The hunt for the ‘angel particle’ continues
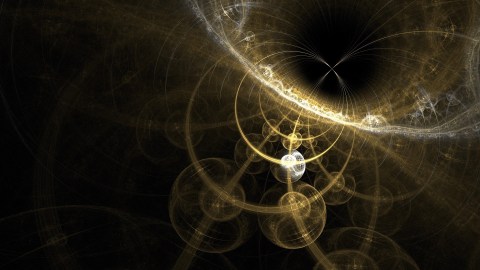
Pixabay
- In 2017, researchers believed they had found evidence for the so-called “angel particle”; that is, a Majorana fermion.
- Majorana fermions differ from regular fermions in that they are their own antiparticles.
- New research shows that the previous finding was due to an error in the scientists’ experimental device. Thus, it’s back to the drawing board in the search for the Majorana fermion.
A theoretical class of particles called Majorana fermions remains a mystery. In 2017, scientists believed they had uncovered evidence for the existence Majorana fermions. Unfortunately, recent research shows that their findings were actually due to a faulty experimental device, bringing researchers back to the drawing board in the search for the exotic particles.
What are Majorana fermions?
The Standard Model of particle physics currently is our best means of explaining the fundamental forces of the universe. It classifies the various elementary particles, like photons, the Higgs boson, and the various quarks and leptons. Broadly, its particles are divided into two classes: Bosons, like the photon and Higgs, and fermions, which comprise the quarks and leptons.
There are a few major differences between these types of particles. One, for instance, is that fermions have antiparticles, while bosons do not. There can be an anti-electron (i.e., a positron), but there’s no such thing as an antiphoton. Fermions also can’t occupy the same quantum state; for instance, electrons orbiting an atom’s nucleus can’t both occupy the same orbital level and spin in the same direction — two electrons can hang out in the same orbital and spin in opposite directions because this represents a different quantum state. Bosons, on the other hand, don’t have this problem.
But back in 1937, a physicist named Ettore Majorana discovered that there a different, unusual kind of fermion could exist; the so-called Majorana fermion.
All the fermions in the Standard Model are referred to as Dirac fermions. Where they and Majorana fermions differ is that the Majorana fermion would be its own antiparticle. Because of this quirk, the Majorana fermion has been nicknamed the “angel particle” after the Dan Brown novel “Angels and Demons,” whose plot involved a matter/anti-matter bomb.
Majorana fermions are predicted to appear in devices where a superconductor is affixed on top of a topological insulator (also referred to as a quantum anomalous Hall insulator [QAH]; left panel). Experiments performed at Penn State and the University of Würzburg in Germany show that the small superconductor strip used in the proposed device creates an electrical short, preventing the detection of Majoranas (right panel).
Cui-zu Chang, Penn State
A “smoking gun”?
Until 2017, however, there remained no definitive experimental evidence for Majorana fermions. But during that year, physicists constructed a complicated experimental device involving a superconductor, a topological insulator — which conducts electricity along its edges but not through its center — and a magnet. The researchers observed that in addition to electrons flowing along the edge of the topological insulator, this device also showed signs of producing Majorana quasiparticles.
Quasiparticles are an important tool that physicists use when searching for evidence of “real” particles. They aren’t the real thing themselves, but they can be thought of as disturbances in a medium that represent a real particle. You can think of them like bubbles in a Coca Cola — a bubble itself isn’t an independent object, but rather a phenomenon that emerges from the interaction between carbon dioxide and the Coca Cola. If we were to say there was some hypothetical “bubble particle” that really existed, we could measure the “quasi”-bubbles in a Coca Cola to learn more about its characteristics and provide evidence for this imaginary particle’s existence.
By observing quasiparticles with properties that matched theoretical predictions of Majorana fermions, the researchers believed that they had found a smoking gun that proved these peculiar particles really existed.
Regrettably, recent research showed that this finding was in error. The device that the 2017 researchers used was only supposed to generate signs of Majorana quasiparticles when exposed to a precise magnetic field. But new researchers from Penn State and the University of Wurzburg found that these signs emerged whenever a superconductor and topological insulator were combined regardless of the magnetic field. The superconductor, it turns out, acted as an electrical short in this system, resulting in a measurement that looked right, but was really just a false alarm. Since the magnetic field wasn’t contributing to this signal, the measurements didn’t match theory.
“This is an excellent illustration of how science should work,” said one of the researchers. “Extraordinary claims of discovery need to be carefully examined and reproduced. All of our postdocs and students worked really hard to make sure they carried out very rigorous tests of the past claims. We are also making sure that all of our data and methods are shared transparently with the community so that our results can be critically evaluated by interested colleagues.”
Why does this matter?
Beyond the intrinsic value of better understanding the nature of our universe, Majorana fermions could be put to serious practical use. They could lead to the development of what’s known as a topological quantum computer.
A regular quantum computer is prone to decoherence — essentially, this is the loss of information to the environment. But Majorana fermions have a unique property when applied in quantum computers. Two of these fermions can store a single qubit (the quantum computer’s equivalent of a bit) of information, as opposed to a regular quantum computer where a single qubit of information is stored in a single quantum particle. Thus, if environmental noise disturbs one Majorana fermion, its associated particle would still store the information, preventing decoherence.
To make this a reality, researchers are still persistently searching for the angel particle. As promising as the 2017 research appeared, it looks like the hunt continues.