Ask Ethan: Could the ‘Breakthrough Starshot’ project even survive its planned journey?
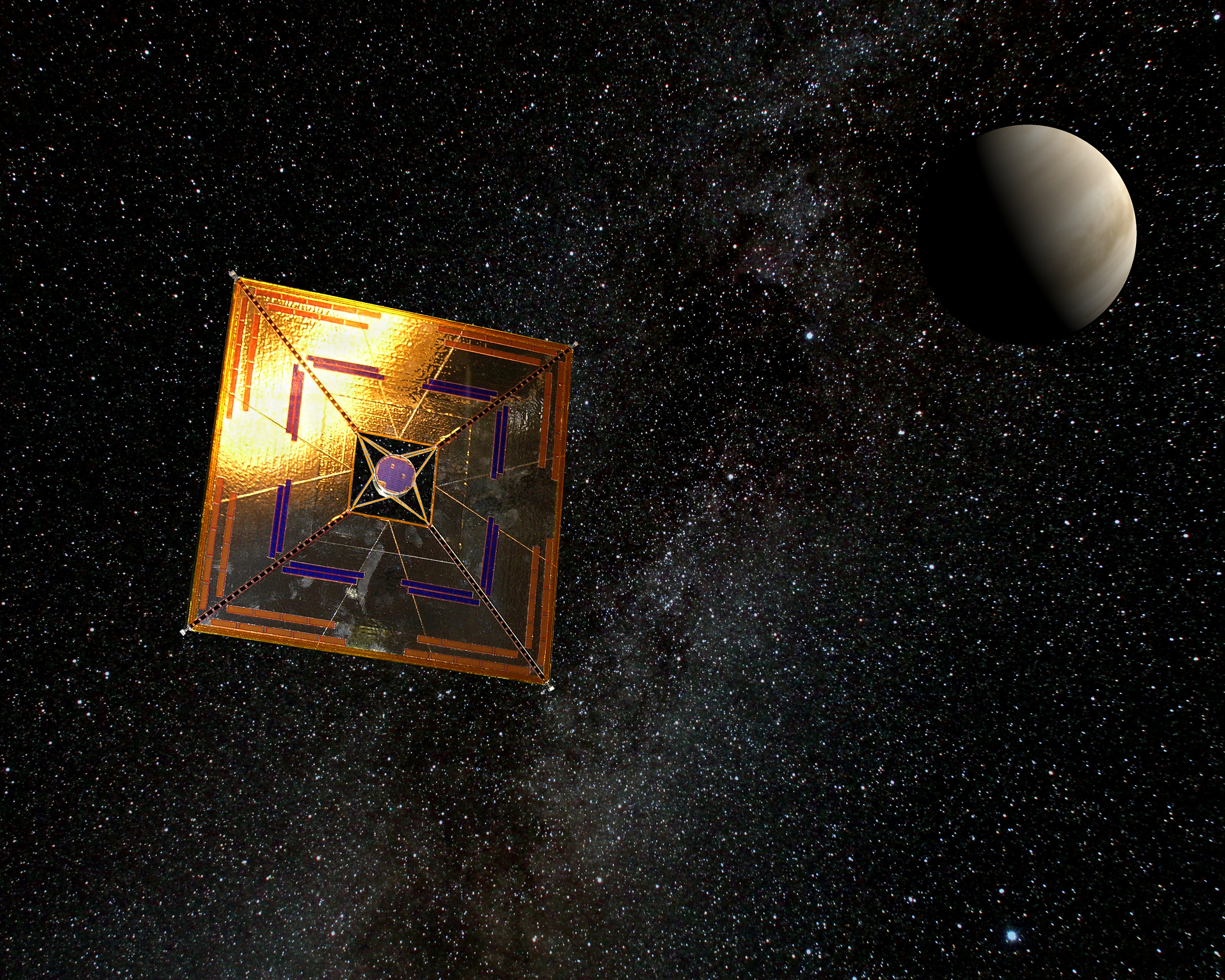
- Breakthrough Starshot is an innovative project aimed at accelerating tiny spacecraft approaching the speed of light, sending them on interstellar journeys.
- But at such speeds, the spacecraft itself would be subject to catastrophic collisions with the particles in the interstellar medium, casting doubt on its viability.
- Although workarounds are possible in principle, the physical limitations the project faces are formidable, and we have a long way to go in overcoming them.
For all of human history, embarking upon an interstellar journey has been a seemingly unreachable dream, made practically impossible by the enormous distances separating our Sun from any of our stellar neighbors. Even with the most powerful rocket technology ever developed, it would take tens of thousands of years to journey to the nearest star outside of our Solar System. Even the fastest spacecraft ever launched from Earth — like the Voyager, Pioneer, and New Horizons missions — only move at a few tens of kilometers per second on their way out of the Solar System, meaning a journey of a few light-years will take a thousand human lifetimes to complete.
But recently, a clever idea that leverages recent developments in laser technology has hoped to change all of that: Breakthrough Starshot. By accelerating a “laser sail” to appreciable fractions of the speed of light, the project hopes to send an attached micro-spacecraft to interstellar destinations in decades, not millennia. But would those proposed spacecrafts survive the journey? That’s what Patreon supporter George Church wants to know, asking:
“If Breakthrough Starshot were to go at velocity=0.2c from Earth to [the] Alpha Centauri system, how many particles (protons, dust grains, etc.) and temperatures would be encountered, and what would be the consequences of each on a thin light sail?”
It’s a fascinating question, and we know enough about the Universe to calculate the answer. Let’s dive in and find out.
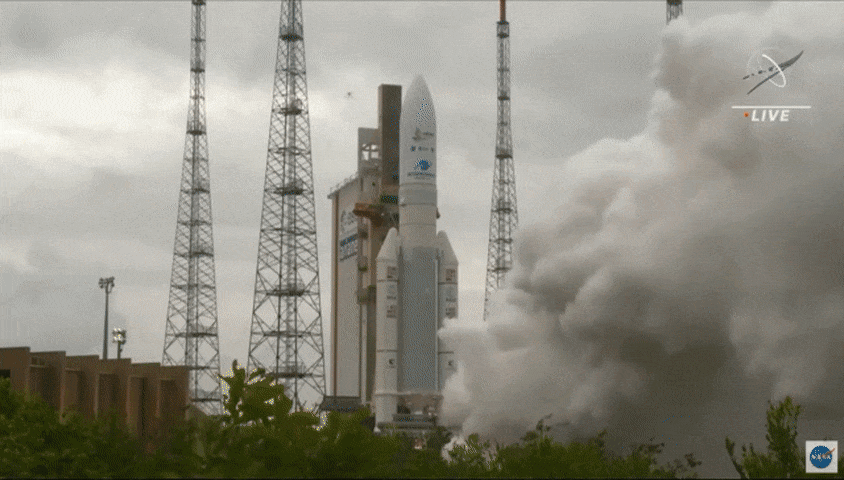
The only way we’ve ever ventured beyond planet Earth is through the science of rocketry: where fuel and energy are expended, creating thrust, and that thrust accelerates the spacecraft. Through gravitational encounters with other massive objects, such as the planets within our Solar System, we can give these spacecraft extra “kicks,” accelerating them to even greater speeds.
Fundamentally, it’s the thrust from the rockets themselves that are limited, as they run on chemical fuel. When you extract energy based on chemical reactions, it’s the transitions in how electrons and atoms are bound together that liberates energy, and that energy is only an extremely tiny fraction of the total mass involved: something like a millionth of a percent of the mass can get converted into energy.
If we could leverage a more efficient fuel — involving nuclear reactions or matter-antimatter annihilations, for example — it would be possible to convert more of the rocket’s on-board mass into energy, allowing us to reach greater velocities and shorten our journeys to faraway destinations. However, that technology does not yet exist, and so practical space travel is limited by these factors. At least, so far.
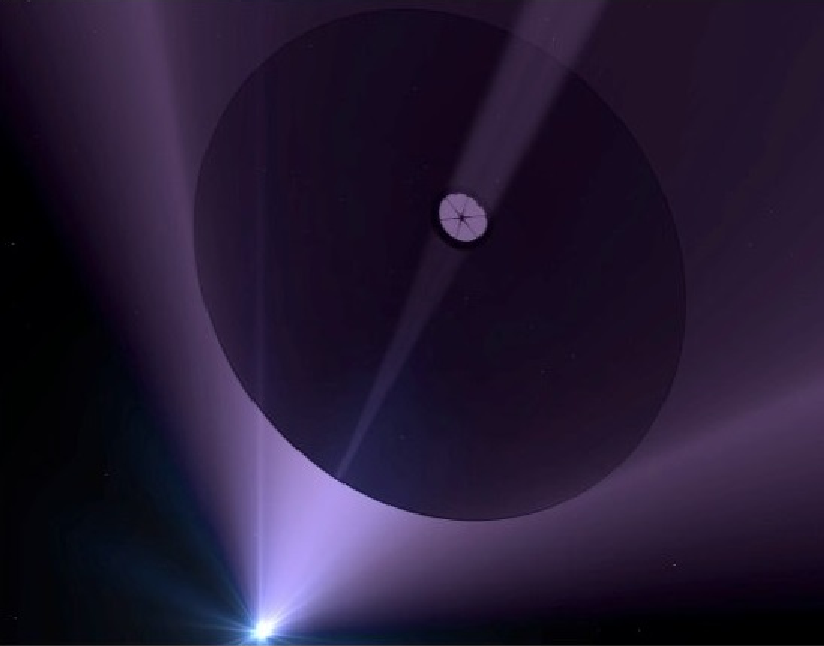
The revolutionary idea behind the Breakthrough Starshot project relies on recent advances in laser technology. The amount of power output that individual lasers are capable of, as well as the level of collimation that lasers can achieve have both increased substantially over the past two decades, while the cost of high-powered lasers have dropped alongside those developments. As a result, you can envision what I consider an ideal scenario, as follows:
- An array of high-powered lasers is constructed in space.
- A series of nanotechnology-based spacecraft are constructed, and attached to a thin, light, highly reflective but sturdy “sail.”
- The total mass of the spacecraft and the sail, combined, comes in only at about one gram.
- Then the laser array fires at one nanocraft at a time, accelerating it in one direction — towards its ultimate interstellar destination — to as great a speed as possible for as long as possible.
- After a journey across the interstellar medium, it arrives at its destination, where it gathers information, takes data, and transmits it back across the same interstellar distance, all the way back to Earth.
That’s the “dream scenario,” and even this scenario is too optimistic, in detail, to be considered by the Breakthrough Starshot team.
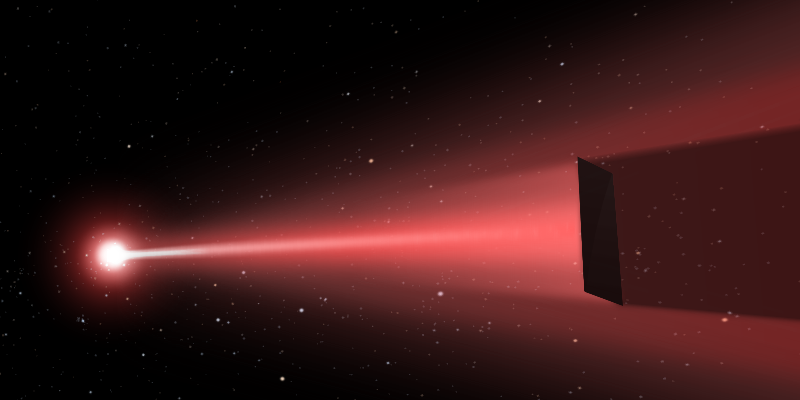
For one, they don’t envision a laser array in space, but rather on the ground, where the lasers themselves are dispersed by the atmosphere. This is a cost-saving measure that eliminates the need to launch and assemble the array in space, but it comes with its own obstacles, as Engineering Director of Breakthrough Initiatives Pete Klupar put it:
“The primary effort (and funding) is focused on the ability to coherently combine a near infinite number of lasers.”
Even with our current best adaptive optics and phased array technologies implemented, a terrestrial laser array, even at high altitudes, would need to see improvements of a factor between 10 and 100 to be viable. In addition, even the most reflective surfaces known to humanity — which reflect 99.999% of the energy incident upon them — would currently absorb about ~0.001% of the total energy impacting them. This is, at least at present, doubly catastrophic.
- It would incinerate the light sail in short order, rendering it useless and incapable of accelerating to anywhere near the design parameters.
- The light sail itself, while being accelerated by the incident lasers, would experience a differential force on it across its surface, creating a torque and causing the sail to rotate, making a continuous, directed acceleration an impossibility.
Additional obstacles pose difficulties that go well beyond the limits of current technology, and every one of them must be overcome to achieve Breakthrough Starshot’s goal.
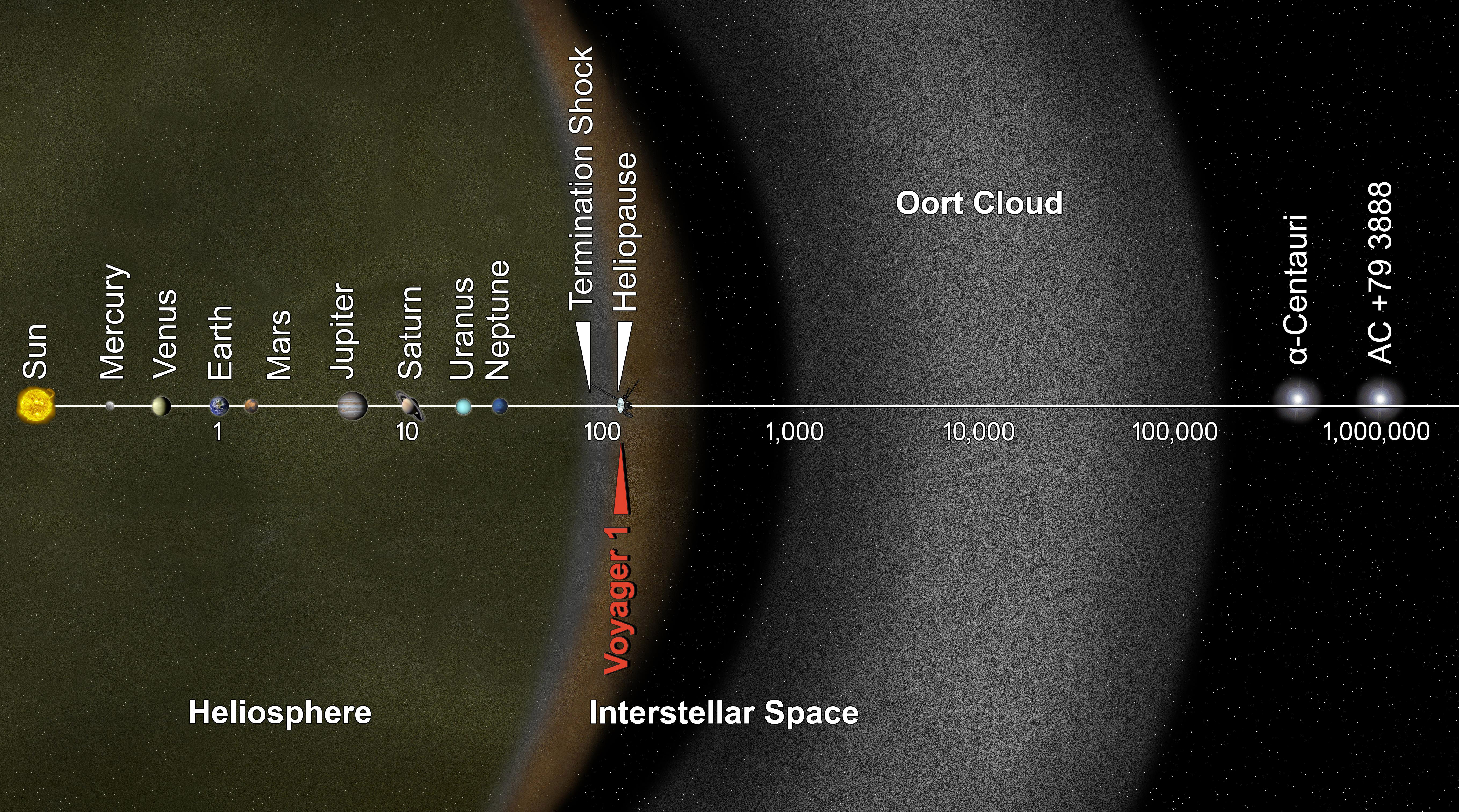
But let’s assume, for the sake of argument, that all of these obstacles not only can be, but actually will be, overcome. Assume we can:
- create an array of powerful enough, collimated enough lasers
- create a sub-gram nanocraft with all of the proper equipment on board its chip
- create a sufficiently reflective, light, and stable-against-rotations light sail
- accelerate and direct this spacecraft towards the nearest star system: Proxima/Alpha Centauri
Let’s even assume we can reach our desired speeds: 20% the speed of light, or ~60,000 km/s. That’s approximately 300 times the speed of a typical star through our galaxy, or a few thousand times the relative speed of the stars through the interstellar medium.
So long as we remain within the Solar System, the largest threat comes from dust particles, or the same types of micrometeoroids that typically punch holes through the spacecraft we launch in our own planet’s vicinity. The big enemy of keeping our spacecraft intact is simply kinetic energy, which — even at 20% the speed of light — is still well-approximated by our simple, non-relativistic formula: KE = ½mv2, where m is mass and v is the relative speed of the particles colliding with our object.
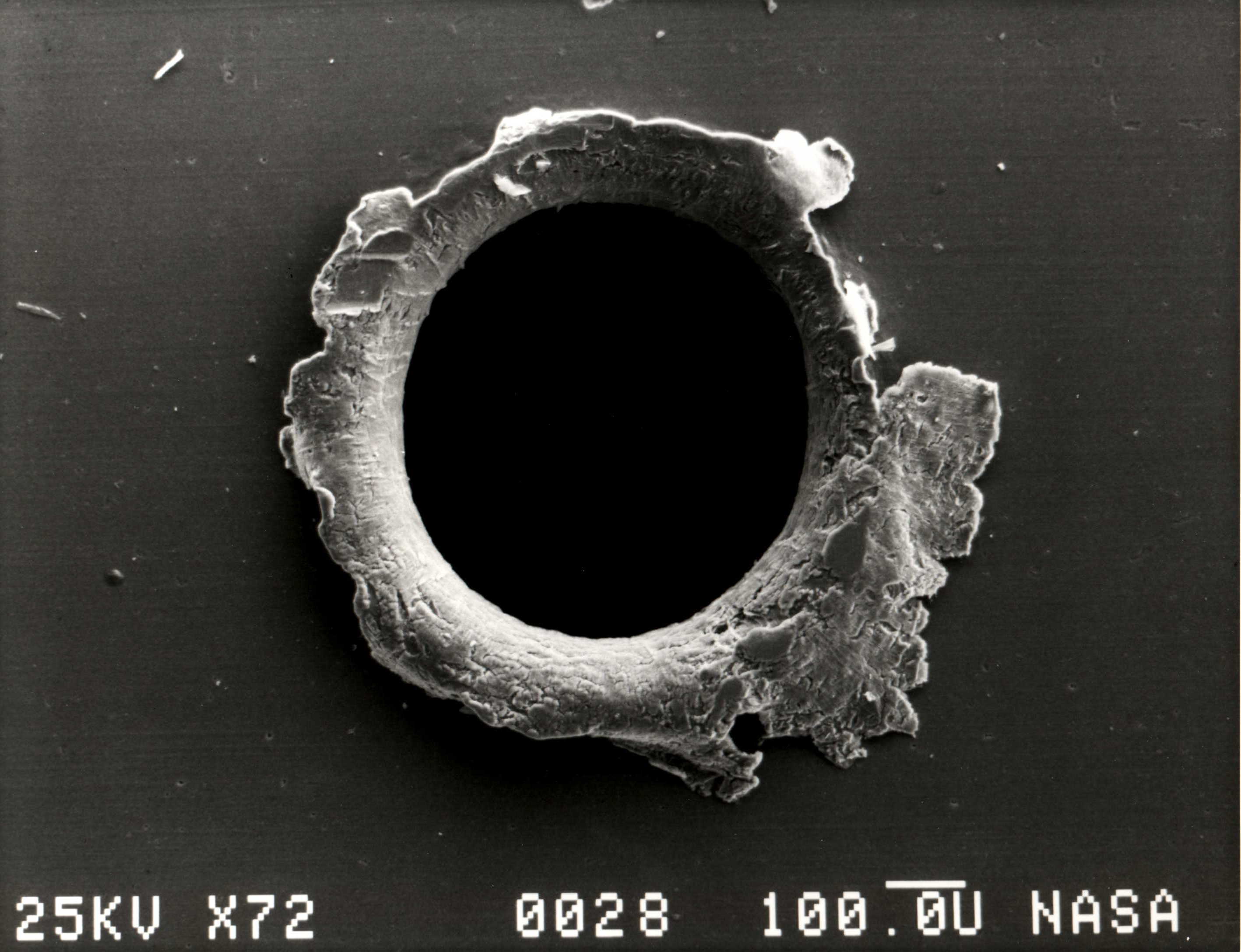
Once we leave the Solar System, however, the density and size distribution of particles that a traveling spacecraft will encounter changes. The best data we have for that comes from a combination of modeling, remote observations, and direct sampling courtesy of the Ulysses mission. The average density of a cosmic dust particle is about 2.0 grams per cubic centimeter, or about twice the density of water. Most of the cosmic dust particles are tiny and low in mass, but some are larger and more massive.
If you were able to reduce the cross-sectional size of your entire spacecraft to one square centimeter, you’d expect, on a ~4 light-year journey, to encounter no particles that are ~1 micron or larger in diameter; you’d only have about a 10% chance of doing so. However, as you look to smaller particles, you start to anticipate a much larger number of collisions:
- 1 collision with particles about ~0.5 microns in diameter
- 10 collisions with particles about ~0.3 microns in diameter
- 100 collisions with particles about ~0.18 microns in diameter
- 1000 collisions with particles about ~0.1 microns in diameter
- 10,000 collisions with particles about ~0.05 microns in diameter
- 100,000 collisions with particles about ~0.03 microns in diameter
- 1,000,000 collisions with particles about ~0.018 microns in diameter
- 10,000,000 collisions with particles about ~0.01 microns in diameter
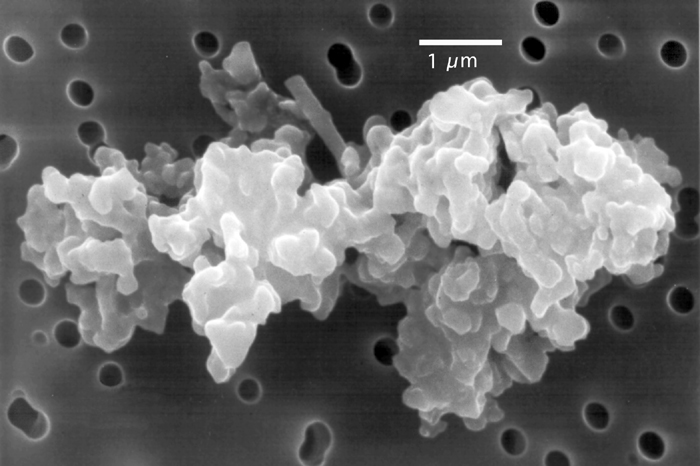
You might think this is not a big deal, to encounter such a large number of such tiny particles, especially when you consider how minuscule the mass of such particles would be. For example, the largest particle you’d hit, at 0.5 microns in diameter, would only have a mass of about 4 picograms (4 × 10-12 g). By the time you got down to a particle of ~0.1 microns in diameter, its mass would be a paltry 20 femtograms (2 × 10-14 g). And at a size of ~0.01 microns in diameter, a particle would only have a mass of 20 attograms (2 × 10-17 g).
But this, when you do the math, is disastrous. It isn’t the biggest particles that impart the most energy to a spacecraft traveling through the interstellar medium, but the smallest ones. At 20% the speed of light, a ~0.5 micron diameter particle will impart 7.2 Joules of energy to this tiny spacecraft, or about as much as energy as it takes to raise a 5 pound (~2.3 kg) weight from the ground to over your head.
Now, a ~0.01 micron diameter particle, also moving at ~20% the speed of light, will only impart 36 micro-Joules of energy to that same spacecraft: what seems like a negligible amount.
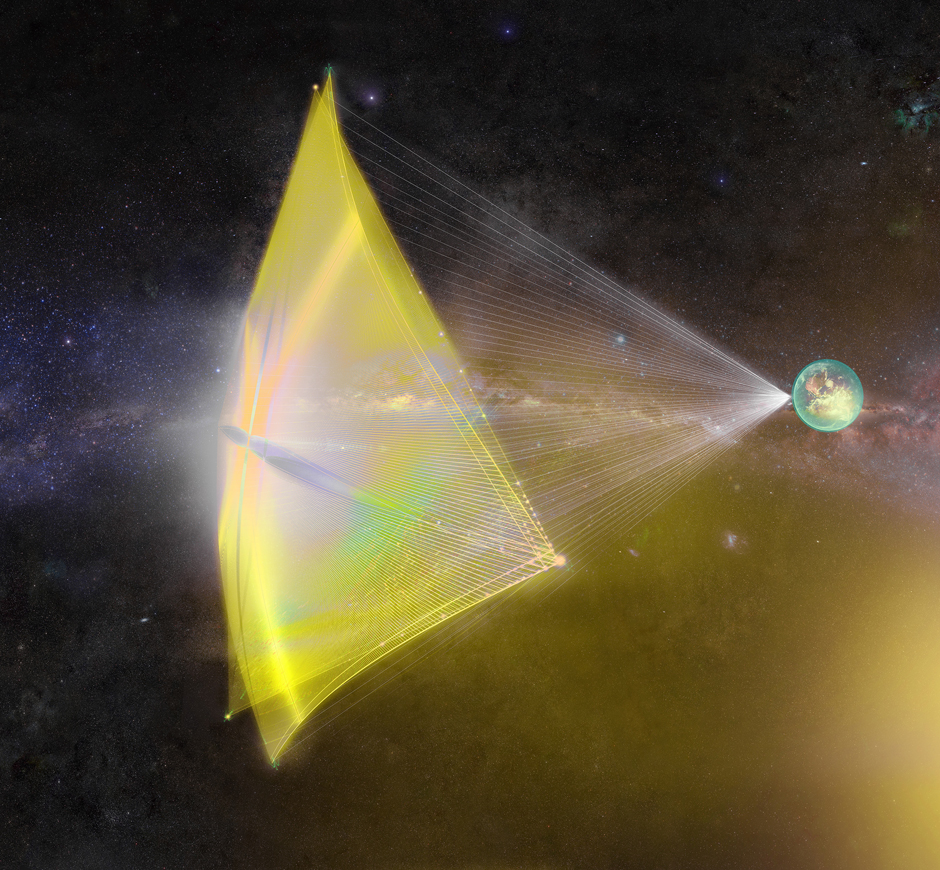
But these latter collisions are 10 million times more frequent than the largest collisions expected to occur. When we look at the total energy loss anticipated from dust grains that are ~0.01 microns or larger, it’s straightforward to calculate that there are a total of about ~800 Joules of energy that will be deposited into every square centimeters of this spacecraft from collisions with the various-sized dust particles in the interstellar medium.
Even though it will be spread out, in time and over the cross-sectional area of this tiny spacecraft, that’s a tremendous amount of energy for something that has a mass of only ~1 gram or so. It teaches us a few valuable lessons:
- The current Breakthrough Starshot idea, of applying a protective coating of a material like beryllium copper to the nanocraft, is wildly insufficient.
- The laser sail will be in danger of becoming absolutely shredded in short order and will also cause a substantial drag on the nanocraft if it isn’t jettisoned or (somehow) folded and stowed after the initial laser-driven acceleration takes place.
- Collisions from even smaller objects — things like the molecules, atoms, and ions that exist throughout the interstellar medium — will add up as well, and will potentially have even greater cumulative effects than dust particles will.
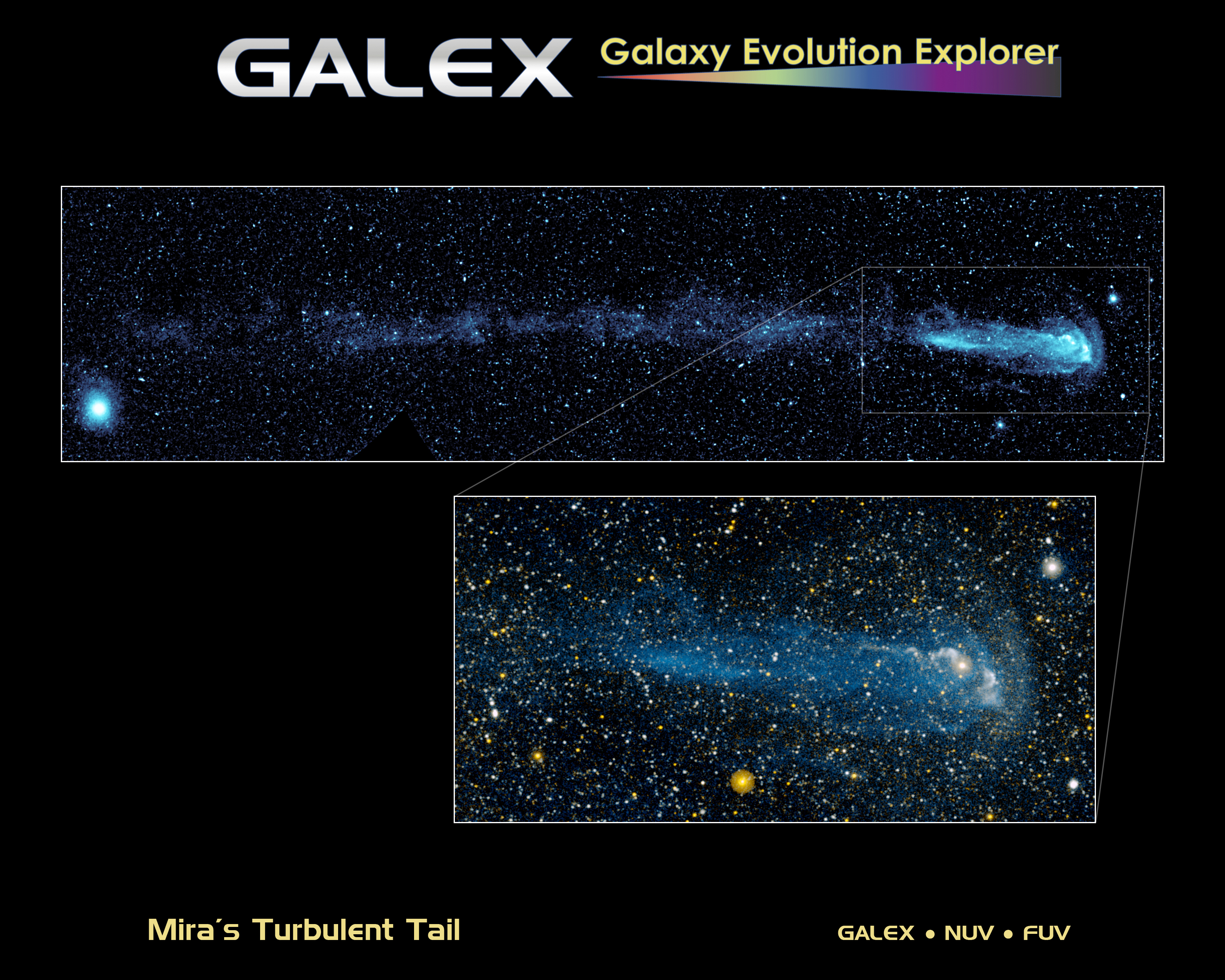
There are, of course, clever solutions to many of these problems that are available. For example, if you determined that the light sail itself would suffer too much damage or would slow down your journey by too great an amount, you could simply detach it once the laser acceleration stage was complete. If you designed your nanocraft — the “spacecraft” part of the apparatus — to be very thin, you could direct it to travel so that its cross-section was minimized. And if you determined that the damage from ions would be substantial, you could potentially set up a continuous electric current through the spacecraft, generating its own magnetic field to deflect charged cosmic particles.
However, each of these interventions comes along with its own drawbacks. The goal of the mission, remember, is to not only reach a distant star system, but to record data and transmit it back to Earth. If you jettison the laser sail, you lose your ability to transmit that data back, as the sail itself was designed to also participate in data transmission. If you make your spacecraft very thin, then you have to worry about collisions imparting angular momentum to it, where the craft may wind up spinning out of control. And any magnetic field the spacecraft generates runs the risk of changing its trajectory dramatically, as the interstellar medium has non-negligible electric and magnetic fields in it as well, which interact.
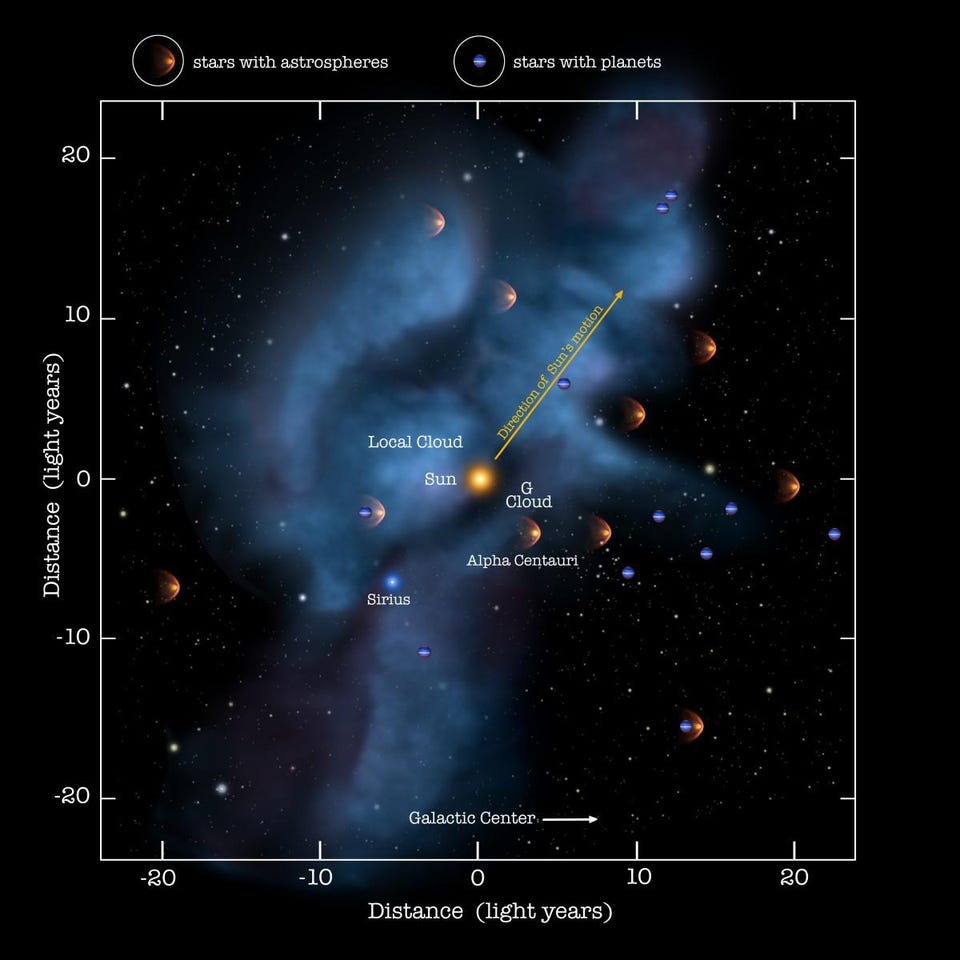
The best thing, at present, that one can state about the Breakthrough Starshot initiative is that there are no violations of the known laws of physics that need to occur in order for the mission to succeed. We “only” need, and this is a very loose definition of “only,” to overcome a tremendous series of engineering problems that have never been addressed on a scale like this before. To keep this spacecraft operational during a multi-decade, ultra-high-speed journey through multiple light-years of interstellar space will require advances that are far in excess of what is even being actively researched today.
Nevertheless, taking on the most challenging, ambitious problems is often how we motivate the largest leaps and breakthroughs in science and technology. Although we probably won’t, as the scientists behind the initiative are often fond of claiming, be able to reach and communicate from another star system within our present lifetimes, there’s every reason to make the most earnest attempt we can toward that goal. While we should fully expect to fail in dozens of novel, spectacular ways along the journey, those failed attempts are precisely what’s needed to pave the eventual road to success. After all, the greatest folly, when reaching for the stars, is to fail to even make the attempt.
Send in your Ask Ethan questions to startswithabang at gmail dot com!