The dark side of neuroplasticity
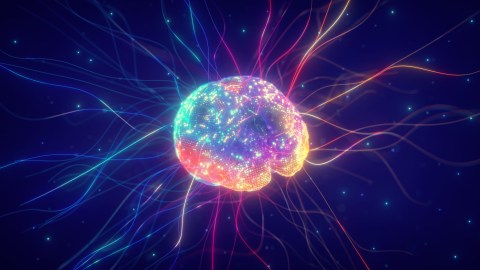
- Neuroplasticity refers to the brain’s ability to reorganize its structure and function in response to new experiences.
- Many believe that harnessing the power of neuroplasticity could lead to treatments or cures to afflictions ranging from depression to paralysis.
- However, neuroplasticity has a dark side: addiction and, as a new study shows, epilepsy.
In 1913, Santiago Ramón y Cajal, the father of modern neuroscience, stated, “In the adult… the nerve paths are something fixed, ended, and immutable. Everything may die, nothing may be regenerated.” This quickly became a central dogma of neuroscience, which persisted for decades. In the 1960s, however, evidence for what we now call neuroplasticity began to emerge: Research showed that neurons can alter their structure and function, and that the brains of various species, including mammals, can grow new cells in adulthood.
It was not until the 1990s, with studies showing that the adult human brain generates new cells, that the dogma was overturned. Today, it is widely believed that neurplasticity is the rule rather than the exception, and that every experience we have alters the structure or function of the brain in one way or another. Brain plasticity is often touted as a miracle cure, but it does have a dark side. Addiction, for example, occurs as a result of neuroplasticity within the brain’s reward system. Now, an animal study by a team of researchers from Stanford University shows that a newly described form of plasticity likely contributes to the progression of epilepsy.
A primer on neuroplasticity
The most widely studied form of neuroplasticity occurs at synapses, the junctions between neurons, at which the cells transmit chemical signals to each other. Synaptic plasticity involves the strengthening or weakening of the signaling process, in response to increased or decreased neuronal activity, making signaling within that pathway more or less efficient. Synaptic plasticity is widely believed to be crucial for learning and memory formation. Addiction can be thought of as a maladaptive form of synaptic plasticity involving the modification of connections in the dopamine pathways, which play a key role in reward processing, leading to powerful and long-lasting memories of drug experiences.
Another widely studied form of plasticity is adult neurogenesis, or the formation of new nerve cells. This occurs in several regions of the human brain, most notably the hippocampus, which plays important roles in learning, memory, and spatial navigation. There is, however, an on-going debate about the significance of this process. Studies provide conflicting evidence about the numbers of new cells formed in the hippocampus, and it is still unclear what role, if any, the newly formed cells have in brain function.
More recently, a hitherto unknown form of neuroplasticity, has been discovered. This involves the redistribution of myelin, a fatty tissue that insulates nerve fibers and increases the velocity of the electrical impulses they carry. In the brain and spinal cord, myelin is produced by non-neuronal cells called oligodendrocytes. Due to its high fat content, it appears white under the microscope — hence the terms “white matter” (brain areas enriched in myelin) and “white matter tracts” (bundles of nerve fibers for long distance communication.)
In humans, white matter formation occurs extensively throughout childhood and continues well into the second decade of life. Once myelination is complete, it was believed that the distribution of white matter remained stable. But this is not the case. Using a brain scanning technique called diffusion tensor imaging to visualize white matter tracts in the human brain, researchers have shown, for example, that learning a complex motor skill such as juggling or playing the piano induces changes in the brain’s white matter architecture, and animal experiments show that blocking the formation of new oligodendrocytes impairs memory consolidation.
Naughty neuroplasticity
The new study, led by Juliet Knowles, was performed on an inbred strain of rats that grow to develop spontaneous ”absence” seizures (which involve a lapse in consciousness) similar to those in humans. In these animals, the seizures originate in cells connecting the cerebral cortex to a subcortical structure called the thalamus and spread through the brain via white matter tracts connecting these regions, as well as through the corpus callosum, a huge white matter bundle connecting the two hemispheres.
Knowles and her colleagues examined the brains of these animals before and after they developed seizures and compared them to those of healthy control rats. They found that the number of oligodendrocytes, and the extent of myelination, in the corpus callosum was greater in the epileptic rats after the onset of seizures and increased in parallel with the progression of the seizures. Brain regions that were unaffected by the seizures did not exhibit these differences.
As well as exhibiting a 69% increase in the number of immature oligodendrocytes and a 56% increase in mature cells, the rats also had an abnormal myelin structure, with the myelin sheaths around axon fibers being thicker than those in the control rats. Rats treated with the anti-convulsant drug ethosuximide, however, had fewer seizures, or none at all, and their myelin structure was comparable to that seen in the controls.
The team also used the Cre-LoxP system to breed a seizure-prone, genetically engineered strain of mice that could allow the researchers to delete a cell surface receptor called TrkB from immature oligodendrocytes at any stage by treatment with the drug tamoxifen. During normal development, active neurons secrete a growth factor called brain-derived neurotrophic factor (BDNF), which binds TrkB on immature oligodendrocytes to induce myelination of axons projecting from the corpus callosum to the cortex. These mice developed seizures at around three months of age and exhibited the same abnormalities as the epileptic rats, but deletion of TrkB from immature oligodendrocytes prevented the abnormal increase in myelination and significantly reduced the number of seizures they had.
The results, published in Nature Neuroscience, show that the electrical activity associated with epileptic seizures increased both the proliferation of immature oligodendrocytes and the number of mature oligodendrocytes in the corpus callosum, leading to abnormal overproduction of myelin that in turn promoted the progression of epilepsy.
From mice to men
It is, however, too soon to directly extrapolate the findings to epilepsy in humans. Epilepsy takes various forms in humans, which differ in cause, age of onset, and location and severity of seizures, and so the role of myelin plasticity is also likely to differ between each form. Nevertheless, further investigation of maladaptive myelination may eventually lead to novel strategies for treating epilepsy and other neurological conditions.