Scientists watch a crystal being born
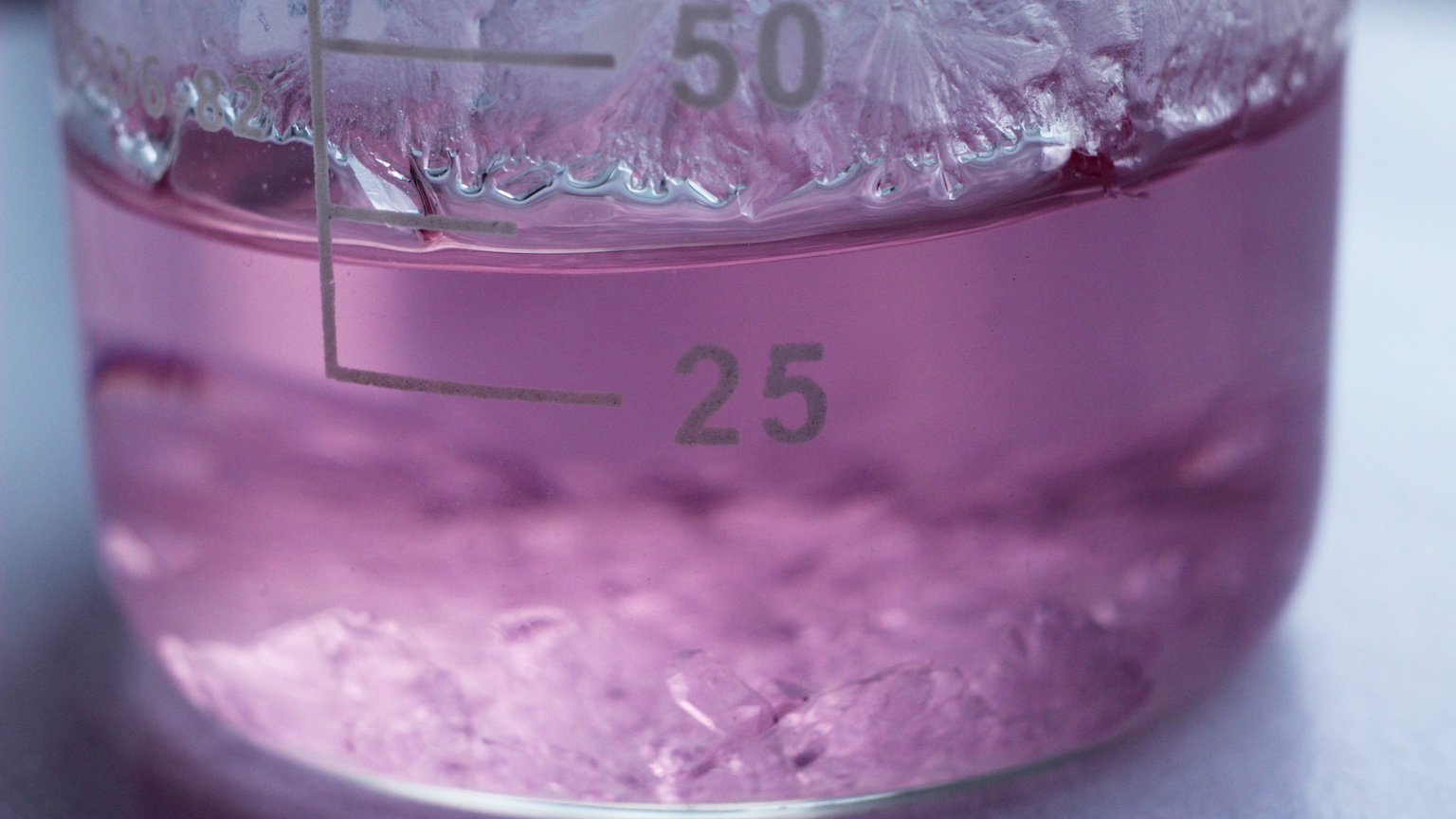
- As crystals begin to form, atoms and molecules will often coalesce, or “nucleate,” at unpredictable times and locations, making it difficult for researchers to study the process on a molecular level.
- A new technique can manually trigger crystal nucleation, while simultaneously probing the coalescing molecules involved.
- This could help researchers understand the origins of crystal structures in far more detail.
On molecular scales, many solid materials display an orderly arrangement of atoms and molecules. Yet in many cases, these orderly crystal structures grow out of far more disorderly forms of matter, such as liquids or solids dissolved in solution (think: salt water).
Under the right conditions, crystals will begin to form as small groups of atoms and molecules cluster together, forming tiny, solid “nuclei.” Over time, atoms and molecules surrounding the newly formed nucleus will attach themselves to it, until the whole system has arranged itself into an orderly crystal lattice. This process plays a vital role in nature and underlies the formation of materials including ice, coral, and volcanic rock.
By triggering nucleation in controlled conditions, researchers have engineered many materials that are now used in cutting-edge technologies.
The crystallization conundrum
Despite its importance across a diverse array of processes, there is still much that researchers don’t understand about how crystal nucleation unfolds. Today, the most advanced imaging techniques allow researchers to study samples at the atomic level and observe how they evolve over time.
Yet, imaging is still difficult because nucleation is a “stochastic” process, meaning the times and places where nuclei will begin to form are essentially random. If researchers don’t know where to look, experiments only can provide us with limited details about the molecular mechanisms involved. Theorists try to fill in the gaps, but there is a mismatch between theory and experimental data — for instance, involving the rate at which nuclei will form under certain conditions.
Single crystal nucleation spectroscopy
To tackle the challenge, Takuji Adachi at the University of Geneva, together with colleagues at McGill University in Montreal, suggest a new approach, involving a combination of two widely used techniques. Their report is published in the journal PNAS.
The first of these is “optical trapping,” which utilizes laser beams to confine single atoms or molecules in fixed positions or move them precisely to pre-determined locations — just like a tiny pair of tweezers. The second technique is “Raman spectroscopy,” in which a laser is fired at a molecular sample. As the photons contained in the laser light interact with vibrating atoms and molecules, they will either gain or lose energy in a way that is unique to the molecules contained in the sample. In other words, the sample produces a molecular “fingerprint,” allowing scientists to determine its composition.
Using these two techniques in tandem, Adachi’s team created a new technique, which they call “single crystal nucleation spectroscopy” (SCNS). In their experiment, they used a near-infrared laser to confine a single molecule of glycine in a water solution. (Glycine is the simplest amino acid, the building blocks of proteins.) As had been shown in previous studies, optical tweezing generates a force that attracts other glycine molecules surrounding the one being confined. Subsequently, the glycine molecules coalesced into a newly formed nucleus.
Simultaneously, the researchers probed the nucleus using a Raman laser beam. By closely monitoring energy shifts in the photons emerging from the nucleus, they could visualize how its crystal structure evolved over time. Based on their Raman spectra of nucleating glycine, Adachi’s team proposed that these orderly crystal structures emerged out of linear networks of molecules, linked together by hydrogen bonds.
This result could be an important first step toward experiments that can put our existing theories of crystal nucleation to the test. This would allow researchers to better understand the conditions that allow nucleation to occur most readily in particular liquids and solutions.
Practical crystallography
In turn, Adachi’s team hopes that SCNS will help researchers engineer purer, more stable crystal structures. This could lead to whole new classes of materials, better suited for advanced technologies, or perhaps the discovery of new drugs, engineered to target specific medical conditions with unprecedented precision.