10 planetary facts that extend beyond our Solar System
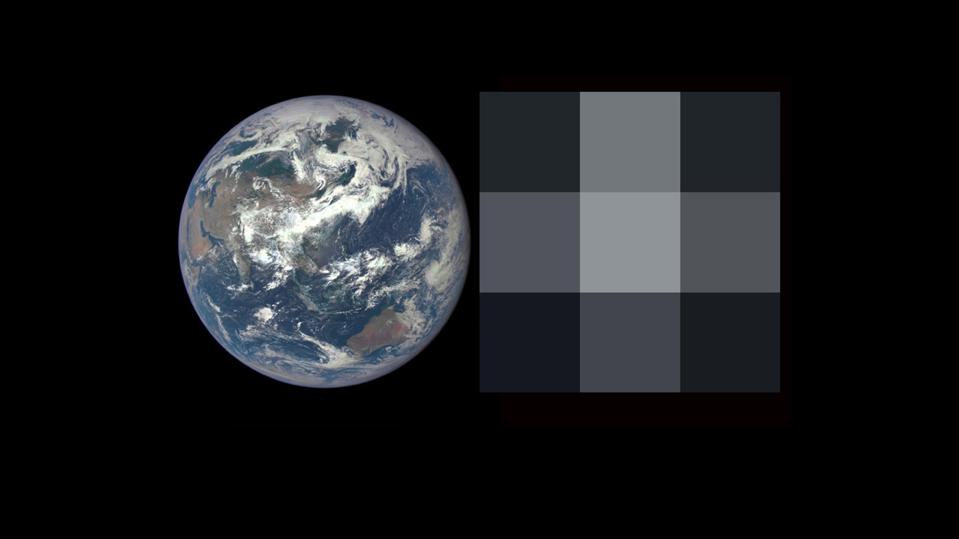
- Over the last ~30 years, our understanding of exoplanets, or planets beyond our own Solar System, has gone from purely hypothetical to an observationally rich field.
- With over 5000 exoplanets under our belt and several planet-rich systems having been directly imaged, we’ve learned so much that defied our initial expectations.
- Here are 10 facts that would blow even the most brilliant astronomer’s mind if you had presented them back in 1990. I bet they blow your mind, too.
It’s hard to imagine, but back in 1990 — the year that the Hubble Space Telescope was launched — we had yet to discover a single planet beyond the ones within our own Solar System. We were fairly certain they existed, but we didn’t know if they were rare, common, or everywhere. We didn’t know whether rocky planets or gas giants were “normal” planets, or whether there were other types that our own Solar System doesn’t have. And for better or for worse, we operated under the assumption that our Solar System was relatively typical, and that its structure, of inner, rocky planets, an asteroid belt, gas giants, and a Kuiper belt and Oort cloud beyond them would be the template for most, if not all, other planetary systems.
What a wild ride the last ~30 or so years have been, and how much they turned our assumptions on their heads. With over 5000 exoplanets now under our belts, and many other protoplanetary disks (where planets form) having been directly imaged, we now realize that much of what we initially thought was entirely too presumptive of us, and that nature is full of surprises. Here are 10 planetary facts that would’ve surprised practically every working astronomer back in 1990, and might still surprise you today!
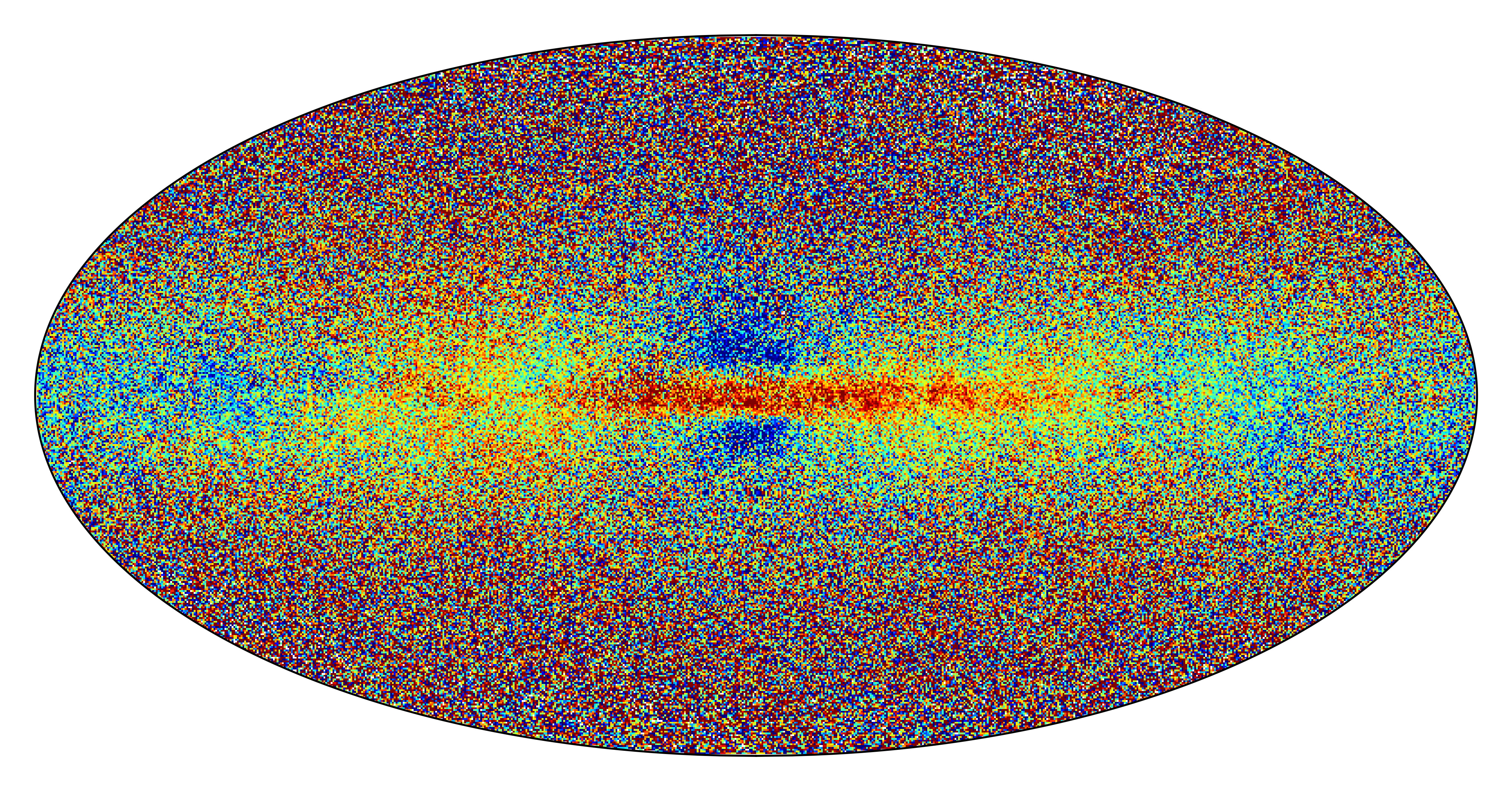
1.) Not every star can have them. One of the first surprises that awaited exoplanet scientists came when the Kepler mission first began examining a large field of over 100,000 stars, looking for planetary transits. When a planet passes in front of its parent star, it blocks a fraction of the star’s light. As multiple orbits and multiple transits build up, we can better pin down the orbital distance and physical size of the exoplanet. Initially, based on the number of stars we were looking at and the geometric chances of having a transit observable from our particular line-of-sight, it looked like perhaps ~100% of stars would have planets.
But it turns out that this isn’t the case. When we classify stars by metallicity, or the percentage of elements heavier than hydrogen-and-helium within the star, there’s a clear drop-off in planetary abundances. Practically all stars with 25% or more of the heavy elements found in the Sun have planets, only a fraction of stars with between 10-25% of the Sun’s heavy elements have planets, and only two or three stars with under 10% of the Sun’s heavy elements have planets at all. Unless you form from material that’s been sufficiently enriched by prior generations of stars, your star isn’t likely to have planets.
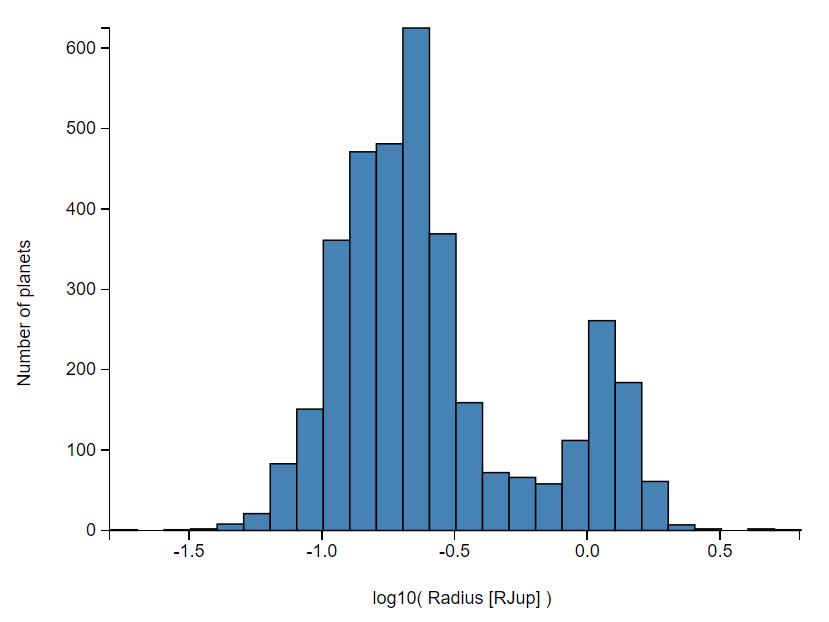
2.) Super-Neptunes (or Mini-Saturns) are rare. We knew, from our own Solar System, that gas giant planets came in at least two different sizes: about four times Earth’s radius, like Neptune and Uranus, and about ten times Earth’s radius, like Jupiter and Saturn. But what else would we find? Would these sizes of worlds be common or rare? Would there be large numbers of gas giant planets found with properties unlike those found in our Solar System, like super-Jupiters, “tweeners” that were in between Neptune and Saturn in size, or mini-Neptunes?
It turns out that both Jupiter-sized and Neptune-sized planets are very common, with mini-Neptunes also being even more common than Neptunian worlds. But in between the sizes of Neptune and Saturn are very few planets at all, suggesting that there’s some physical reason why planets tend to avoid forming with sizes between 5-and-9 Earth radii. That reason is still under investigation, but it’s fantastic to know that Neptunes and Jupiters are common, while in-between worlds aren’t!

3.) Ultra-distant gas giants are fairly common. Here in our own Solar System, there’s a big “cliff” out beyond 30 times the Earth-Sun distance, or 30 astronomical units (AU). We have eight major planets interior to that distance, but none that are even as large as the smallest planet, Mercury, beyond that distance.
But around many stars, there are giant planets located a great distance away: 50 AU, 100 AU, or even several hundred AU away from the main star in their system. Some of these planets are so large that their cores exceed 1 million K in temperature, enabling them to fuse deuterium and become brown dwarfs, while others fall below that mass threshold and instead only generate infrared light, similar to Jupiter.
These systems, like HR 8799 (above), are some of the best systems for direct imaging, and have revealed to us many directly imaged exoplanets thus far.
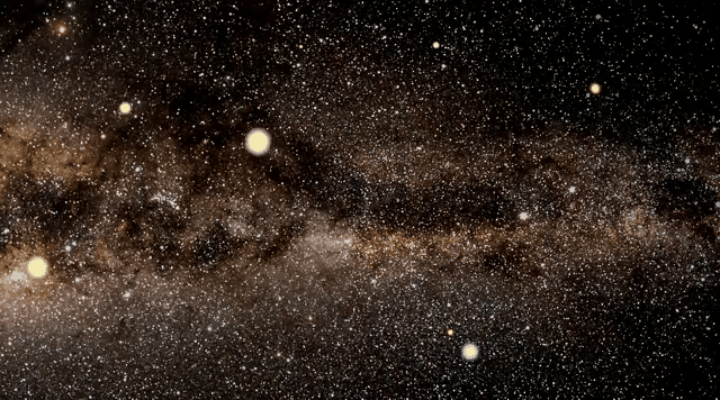
4.) Many planets are orphans, without a parent star. In this Universe, what you see isn’t what you get; it’s only representative of the fraction of what you got that survived until the present day. This is true in our Solar System, where many now think that there was a 5th gas giant in our early history that got ejected long ago, and it’s true elsewhere throughout the Universe as well. Some planets remain with their parent stars, others are ejected and roam the Universe as orphan (or rogue) planets, and others very likely come into existence in star-forming regions around clumps of matter that were too low in mass to form a star.
Fortunately, a novel method has begun to reveal these rogue planets: gravitational microlensing. As these planets travel through the galaxy, they’ll inevitably pass through our line-of-sight to one or more stars, and when they do, their gravity will bend, distort, and temporarily magnify the light from one of those co-aligned stars. That characteristic microlensing signal has been observed several times, revealing these otherwise invisible orphan planets. With improved observatories and greater wide-field continuous imaging, microlensing may someday reveal more total exoplanets than all other methods combined.

5.) Ultra-hot planets are the easiest to detect. When it comes to our Solar System, Mercury is the closest planet to our Sun, with an orbit of just 88 days and a maximum daytime temperature of over 800 °F (427 °C). But some of the exoplanets that we’ve found have temperatures of several thousands of degrees, and orbit their parent stars in only a handful of days or even in a matter of hours.
It turns out there’s good reason for this: the two methods we use, the radial velocity method (where we measure the “wobble” of a star due to the gravitational effects of an orbiting planet) and the transit method (where we measure the periodic dimming of the parent star as the orbiting planet blocks its light) are both biased toward planets that orbit extremely close to their parent stars.
While the first discovered exoplanets were hot and massive, we’ve now discovered a great number of planets of all masses that are very close to their parent stars. That’s not because they’re super common, but because fast-moving planets lead to more dramatic changes in their parent star’s motion and allow us to observe greater numbers of transits in the same amount of observing time. It’s not worth taking a second look at the stars we’ve monitored for evidence of additional hot planets; we’ve probably already seen most of them in the fields of view where we’ve looked.
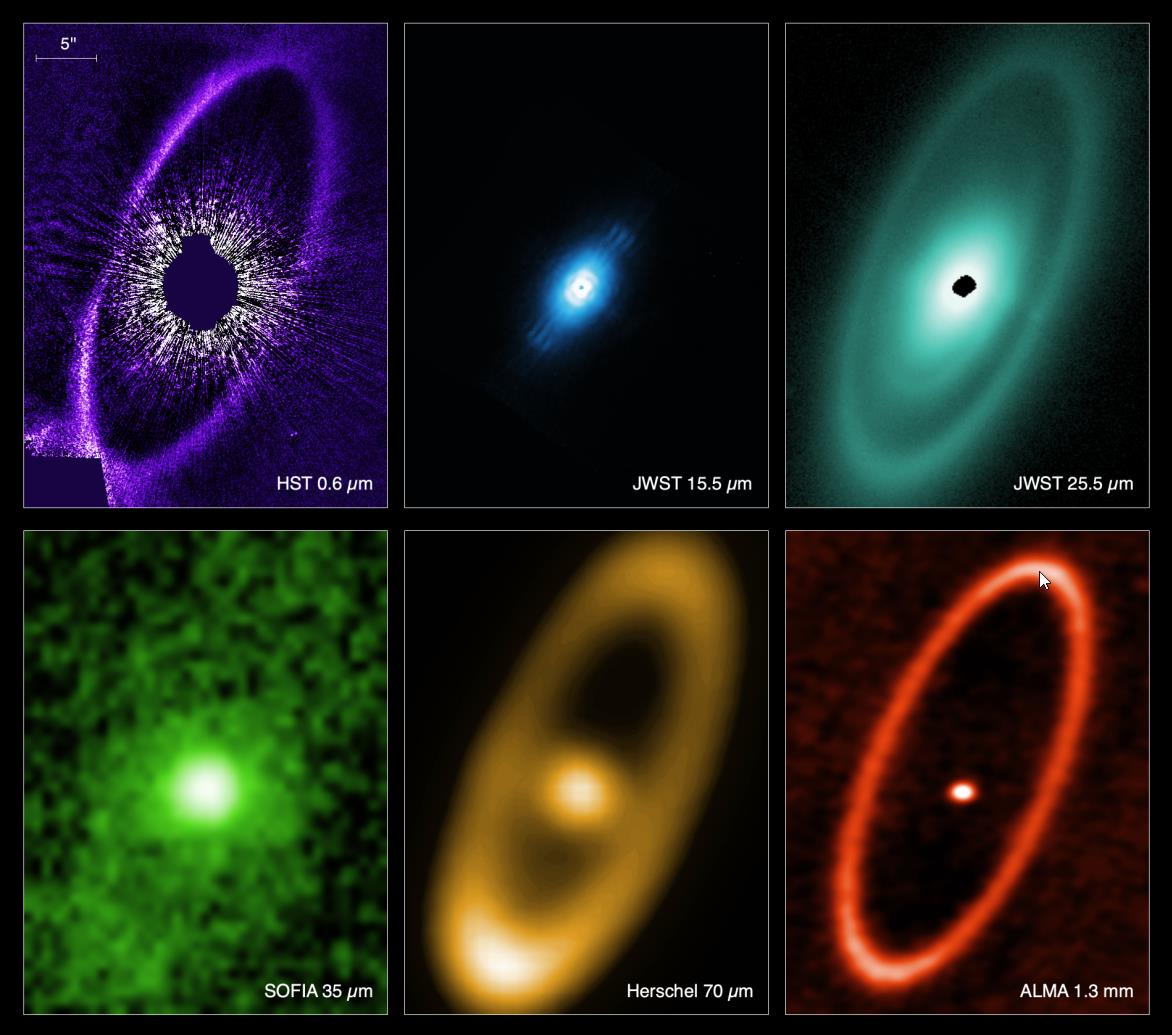
6.) Long after the planet-forming gas is gone, dusty debris remains. This one was a bit of a puzzle that’s only been unveiled extremely recently. We’ve known for a long time that planet-formation occurs very quickly, and is only possible as long as gas remains around a young star. Once that protoplanetary disk evaporates, planet-formation is complete. Dust, on the other hand, is produced whenever two bodies collide, and can be caused by comet storms, asteroid collisions with one another or with rocky bodies, or several other violent events.
But while the gas is all gone after only perhaps 10-20 million years around a newly formed star, the dust can persist for several hundred million years (and perhaps even a billion or more) all throughout stellar systems. While a number of systems have exhibited dust within the analogue of their Kuiper belts, recent observations have shown some big surprises, including:
- dust found all throughout the inner disk-like region of a stellar system,
- an intermediate ring of dust between the asteroid belt-like and Kuiper belt-like regions of a stellar system,
- and systems with up to hundreds of times the amount of dust present in our own Solar System.
These clues add up to a tantalizing possibility: maybe our own Solar System, during the early bombardment period, was once a dust-rich system, too.
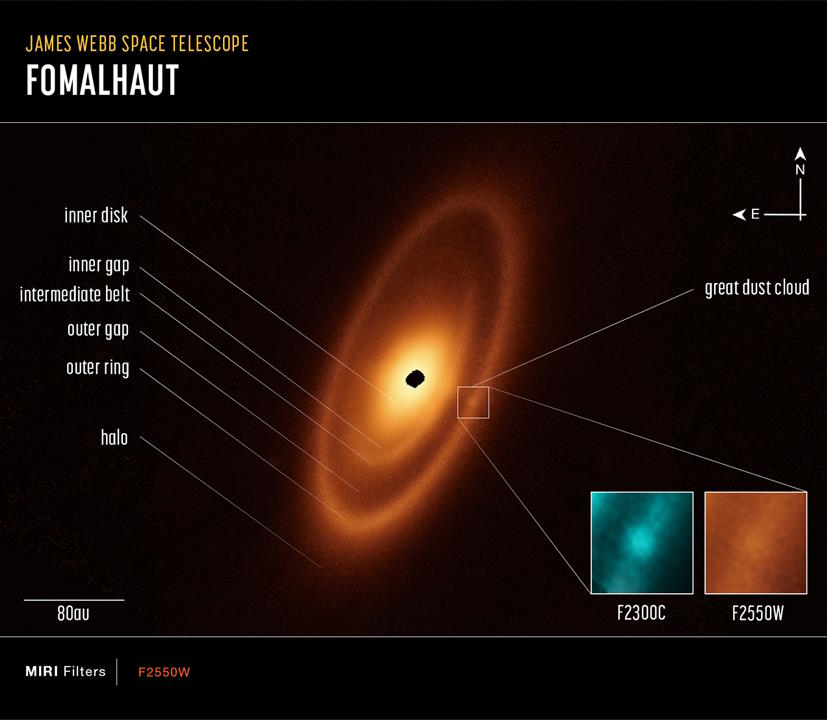
7.) Asteroid belts and Kuiper belts are just the tip of the iceberg. We initially thought that an asteroid belt and a Kuiper belt would make sense, and might even be universal properties for stellar systems. After all, the different types of ices that form in space all have their own melting/boiling/sublimation points, and that creates a series of what are known as “frost lines,” or places on the border of where ice of a specific species (water-ice, dry ice, methane ice, nitrogen ice, etc.) can or cannot exist around a star. These lines should correspond to where a belt of asteroids forms, in between any interior and exterior planets.
Similarly, there should be a collection of small planetesimals left over out beyond the final planet in a system: a Kuiper belt. So why, as we just observed around Fomalhaut, are we seeing a third belt at intermediate distances? Are there other systems that have more than a Kuiper belt and an asteroid belt, and what sort of physical formation mechanisms drive them into existence? Is our Solar System even common in this regard, or are multiple (perhaps even more than three) belts the norm? We’re truly right at the scientific frontiers here, and this is one discovery that was entirely unexpected.
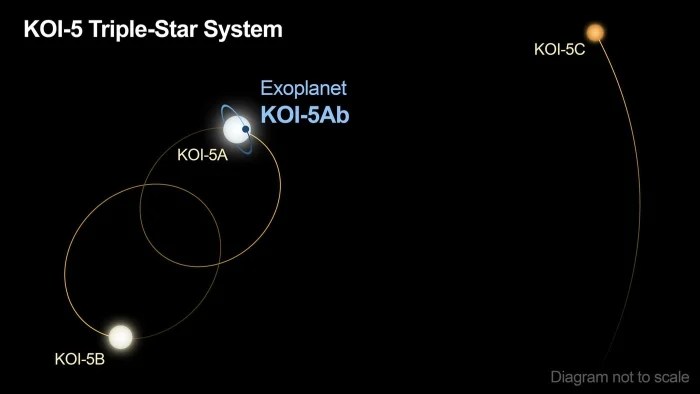
8.) Multi-star systems can have planets nearly as easily as singlet stars. For a long time, the idea of a Tatooine-like system, where a planet would observe multiple Sun-like stars in their daytime sky, was treated as a physical impossibility. The rationale was that the gravitational three-body problem would render any planet that orbited with multiple large masses nearby would eventually be ejected, rendering such systems what we call in the physics community “dynamically unstable.”
And while this is technically true, the timescale for that instability can be several tens of billions of years: longer than the age of the Universe. For every pair of orbiting stars, there are three regions that are quasi-stable:
- close in orbit around the primary (larger mass) star,
- close in orbit around the secondary (lower mass) star,
- or far away from the center-of-mass of both stars.
We’ve now found exoplanets that fall into all three of these categories, leading to the understanding that except for a few gravitationally unstable regions set by the relative masses and distances between the stars in a single system, there are plenty of places where planets can stably orbit over the lifetime of a stellar system. In time, we may yet find that the same percentage of multi-star systems are home to planets as singlet-star systems are.
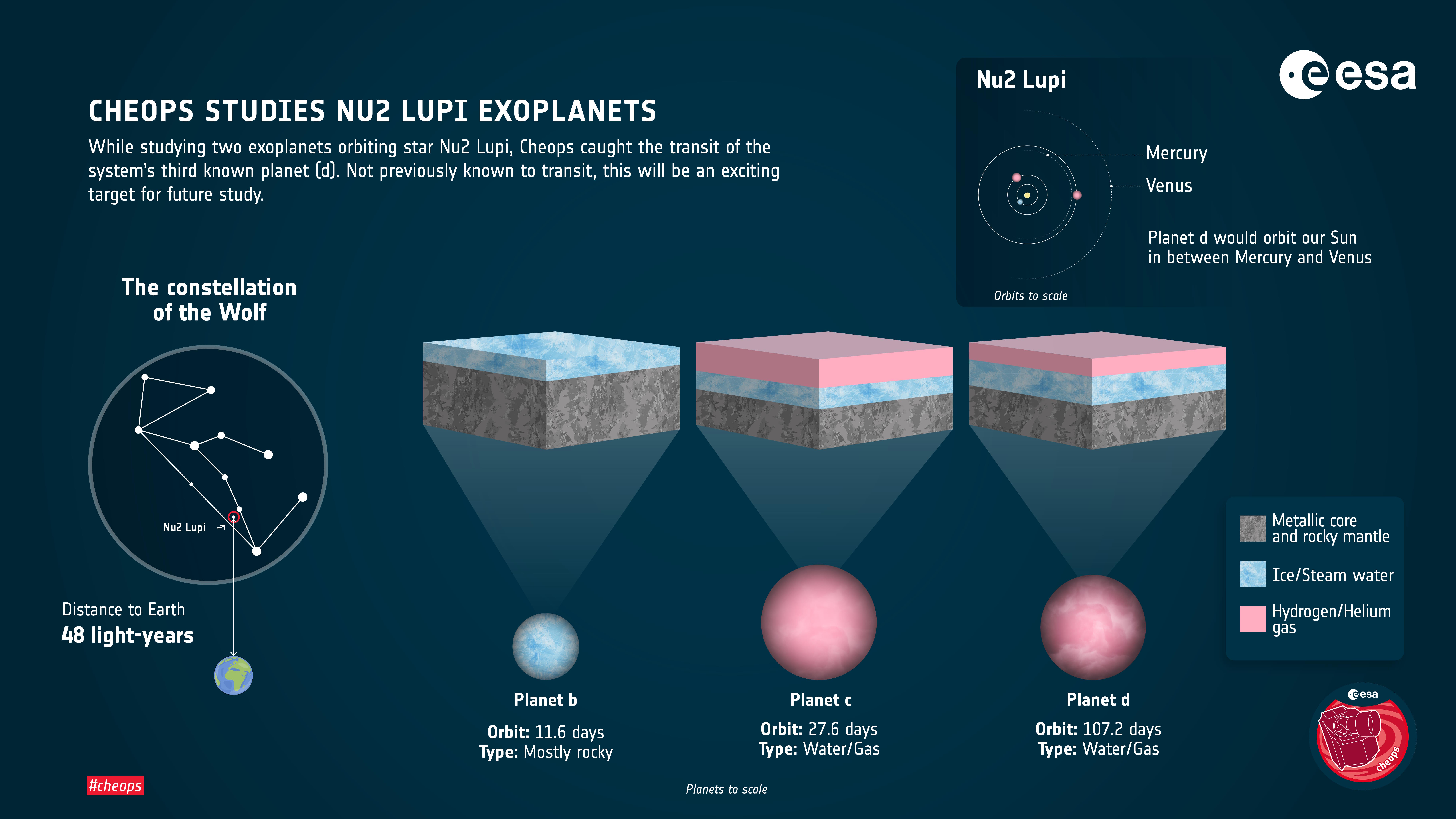
9.) You can only be slightly more massive than Earth and still be rocky and life-friendly. We really jumped to a premature conclusion the first time we discovered an exoplanet with a mass-and-radius that was larger than Earth’s but smaller than Neptune’s: we called them super-Earth worlds. While that’s a tempting way to think about these worlds, it should be equally tempting to think of them as mini-Neptunes, as our simple methods of exoplanet detection have not yet reached the sensitivity to measure and characterize the atmospheres of these worlds. If they’re thin and have rocky surfaces, we’d expect them to be Earth-like; if they’re thick and have large, volatile gas envelopes before you ever reach a solid surface, we’d expect them to be Neptune-like.
As measurements of the combination of exoplanet mass, exoplanet radius, and the exoplanet temperature (based on distance from its primary parent star) show, you can only be about ~30% larger and about ~2x as massive as Earth before you transition into a Neptune-like world, as it becomes very easy to hold onto volatile gases with only a little bit more mass than a planet like Earth has. There are exceptions to this general rule, but the exceptions are largely found among very hot worlds whose volatiles are easily boiled and evaporated away. All the while that we’ve been wondering where our Solar System’s “super-Earths” are, the answer has been right under our noses: we are nearly as “super” as an Earth-like planet can get.
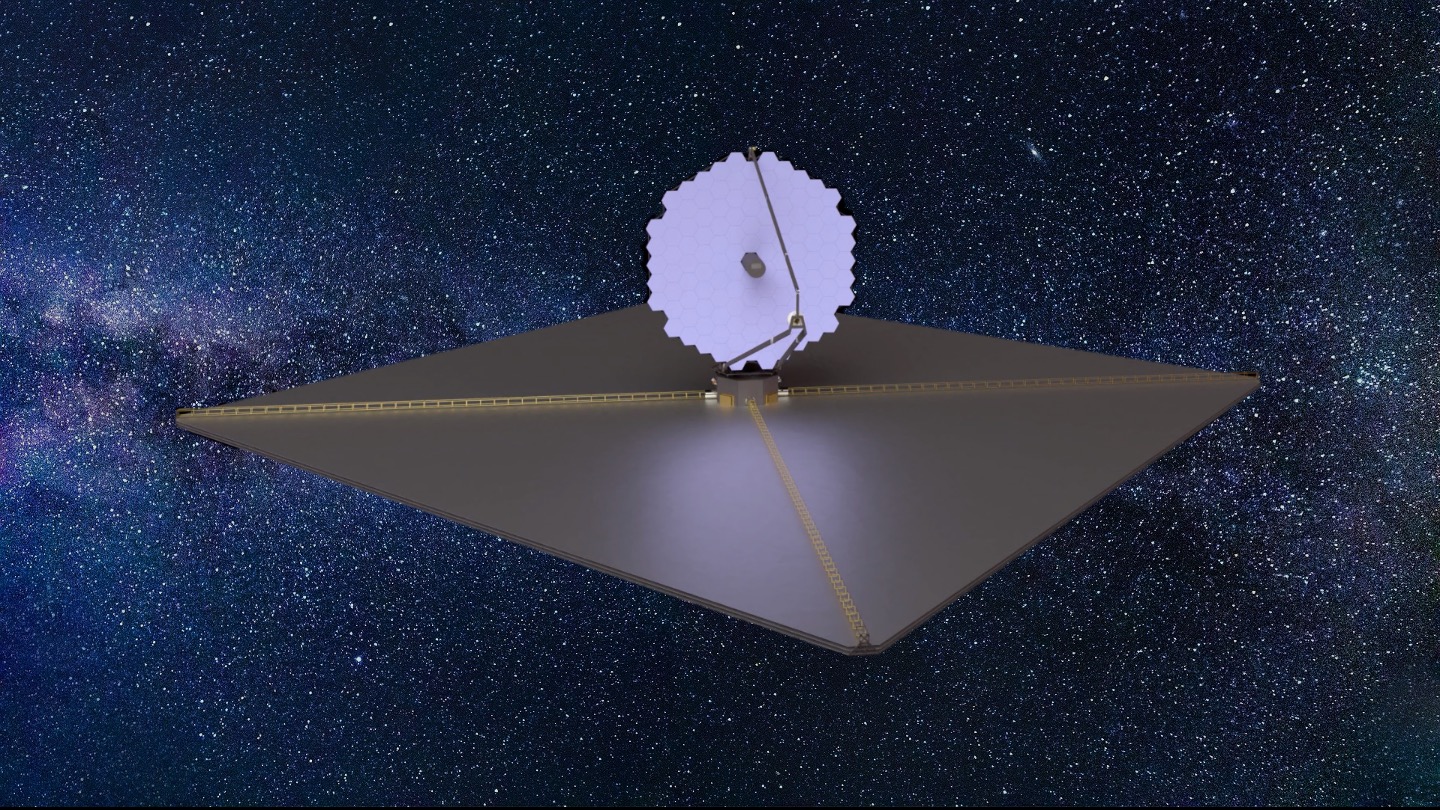
10.) The exoplanet holy grail, of directly imaging Earth-sized planets in the so-called Habitable Zone, is finally within reach. This is a big one, and it’s finally coming. We’ve often dreamed of what an appropriately advanced alien civilization would see if they looked at Earth from afar, and how they’d tell our planet is inhabited. As the planet rotated on its axis, they would see evidence for clouds, oceans, and variable continents. As the seasons changed, they would see icecaps grow and retreat while the continents greened and browned. And if they could measure our atmospheric contents, they would see gas levels change in a way that indicated that we were not only an inhabited world, but that a technologically advanced species lived here.
With the upcoming NASA flagship mission in either the 2030s or 2040s known as Habitable Worlds Observatory headed our way, we’re going to meet that goal: not for Earth, but for any Earth-like planets that happen to be located around the ~20 or so nearest star systems to our own. The combination of having a space-based telescope that’s sufficiently large, with sufficiently advanced instruments, and with an unprecedentedly efficient coronagraph can finally reveal the nearest rocky worlds to us directly, and measure their atmospheres for signs of life, including intelligent life. The great dream of 20th century astronomers will come to fruition in just another 15-20 years, and humanity just might reap the ultimate rewards: getting an affirmative answer to the question of “Are we alone in the Universe?”