett Schlawin): The telescope is like a huge bucket that collects light from the Universe, but an instrument team is responsible for collecting that light at the bottom of the bucket so we can get pictures back at Earth. For the Near Infrared Camera (NIRCam), for example, the team began long before I started with Marcia Rieke and the original team’s proposal to build and deliver the instrument. That meant designing, building, testing and now turning on and calibrating the camera. The instrument team builds and configures the camera’s electronics, detectors, moving parts, heaters, sensors, lenses, mirrors, supports and communication with the telescope’s brain. In the end, the goal is to make it as easy as possible to point JWST to an interesting object and collect amazing pictures and spectra from it.
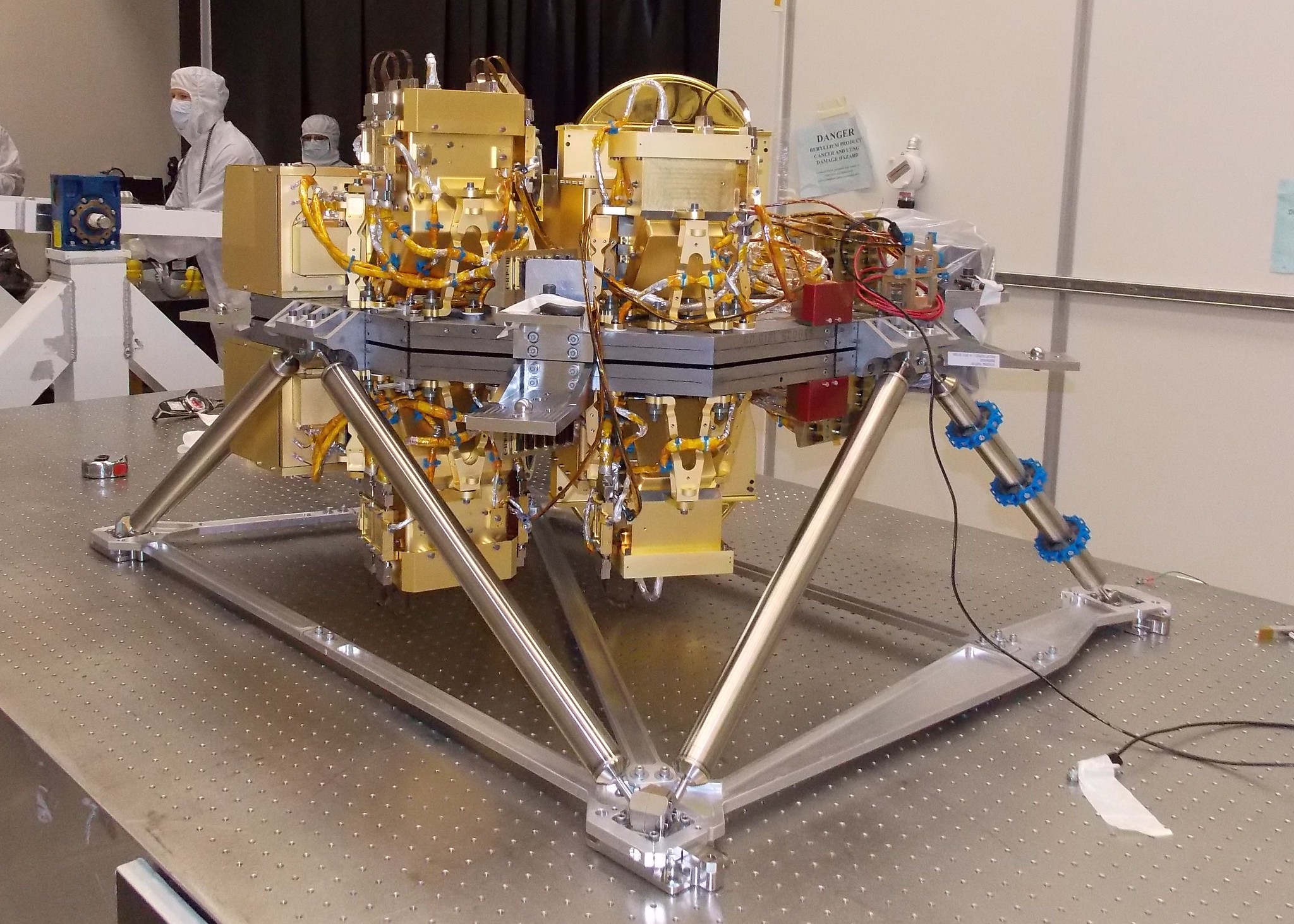
Q2.) It was widely reported that the science instruments aboard James Webb were largely complete some 10+ years ago. If that’s the case, why do we need such large instrument teams with such a wide variety of expertise?
Answer (Everett Schlawin): Even after building an instrument, the work is not done. In some cases, parts may need to be replaced. [Note: see the following question for an example!] The bulk of the work of the large team is making sure the instruments will function as expected. They were shaken, vibrated and blasted with sound waves, evacuated of air and frozen to -387 Fahrenheit. After all of these hardships, which were designed to emulate launch and space, we made sure they still functioned. We needed a variety of experts to make sure that the camera can work on the brightest objects in the night sky you can see with your eye (Solar System objects) but also the faint galaxies nearing the edge of the observable Universe. We needed engineers to make sure the motors and wheels spin right. We needed a whole contingent of colleagues in Baltimore Maryland to interface with the mission operations center and management. All of these steps will allow JWST to return pictures and spectral “rainbows” of planets, stars, galaxies, gas clouds, disks of dust, black hole neighborhoods and anything new to be discovered.
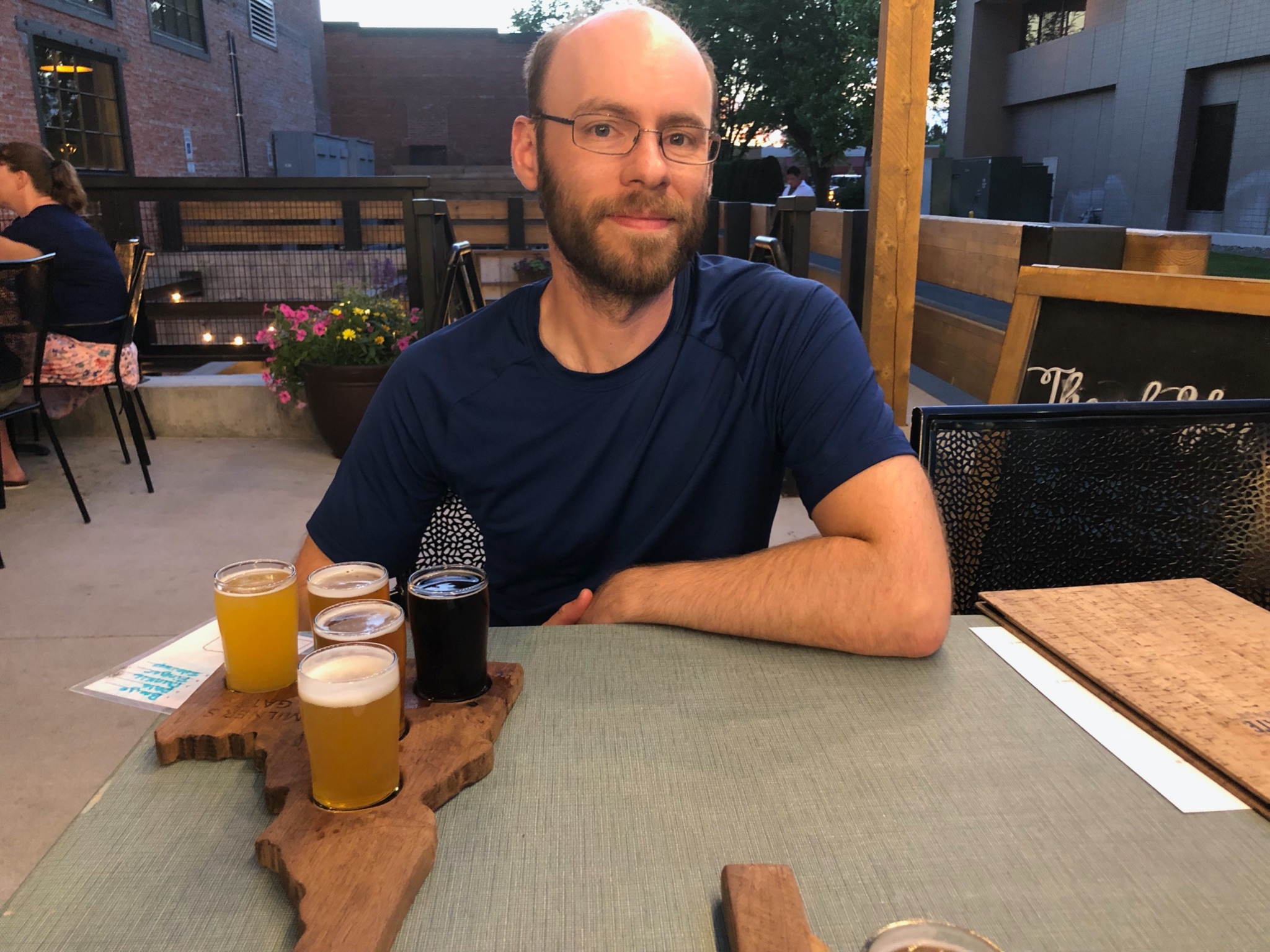
Q3.) I understand there was a major problem with the first batch of detectors, designed by Teledyne, for the James Webb Space Telescope. What went wrong, and how did the instrument team help in remedying the problem?
Answer (Jarron Leisenring): During routine testing of detectors in 2010, two independent instrument teams (from NIRCam and NIRSpec) discovered that numerous pixels on their detectors had begun to degrade only a few years after they were manufactured. A review board was convened to investigate the problem, determine the root cause, and estimate its impact on the telescope’s science performance. The review quickly found the root cause to be a design flaw that broke down the pixels by allowing an indium-gold intermetallic to form. Identifying and resolving this issue required a well-rounded team of engineers and scientists with diverse skills and backgrounds. A fix to the manufacturing process was subsequently implemented, and a new batch of detectors were quickly manufactured. Ultimately, the new detectors turned out to be much better than the old ones even prior to the degradation, because they benefited from additional enhancements that had since been implemented in the manufacturing.
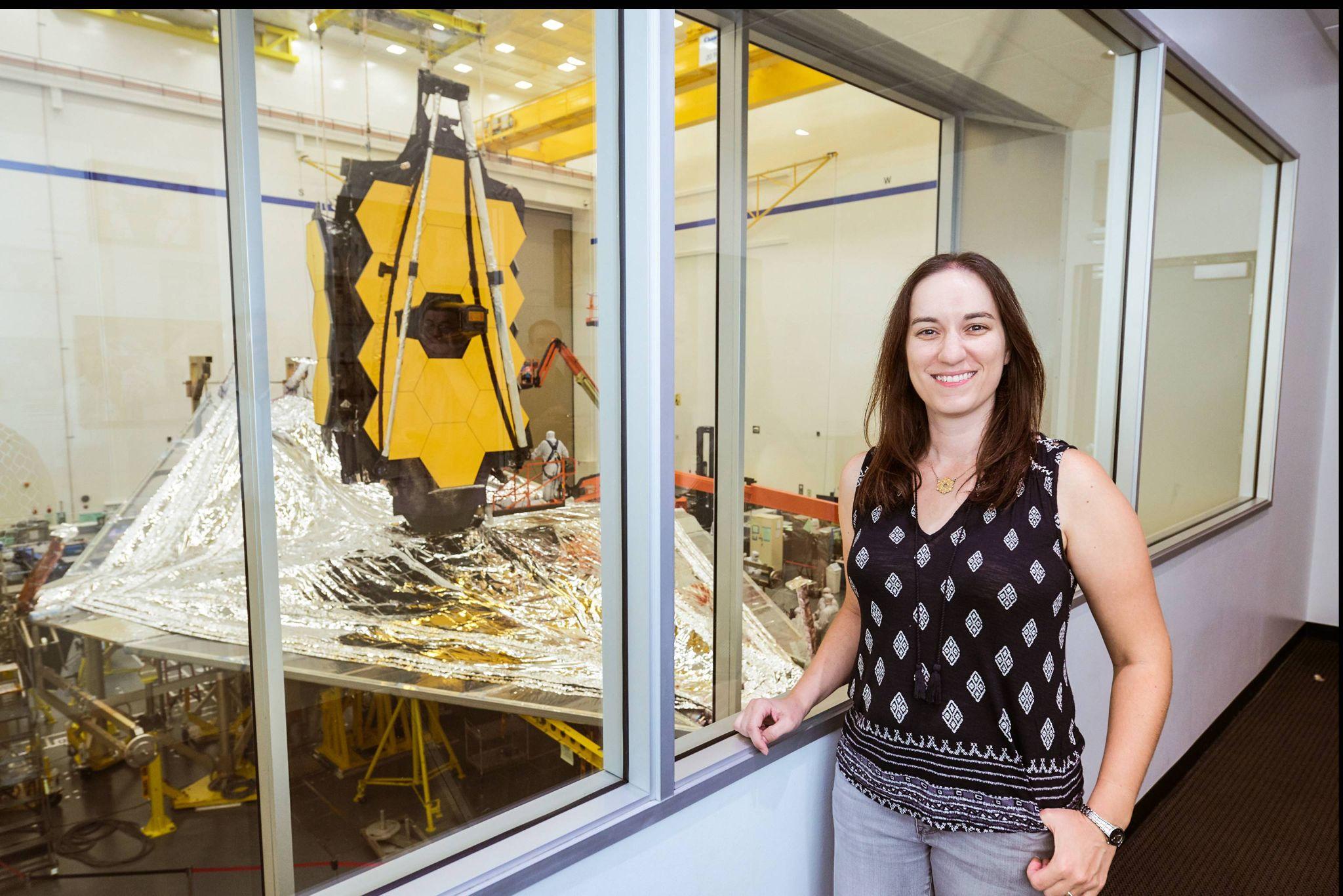
Q4.) Why would an astronomer, particularly an astronomer who’s specialized in observational astronomy like most of you, want to be a part of an instrument team like the one(s) you’re on?
Answer (Stacey Alberts): I think like most grad students applying for their first postdoctoral jobs, I was envisioning the traditional career path. In astronomy, that usually means a couple 2-3 year postdoc positions on opposite sides of the world and then the hopeful but scary bid for one of the competitive professorships. I applied to my current job on a JWST instrument team on a whim, thinking I was surely unqualified. But when George Rieke (science lead for MIRI) emailed me and said let’s talk, I began to think about a more non-traditional path.
Being part of a project like JWST, working on both early science plans and the instrument development and calibration needed to make them happen, is a once in a lifetime opportunity to work with a huge team to make something miraculous a reality. In academia, we often emphasize standing out in a crowd, but something like JWST rides on the shoulders of thousands. For young observational astronomers like me, working on JWST meant that in addition to the science we had been trained to do, we got to do a deep dive on how the sausage is made, testing the instruments at Goddard Space Flight Center while JWST’s mirror was being assembled in the next room. We got to go to Johnson Space Center and take and analyze detector readings while JWST sat in a cryovac chamber built for the Apollo missions. And now we get to run commissioning activities, working alongside the amazing people in charge at JWST’s Mission Control in Baltimore. We literally get to say “we are go” for commands that are sent to JWST a million miles away. And when the science starts, we will be the first in line to identify idiosyncrasies with the data (because as we know better than most, every instrument has its bad behaviors!) and understand them, enabling better science for everyone.
Many of us spend a lot more time (5, 10, 20 years!) in these roles than the traditional path would recommend. Some have even said to us these jobs are a bad idea if you want to stay in academia long term. And to that I say, “Want more telescopes like JWST?” Me too.
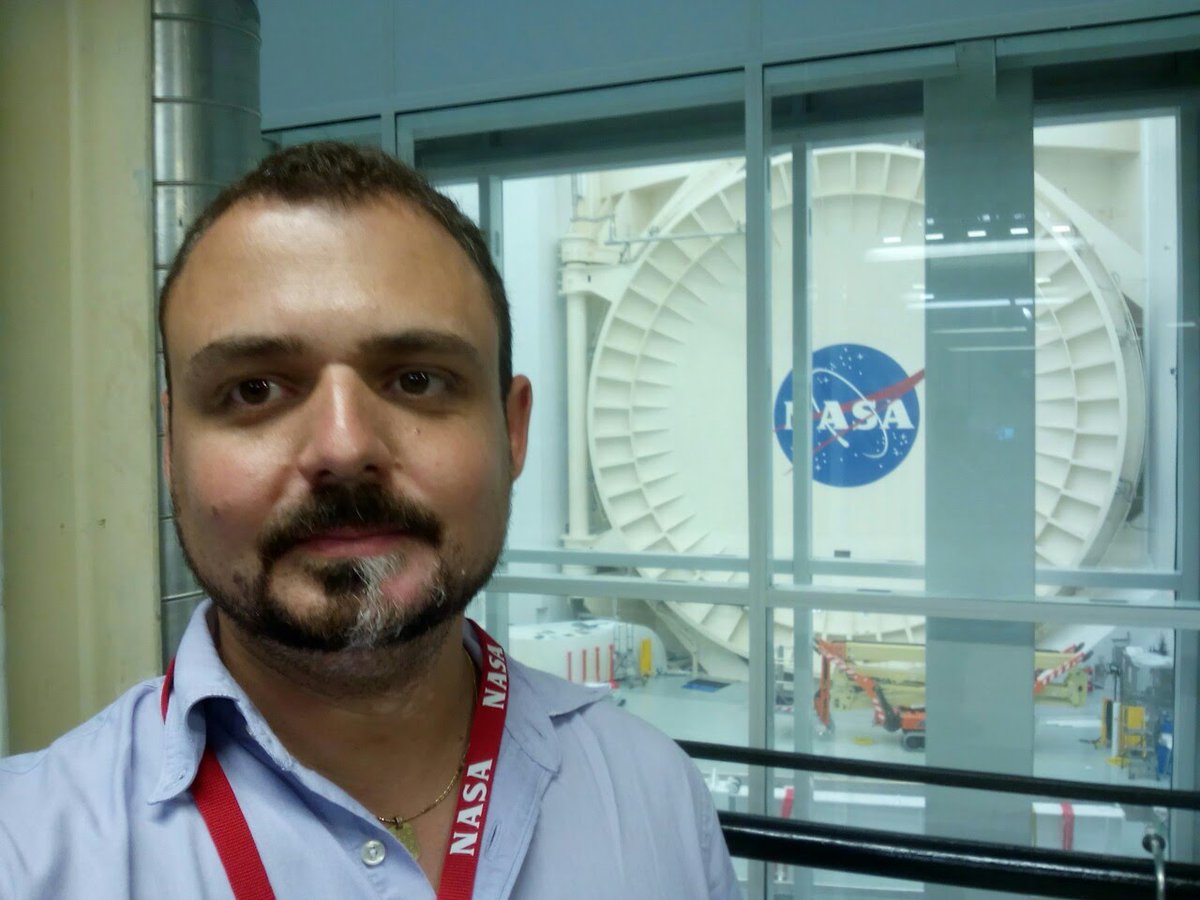
Q5.) I have heard some astronomers complain that members of the instrument team get observing time “for free” instead of having to compete for it like everyone else. Yet when I look at what members of the team are using their observing time for, it seems as though it’s “slam-dunk” science that will undoubtedly benefit the community. What would you say to astronomers who don’t agree?
Answer (Andras Gaspar): When a new observatory or instrument is commissioned, there is an understandable eagerness in the community to use it to unlock the mysteries of the most exciting astronomical objects. The astronomers on these science instrument teams, who have devoted a significant portion of their careers to design, compete for, build, test, verify, deliver, and then commission these instruments to be used by the community at large, have also spent years thinking about various science cases for these specific instruments, cases that will demonstrate their new, unique capabilities. Providing a small fraction of the total observing time — 16% in the first three years, in the case of JWST — to these teams ensures that scientists are able to afford to join instrument teams without sacrificing their research careers.
For example, the NIRCam contract (PI: Dr. Marcia Rieke) was awarded to the University of Arizona in 2002: even before the launch of Spitzer. Some members of the science team have been working on this instrument for over 20 years! Other members, such as us, are early career scientists who have devoted time to testing and calibration in lieu of work that is seen as more competitive in the academic job market! Assuming JWST will work for the next 20 years, as is anticipated following the extremely precise launch by Ariane 5, the total Guaranteed Time Observations (GTO) time will be less than 3% of the observatory’s cumulative observing time. That’s not a lot. In addition, apart from understanding the astrophysical nature of the objects they wish to observe, science instrument team members also have deep-level knowledge of the anticipated behavior of the instrument they worked on. Having us design and analyze the very first observations will benefit everyone. By the way, we are also happy to help and collaborate with anyone that would like to have the input from an instrument team member.
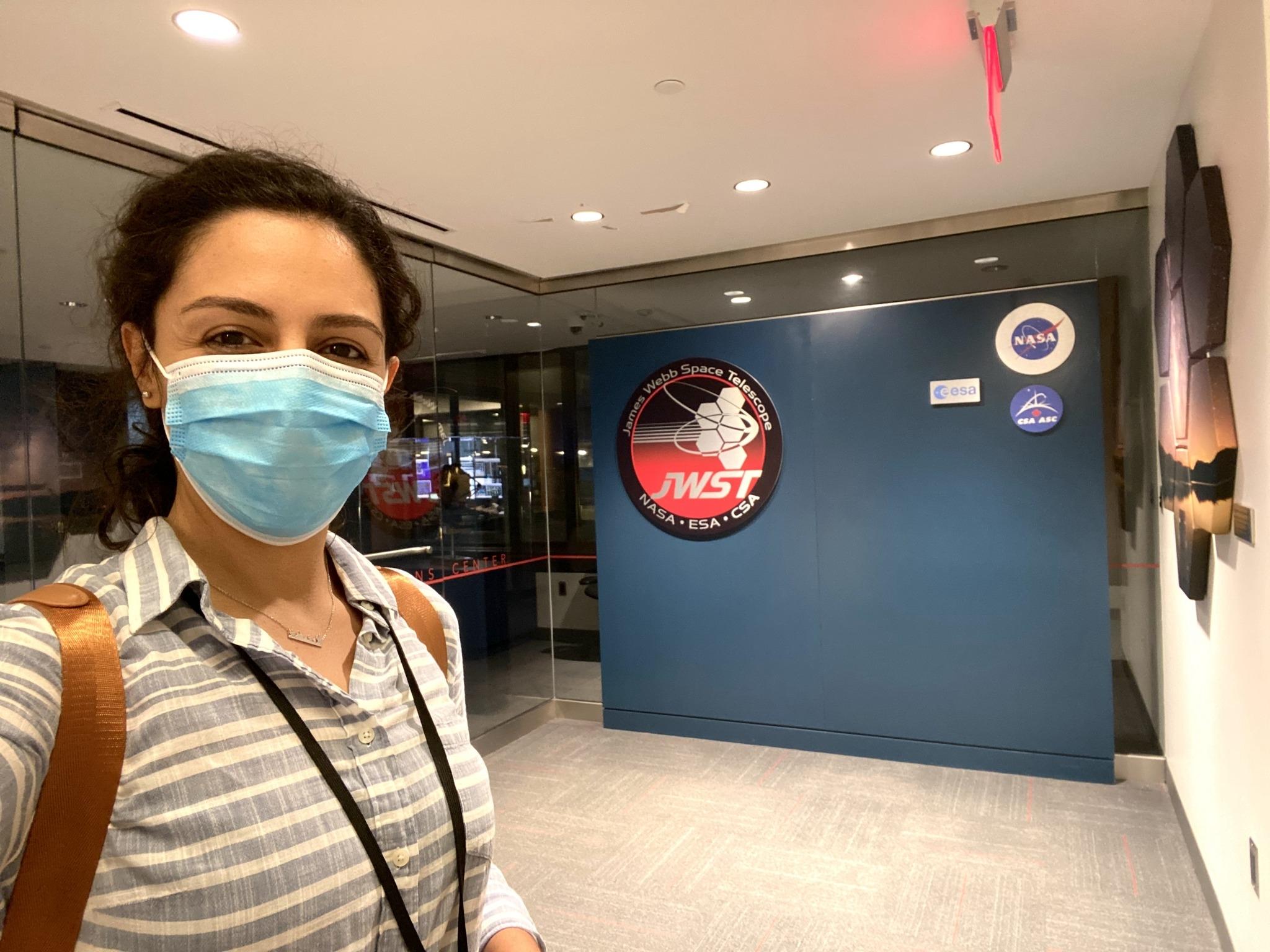
Q6.) James Webb has been called “the successor to Hubble,” but from a science point of view, that’s not quite right. Is there a better analogy out there, given that James Webb’s wavelength range is so different from Hubble’s?
Answer (Irene Shivaei): Although James Webb is the formal successor to Hubble (HST), if you think about the wavelength range that will be observed by James Webb, it is also a successor to Spitzer, as both James Webb and Spitzer are infrared telescopes. With its larger mirror and more advanced technology, James Webb will complement and push the scientific achievements of both of these telescopes into new frontiers.
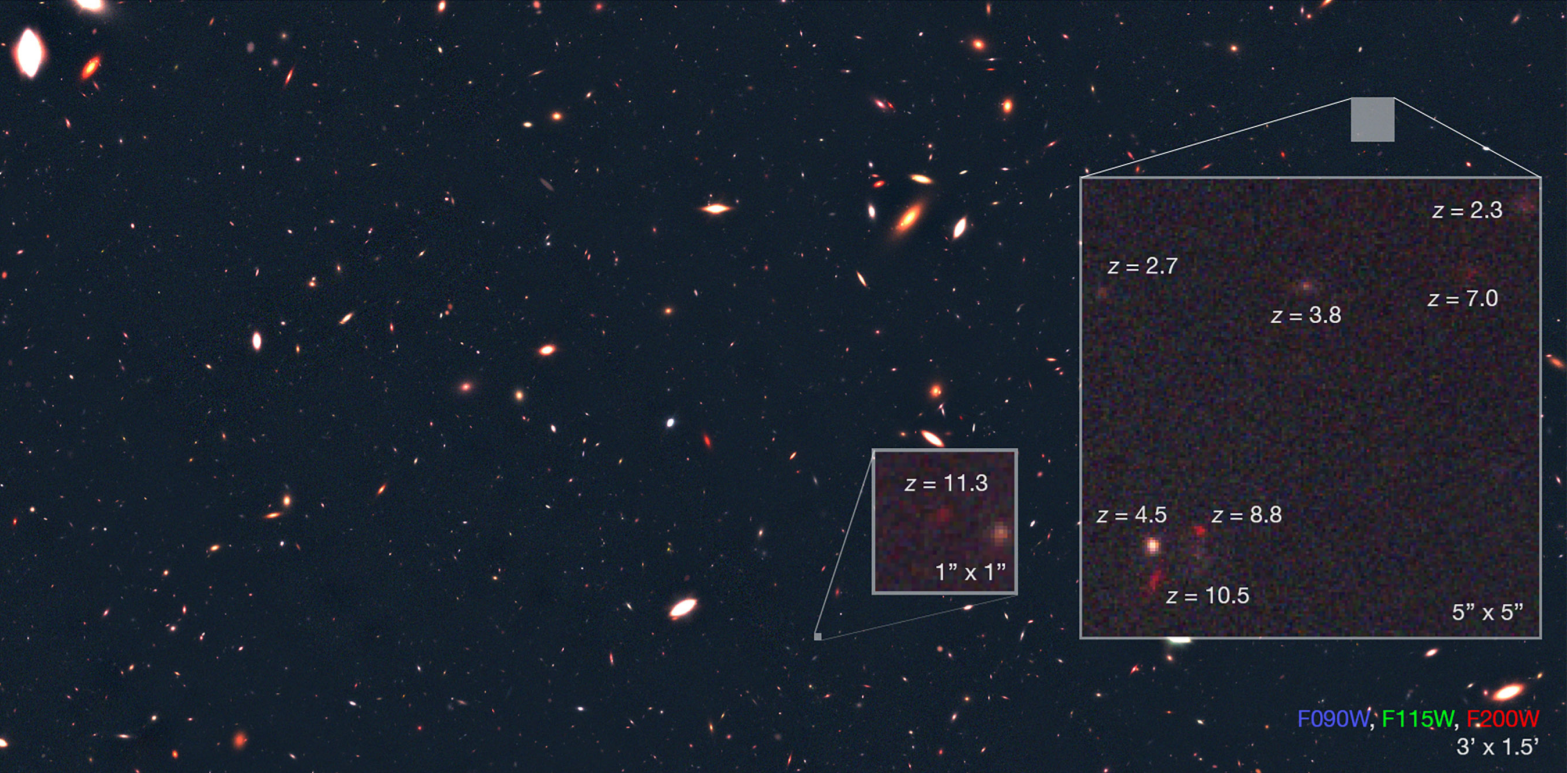
Q7.) There are a lot of people saying that “James Webb’s images might not be as spectacular as Hubble’s,” but many members of the instrument team disagree with that. What should we expect from James Webb as far as images go, and will there be a difference with images taken by, say, NIRCam from those taken with MIRI?
Answer (Andras Gaspar): This is a very interesting question. Before giving an answer, let me highlight an important fact: many of the new science observations from JWST will come from spectroscopic and not imaging observations; meaning we may not have “pretty images” with all new results, but rather high confidence detections of certain elements at infrared wavelengths. Now, in cases where we will have images, the beauty of those images will really be in the eye of the beholder. There are three variables to consider here when comparing images taken with JWST or HST, or even different instruments within JWST, e.g. NIRCam or MIRI: signal-to-noise ratio, spatial resolution, and observing wavelength.
The most obvious difference between JWST and HST is the size of their primary mirrors; while HST has a decent 2.4m diameter primary, it is overshadowed by JWST’s enormous 6.5m mirror! As JWST’s aperture is ~3x larger in diameter, it will be able to accumulate ~9x as many photons as HST in the same amount of time, resulting in really high signal-to-noise images. This means we will be able to see faint details that we haven’t been able to before in much shorter observation times.
For spatial resolution, I like to use the motorcycle vs. car headlights analogy: with a bigger telescope, you are able to determine whether a vehicle in the distance has a single or two headlights. As JWST has a diameter 3x that of HST, if observing at the same wavelength, JWST will have a 3x better spatial resolution! Note, that I said “if” observing at the same wavelength. In fact, JWST will be observing at a wide range of wavelengths, starting at the high-end of HST’s to 30 times as long as HST’s. Because of this, the spatial resolution of the images provided by JWST will be slightly better/on-par at its shorter wavelengths and about an order of magnitude worse at its longest wavelengths than that of HST. However, spatial resolution is not the most important factor!
The “piece de resistance” of JWST is its wide infrared wavelength range! Compared to HST, which works in the near-UV to near-IR wavelength range (0.2-1.7 microns), JWST will observe between 0.7 to 30 microns, enabling the study of a wide range of new and faint astrophysical phenomena at much higher spatial resolution than we were able to achieve previously at each particular wavelength. To demonstrate with dust: at 0.7 microns, interstellar dust obscures the light of background stars, at 1.5 microns, dust will scatter light, at 3 microns, dust can glow hot (e.g., in a circumstellar disk as it spirals into its host star), at 15 microns dust will glow cold in the asteroid belts of other stars, and at 30 microns, we get to see even colder dust populations in the Universe emit light. The same component, dust, will be observed in wildly different settings with a single observatory! Finally, the infrared images taken by JWST — much like as was with Spitzer — will be represented with “false” optical colors, resulting in spectacular colorful images that we can all enjoy, even though we cannot see in the infrared ourselves.
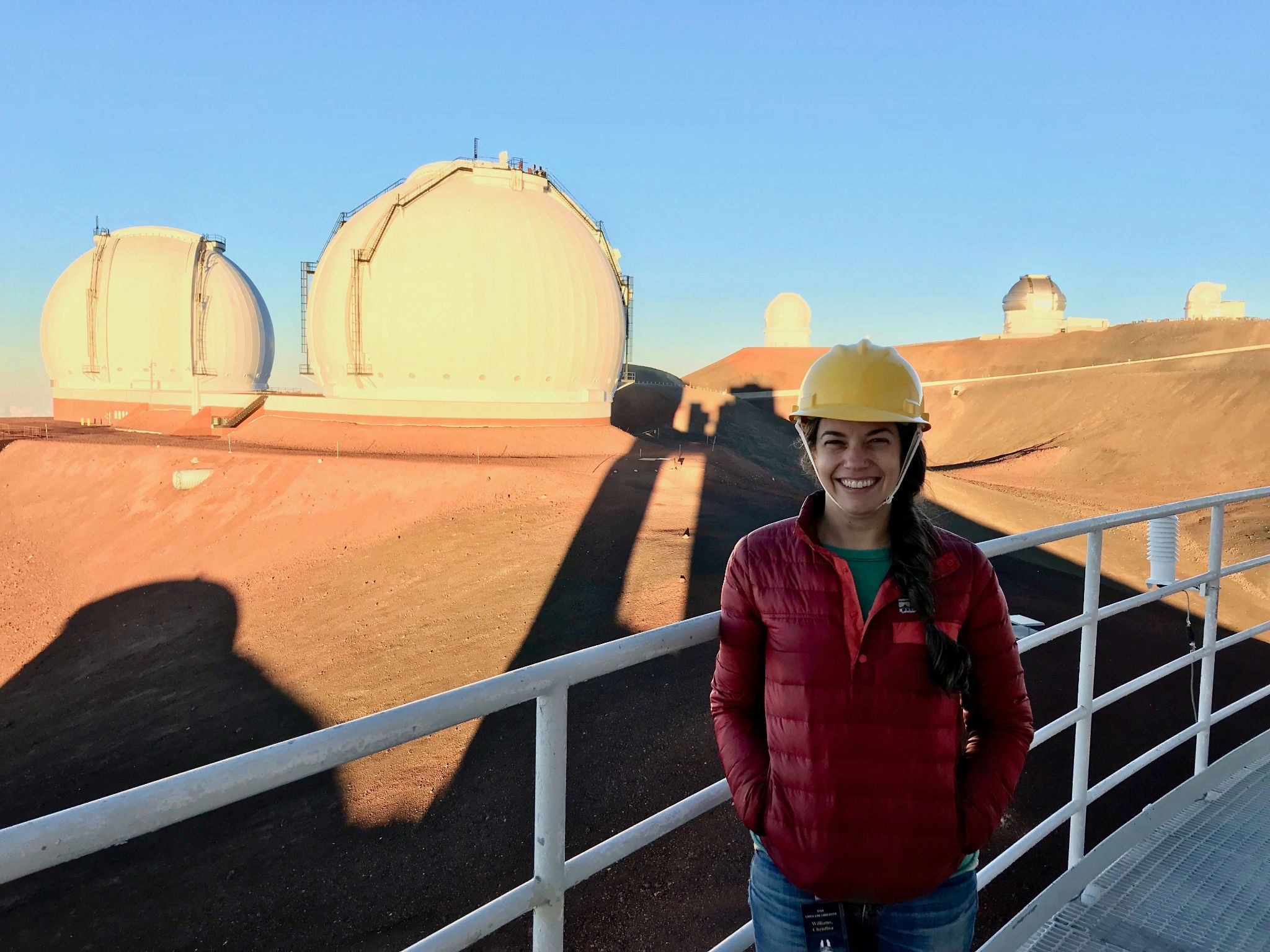
Q8.) When we look back into the distant Universe, we’re seeing back in time as well. With Hubble, we can look back and see the Universe in its infancy: like seeing a human that’s a 1-year-old baby. With James Webb, some have said it’s like seeing a 1-day-old baby instead. What gives Webb such unprecedented power?
Answer (Christina Williams): The light from distant galaxies is dimmed and stretched to infrared wavelengths from the journey across the expanding Universe. This makes them incredibly hard to see and identify because they are super faint and very red. Two characteristics of JWST make it our eyes to the very beginning of galaxies: It is super sensitive to faint light while also being able to see in the near and mid infrared. JWST is the first telescope with a large enough mirror and cold enough instruments to see the first galaxies forming!
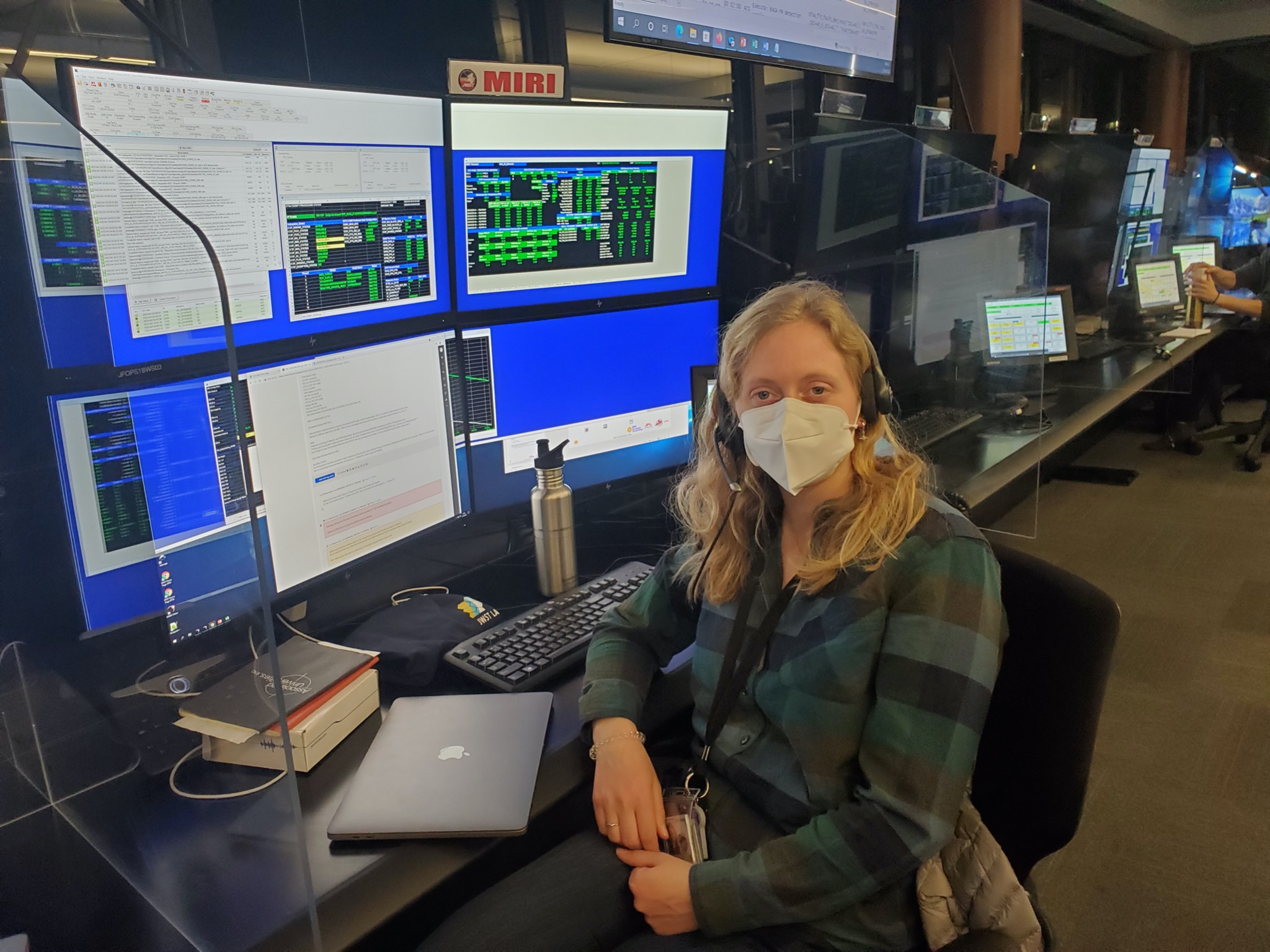
Q9.) Part of the reason an instrument team is so important is that you have the same minds, the same people, thinking about a slew of issues that could bias or contaminate your observations, as well as how to mitigate those issues. What are some of the ways that the data will attempt to fool you, and how can we compensate for them?
Answer (Schuyler Wolff): In order to place such a giant, powerful telescope into space, the mirror was split into segments which complicates the path of the light moving through the telescope optics. This “snowflake” pattern is more complex than the “cross-shaped” diffraction pattern of the Hubble Space Telescope for example. If you point the telescope at a field of stars (or point sources) you will see a field of miniature copies of this snowflake and they will be easy to disentangle. However, the problem becomes more complicated as you look at more extended structures like galaxies or circumstellar disks. These diffraction effects can begin to masquerade as clumps or asymmetries in the galaxy or disk morphologies. In order to compensate for this effect, a model is created of the innate morphology, convolved with the diffraction pattern and compared to the JWST dataset.
This effect is further complicated by the more complex modes of JWST. One of the observing modes I am particularly excited about are the coronagraphs. There are several coronagraphs onboard JWST with varying levels of complexity, but all are designed to block the light from a bright central source in order to image much fainter surrounding material. This further distorts the light path and makes asymmetries more difficult to classify. In particular, I am currently investigating how coronagraphic observations will distort images of debris disks observed around nearby stars.
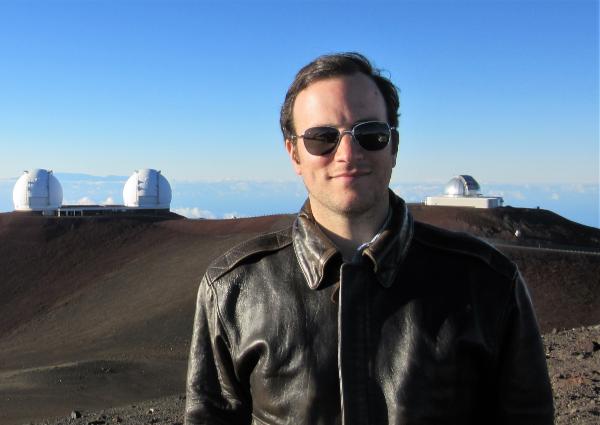
Q10.) It’s easy to get excited about upcoming record-breaking discoveries: the biggest, youngest galaxies, the earliest, most pristine stars, the most distant black hole ever seen, etc. But are there less “flashy” discoveries that James Webb should reveal that are perhaps even more important to pushing our scientific frontiers?
Answer (Thomas Beatty & Irene Shivaei): I’m going to steal some ideas from question 16, below, because one very important but not flashy area that JWST will tell us about is planet formation. For the first time, we will have the tools to conduct large, detailed surveys of exoplanet atmospheres over a range of exoplanet sizes and orbits. The hope is that eventually these surveys will reveal trends in exoplanet composition, and that these trends will tell us about their formation histories. But conducting these surveys will be real yeoman’s work, mostly spending weeks or months to measure the atmosphere of an ordinary exoplanet, sorting and filing it into the correct bin, and then starting again.

Q11.) One of the most exciting capabilities that James Webb possesses is the ability to do “parallel mode” observing. Can you tell us how this works, and whether it’s as good as it sounds: can we really get twice the data at once from a single observation?
Answer (Christina Williams): It is really as good as it sounds: you can collect data with two different science instruments at the same time with JWST. Although this doesn’t translate exactly to twice the data (different instruments collect light at different rates and from different size areas of the sky) it is an amazing way to squeeze every last bit of science out of JWST’s limited lifetime.
When JWST looks at a patch of sky, each instrument collects light from slightly different directions in that patch. This enables astronomers to use parallel mode to build their datasets in a way that uses up as little telescope “clock” time as possible. This kind of efficiency can save limited telescope resources like propellant, while also freeing up the telescope to do even more science projects. In some cases, parallel observing enables new discoveries that wouldn’t otherwise be possible. By piggy-backing on other science projects to map random directions where we have not looked before, the instrument observing “in parallel” can potentially discover new galaxies, structures in parts of the sky we have not looked before, or other things in the Universe we haven’t even considered!
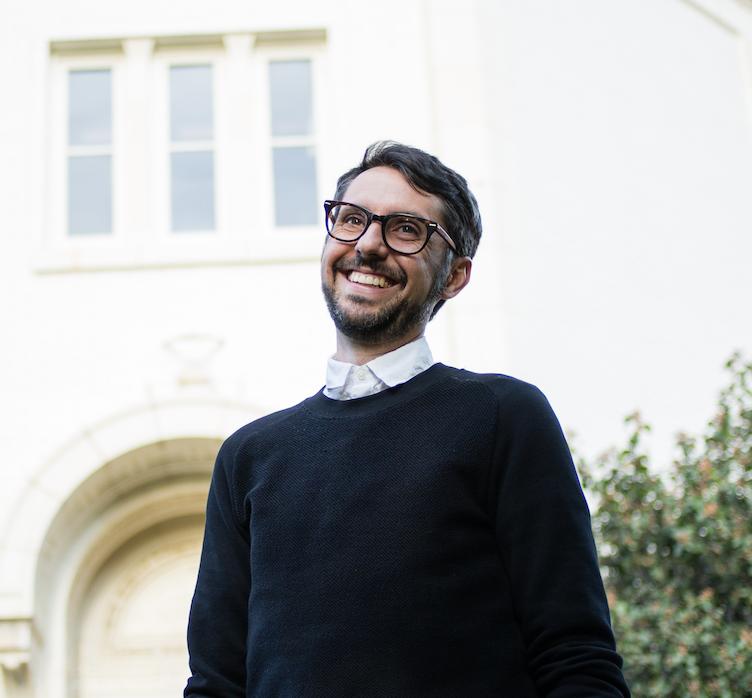
Q12.) Part of the work that your team has done to prepare for James Webb’s first science operations is to simulate what you expect the instruments to see. How have programs like JADES and Jaguar helped scientists like yourself get ready for the actual data that will be coming in?
Answer (Kevin Hainline): When it comes to exploring distant galaxies with JWST, we’re going to be uncovering objects that are far beyond our current observational capabilities from the ground or from space. As a result, we need to simulate galaxies outside of what we’ve seen: galaxies that are fainter, more pristine, more diffuse, or dustier. To that end, members of the NIRCam extragalactic team worked with members of the NIRSpec extragalactic team to create JAGUAR, a deep simulation of galaxies that we have been using for developing our strategies for JWST data analysis. JAGUAR builds on observations of the universe’s galaxies through all of cosmic time, folding in predictions from our theories of galaxy evolution to create a catalog of hundreds of thousands of simulated galaxies.
We have used JAGUAR to craft simulated images, and full “observed” galaxy catalogs recovered from those images, as we’ve been getting ready for the JADES survey, one of the largest data sets on galaxy evolution that we’ll get in the early years of JWST. JADES will combine hundreds of hours of NIRCam imaging and NIRSpec spectroscopy to study the formation and evolution of galaxies at all cosmic times. This ambitious project will result in an incredible legacy dataset, and by using JAGUAR we’ve seen how JWST will let us lift the veil on the universe far beyond Hubble’s capabilities. By simulating JADES with JAGUAR, we have learned better methods for combining our individual images, more accurate techniques for detecting faint galaxies, and efficient procedures for going through the tens of thousands of galaxies we’re going to observe. These predictions for the numbers of galaxies at all distances we are likely to recover are based on our current theories of galaxy evolution, and any differences from what we do observe will help us better craft our theory going forward.
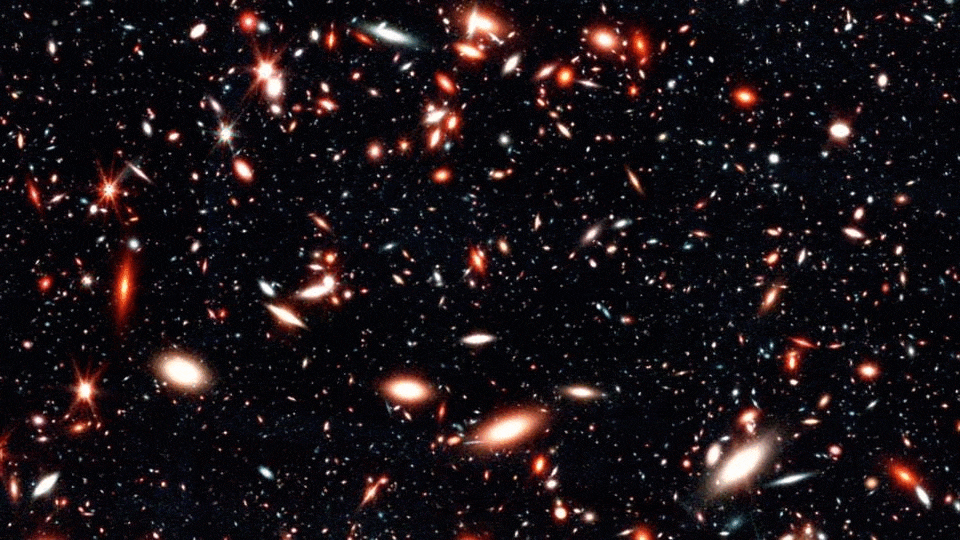
Credit: NASA/ESA and Hubble/HUDF team; JADES collaboration for the NIRCam simulation
Q13.) One of the important concepts you’ve brought up is the idea of maximizing the efficiency of the telescope. How are the observations you’ll be conducting during the first year of science operations designed to do precisely that, and what will that look like?
Answer (Jarron Leisenring): A number of the GTO science programs are designed to push the limits of the instruments. We want to do interesting science and also showcase the cameras’ unique capabilities for the community. Some of these observations are very technically challenging using the instruments in novel ways. Based on some of the accepted General Observers (GO) Cycle 1 programs, I think the GTO observations have provided good templates for the astronomy community to utilize when designing observations to answer interesting questions about the universe.
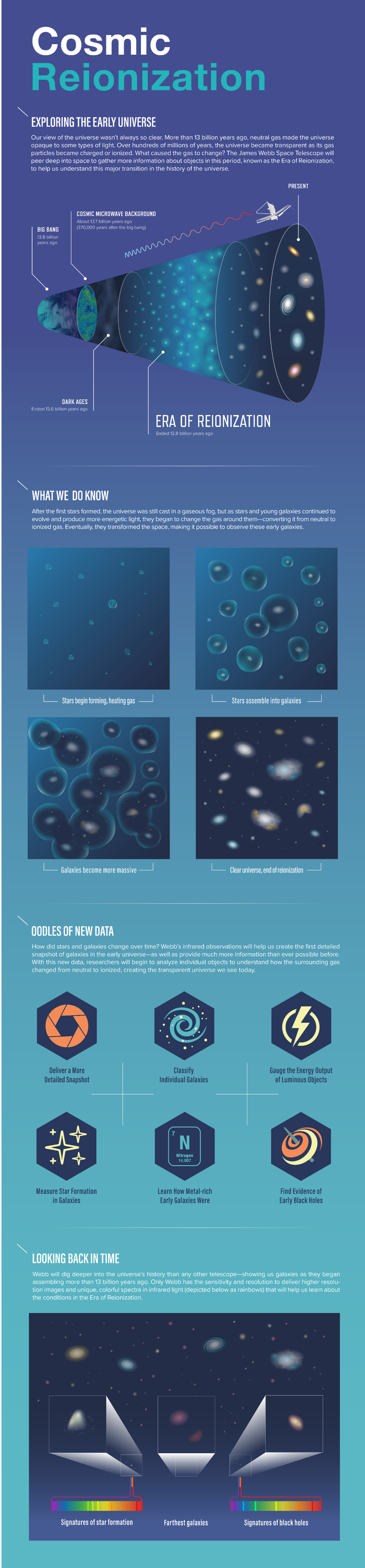
Credit: NASA, ESA, J. Kang (STScI)
Q14.) We’ve learned so much about galaxies from our previous observatories, such as how they evolve over cosmic time, how the populations of stars inside them evolves, and how the rate of star formation rises, peaks, and then plummets. What unanswered questions will James Webb help resolve, and how will we do it?
Answer (Stacey Alberts): The last few decades have been a revolution in the study of galaxies. We’ve pushed the frontier of everything from individual stars to supermassive black holes to gas and dust to dark matter, all the building blocks of galaxies that dictate how they grow and change and die over cosmic time. We’ve pushed against the limits of what we can do with our current telescopes, and discovered many, many more questions.
JWST, with its new sensitive, precise infrared view of the Universe, will blow past these boundaries and address many pressing questions.
- How do aging stars produce the heavy elements that provide the building blocks for the generation of stars and planets and life? JWST will be able to focus on individual (infra)red stars out beyond our Local Group, farther than ever before, as they end their lifecycles in red giants and supernovae, “polluting” the cosmos.
- What has remained hidden from our view behind the visible light-blocking cosmic dust that is pervasive in the most active galaxies? JWST’s infrared instruments can peer through dust to see “missing” young stars and black holes while also telling us about cosmic dust itself, which provides a surface for many of the chemical reactions needed to build more stars and planets.
- What do the smallest galaxies look like? JWST’s huge mirror provides the sensitivity to detect smaller (fainter) galaxies farther away than ever before, showing us for the first time the limits of how small you can grow a galaxy and what they looks like before they combine to form the bigger (brighter) galaxies we’ve studied for so long.
- When did the galaxies first start to shut down the formation of new stars? JWST can see farther back in time, finding the first galaxies that contain only older, red stars.
- What happened even earlier than that, during the mysterious time when the Universe first became transparent (called the epoch of reionization) when photons were first able to stream freely without being absorbed by a fog leftover from the Big Bang? JWST will reveal whether early galaxies were able to “leak” enough energetic photons to disperse that fog.
- And one of everyone’s favorite questions: what did the first galaxies and black holes look like? The UV light of those galaxies, stretched by traveling 13.7 billion years to reach us, will arrive at JWST’s mirror as infrared photons, giving us our first view of the Universe’s first structures. Likewise studying early quasars (super hungry black holes) will give us clues about how the first black holes came into being.
JWST’s unique capabilities will provide many exciting new puzzle pieces to fill in our picture of galaxies, keeping extragalactic astronomers busy for decades to come. But perhaps the most exciting discoveries of all, as we learned from JWST’s predecessors like Hubble and Spitzer, will be the things we haven’t even dreamed of yet.
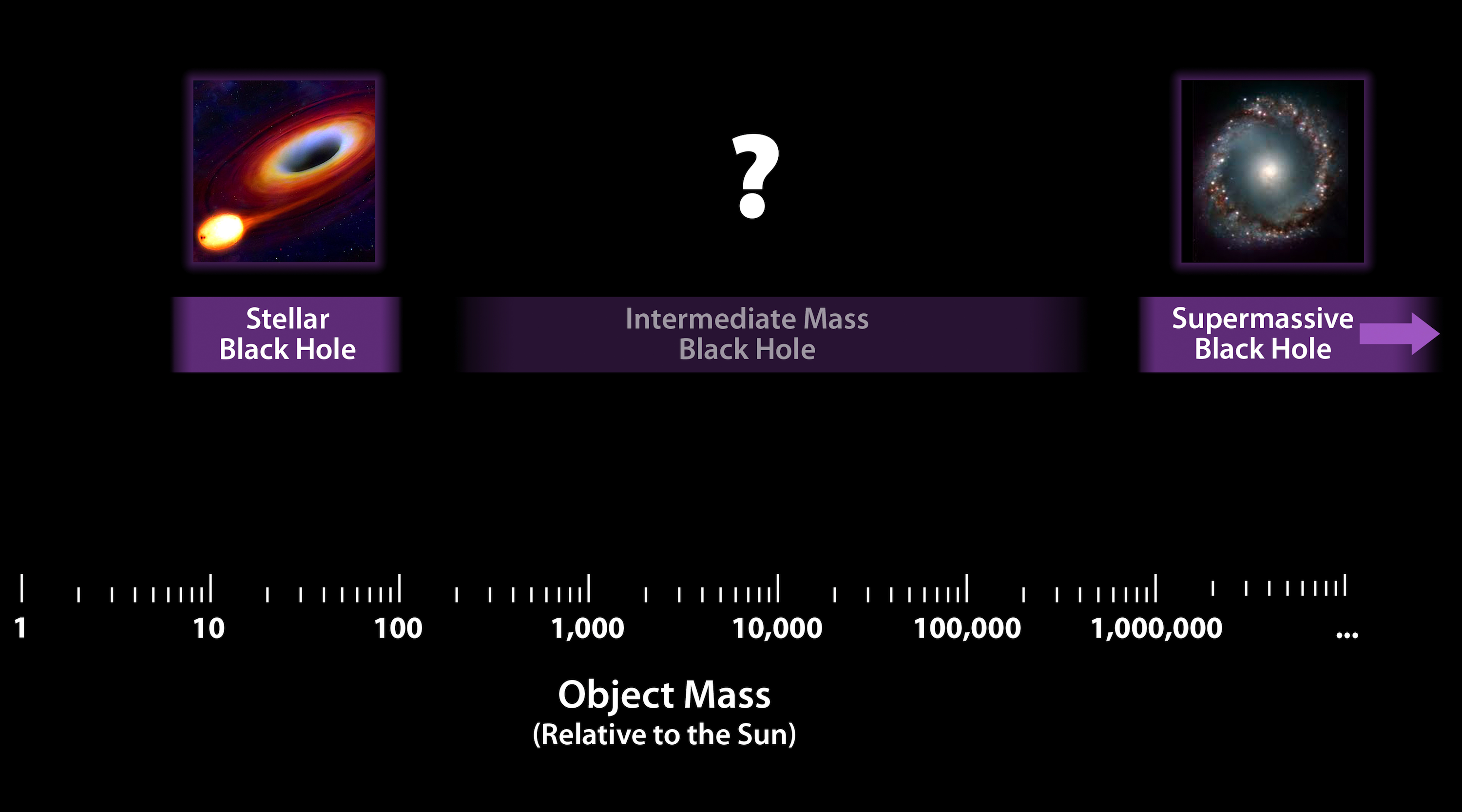
Q15.) James Webb, quite possibly, might help us understand the formation and growth of black holes in the young Universe, from “stellar mass” black holes to supermassive ones. What unique capabilities does Webb have for investigating and finding these objects, and what can we expect to learn?
Answer (Kevin Hainline): While most people assume that black holes are completely invisible to us on the Earth, there are ways that we can uncover the existence of a black hole that is actively feeding. When matter falls onto a black hole, it doesn’t go quietly, but rather it is ripped apart gravitationally and glows brightly. In fact, in supermassive black holes, when they feed, they can glow as bright, if not brighter than the rest of the galaxy around them, an incredible feat considering that these galaxies may contain hundreds of billions of stars. We call the brightest growing supermassive black holes “quasars,” and hunting for them is an entire subfield of extragalactic studies. Understanding the way that black holes have grown from those that are the mass of our Sun to those that are the mass of tens of billions (~1010) of Suns is an important goal of galaxy evolution studies, and JWST will make great strides in that arena, especially as we search for quasars in the early universe.
Many of the common methods used to search for these growing supermassive black holes require observations of galaxies at wavelengths that, for very distant galaxies, have been redshifted to the infrared. So, in order for us to find the youngest quasars, we need sensitive imaging and spectroscopy in the infrared. JWST NIRCam and NIRSpec will allow us to find and characterize thousands of young quasars, enough to understand how they grow alongside the galaxies around them, and perhaps more importantly, how the presence of a bright, growing supermassive black hole can change their host galaxies by starting or stopping star formation. In addition, the JWST instrument MIRI can work on understanding these growing black holes for more nearby galaxies, as the mid-infrared capabilities of MIRI are ideal for peering through thick columns of dust to see “obscured” quasars that cannot be observed via any other methods. Together, JWST will give us a census of black hole growth in the universe that will better help us see how black holes have shaped galaxy evolution.
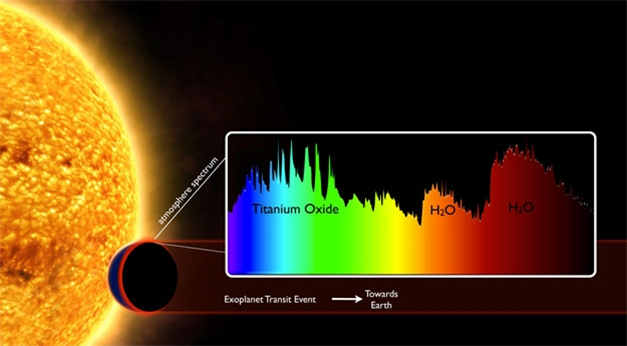
(Credit: ESA/David Sing/PLAnetary Transits and Oscillations of stars (PLATO) mission)
Q16.) Another of James Webb’s major science goals is to detect exoplanets and to characterize their atmospheres. Webb will be capable of leveraging both direct imaging and transit spectroscopy techniques in order to do so; what can we expect to learn and hope to find with these upcoming observations?
Answer (Thomas Beatty): The two broad things that we hope to better understand with JWST are: how do planets form, and which kinds of planets might have conditions like the Earth? Generally speaking, we’ve learned a lot about exoplanet atmospheres over the last two decades, but the data we have right now on exoplanets is roughly the same quality as what we had on the Solar System planets in the 1970s. That means we have some ideas about what the temperatures are on exoplanets, what their atmospheres are made out of, and if there are clouds. We’ve also mainly only been able to look at the atmospheres of giant exoplanets, roughly the sizes of Jupiter down to Neptune. But we know that smaller planets are more common than larger ones. That means we’ve never seen what the atmospheres are like on most of the planets in the universe, and the giant exoplanets we do know about we see in coarse, broad-brush, strokes.
JWST will allow us to measure the compositions of giant exoplanets in much more detail, which will give us a better understanding about how planets form. Giant planets, like Jupiter or Neptune, are the main outcomes of planet formation: most of the material in a protoplanetary disk goes into making these planets. Understanding how giant planets form is therefore very important to understanding the formation process as a whole. We hope that we can use the chemical signatures in the atmospheres of giant exoplanets to tell us about how and where their formation process took place. It’s a bit like dissecting an apple pie to figure out how it was baked. Aha! You might say, the apples aren’t mushy, so they were probably pre-cooked — or, the carbon-to-oxygen ratio is high, so it probably formed farther out from its star.
JWST will also let us look at the atmospheres of smaller exoplanets, much closer in size to the Earth. It will be very difficult for JWST to see what we call biosignatures, or evidence for life, on an exoplanet, since those are very small signals and will probably have to wait for the next-generation of space telescopes. But, JWST will help us narrow down what kind of exoplanets we think might be habitable. We really know almost nothing about the atmospheres of small rocky exoplanets, and JWST will be our first look across this new frontier.
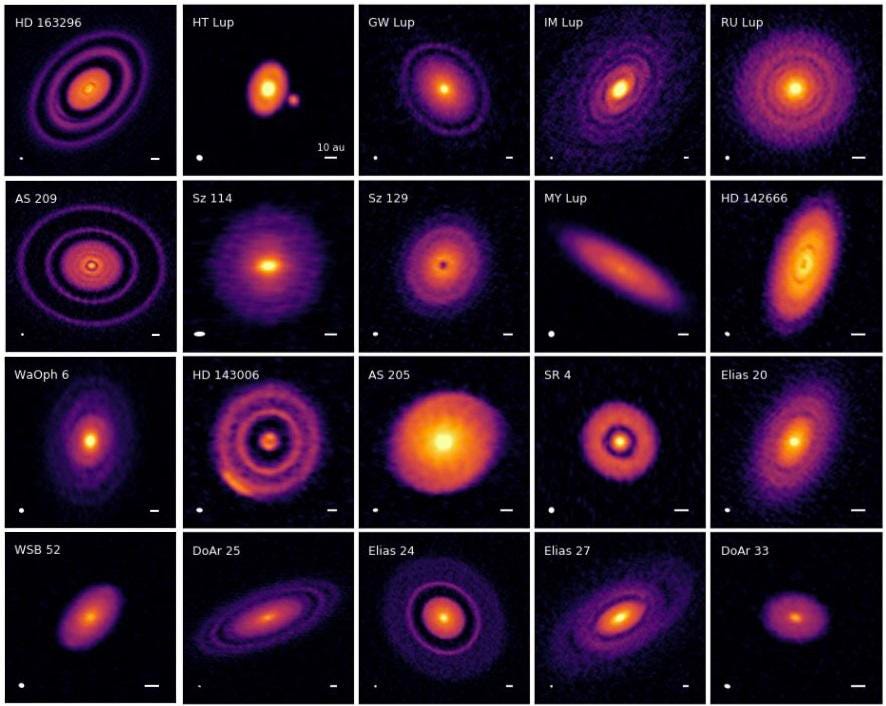
Credit: S.M. Andrews et al., ApJL, 2018
Q17.) Over the past decade, we’ve gained a much greater understanding of circumstellar disks: the environments where planets form around young and newly forming stars. From looking for water-ice to possibly seeing the first asteroid belts around other stars, what will James Webb teach us that will expand the frontiers of what we know about the Universe?
Answer (Schuyler Wolff): Circumstellar disks are the laboratories in which planets are forged. These “alchemists” transform gas and dust into “astronomical gold”: complex solar systems with gas giants, rocky terrestrial planets and belts of leftover debris. Many of the details of planet formation remain unknown and JWST is poised to answer them. In the young, gas rich protoplanetary disks, JWST will enable spectroscopic detection of complex organic molecules in the ice phase. Where ALMA has spearheaded new discoveries in astrochemistry of the gas in young disks, JWST will do the same for the solid material that forms the early building blocks of life. JWST will also enable us to image counterparts to our own asteroid belts around nearby stars for the first time. These debris disks are the remnants of planet formation and can serve as signposts for the unseen planets that have shaped these belts.
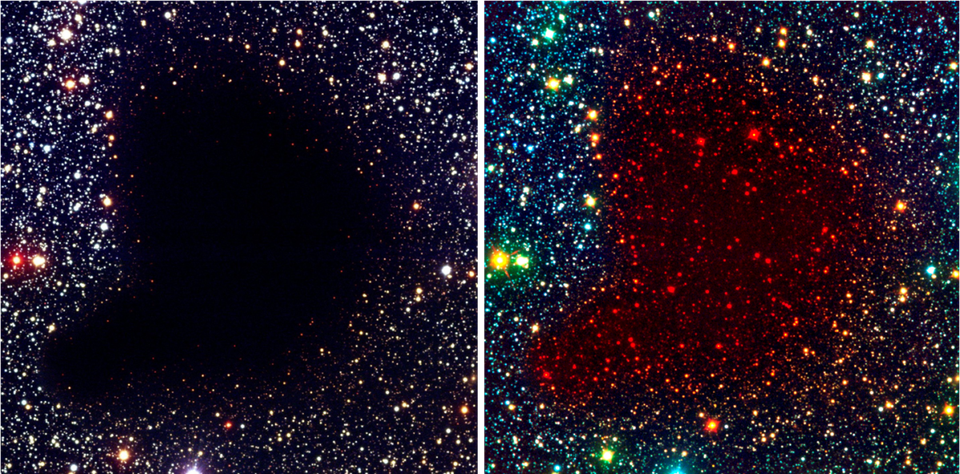
(Credit: ESO)
Q18.) A greatly underappreciated thing to study in the Universe is dust: to most astronomers, dust is a thing that’s “in the way” and needs to be subtracted out to see the objects that it obscures. But James Webb will be outstanding at teaching us, with both MIRI and NIRCam, the properties of dust in the interstellar medium, in galaxies, and more. What do we anticipate learning, and why is it so important in its own right?
Answer (Irene Shivaei): Dust grains are small (smaller than 100 microns) solid particles that are present everywhere in galaxies, from proto-planetary disks to birthclouds of stars and in the medium between the stars (called interstellar medium). These grains have crucial roles in the process of formation of stars that creates a visible galaxy. On the other hand, dust grains absorb the ultraviolet and optical light that is emitted from stars and reradiate it at longer (less energetic) wavelengths in the infrared. Therefore, dust determines how galaxies look like in the ultraviolet, optical (visible), and infrared light.
Altogether, these things make dust a very important component of galaxies, and studying its properties and observing it in the infrared are critical for understanding galaxy formation processes. The best way to study dust is by observing its emission in the infrared. The powerful infrared instruments of James Webb will enable us to look through dust clouds and study the characteristics of dust around stars and in the interstellar medium of nearby galaxies, as well as revealing dust-obscured star forming regions in galaxies 10 billion years ago in an unprecedented way that has not been possible with any other telescope.
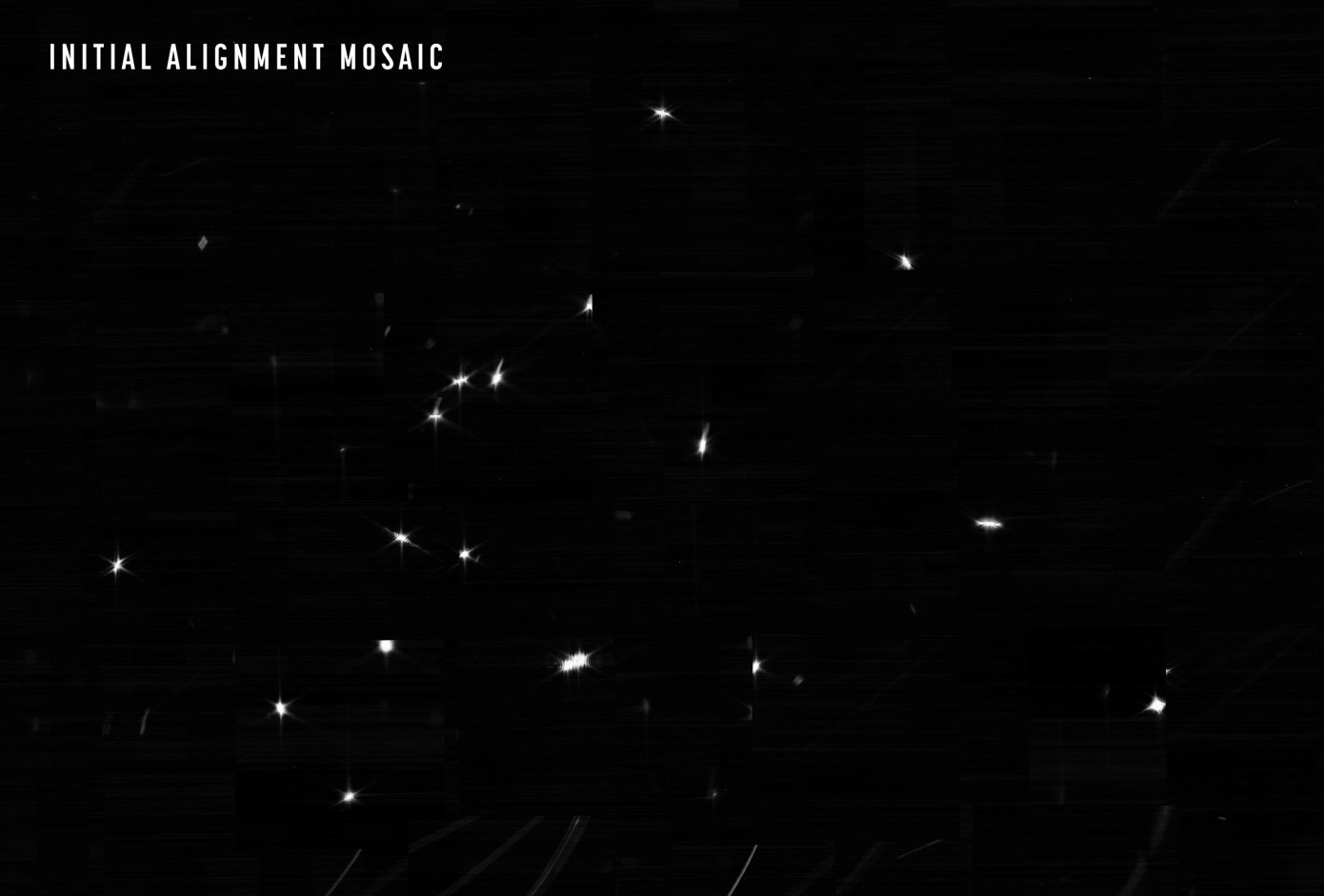
Q19.) There are some really subtle problems that your team has identified, but in doing so, you then come to understand how to better interpret your data. One of them has the best name I’ve seen so far: the “nightmare snowflake,” which refers to what’s known as Webb’s point-spread function. Can you explain to us what this is, and why understanding this “nightmare snowflake” is so important?
Answer (Jarron Leisenring): The point-spread function (PSF) is simply what a point of light looks like when imaged through an optical system. All stars are effectively point sources because they’re too distant and therefore small to spatially resolve. Because JWST’s primary mirror is not circular, but instead a 30-sided tricontagon, it creates an incredibly complicated PSF, the so-called “nightmare snowflake.”
In addition, this snowflake pattern can change in subtle ways that will affect our observations and processing of the data. For instance, the PSF changes slightly depending on the wavelength of an observation, the position in the field of view, and over time. Some of these changes can be minor and unimportant depending on the science case; but for coronagraphic observations, where you occult the central star to search for faint orbiting planets and disk, we want to subtract the stellar light and leave only the planetary objects. It is critical that we understand how the PSF evolves over time in order to identify the extremely faint signals of exoplanets and disks hiding within the broad stellar PSF.
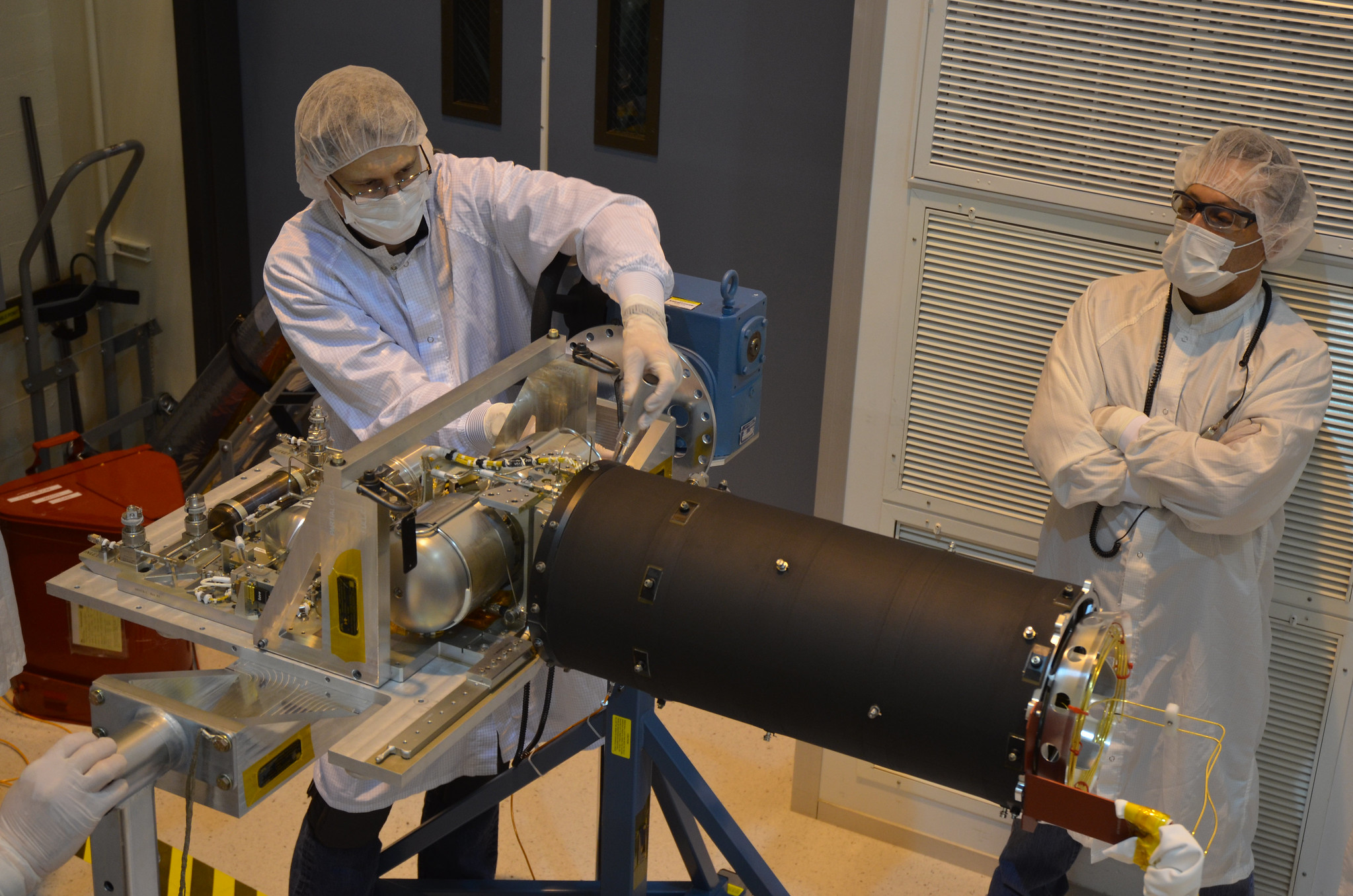
(Credit: NASA/JPL-Caltech)
Q20.) One of the most important endeavors many of you got to participate in was hands-on instrument testing, including multiple cryovac testing runs and making use of the “flight spare” of the NIRCam instrument. Why was this so important, and how will that inform the choices of observations that will be made, at least at the start, using James Webb’s capabilities?
Answer (Everett Schlawin): The “cryovac” tests, which suck the air out of a chamber and freeze it to -387 F are completely critical for confirming that the instruments will take pictures. The warmth of room temperature conditions as well as the electronic properties of the detectors mean we cannot see anything without a cryovac test. So we used a set of lamps inside the chamber to make pretend stars in the cameras. At NASA Johnson (home to many past, present and future human spaceflight endeavors), the entire optical train was used down to the 4 science instruments and fine guidance sensor. Our team also made sure that the wheels can rotate to change colors and tuned all the voltages and currents in the light sensors, heaters, motors, electronic “brains,” and components can function at their best. Without finding these settings, the images could potentially come out empty, full of “snow-like” noise or washed out.
The flight spares allowed us to set up a mini-NIRCam in our lab at the University of Arizona. This lets us try out new ideas or modes, test software and lower the noise as much as possible. Reducing the noise is critical to finding the faintest galaxies, resolving small details or measuring the tiny winks of light as planets go in front or behind their host stars.
Thanks to all nine of the professional astronomers, all members of the NIRCam and MIRI teams, who helped answer these questions: Everett Schlawin, Jarron Leisenring, Stacey Alberts, Andras Gaspar, Irene Shivaei, Thomas Beatty, Christina Williams, Schuyler Wolff, and Kevin Hainline. And thanks to you for reading all the way to the end!