No, Roger Penrose, We See No Evidence Of A ‘Universe Before The Big Bang’

Despite the claims of one of Earth’s newest Nobel Laureates, the data doesn’t lie.
One of the greatest scientific successes of the past century was the theory of the hot Big Bang: the idea that the Universe, as we observe it and exist within it today, emerged from a hotter, denser, more uniform past. Originally proposed as a serious alternative to some of the more mainstream explanations for the expanding Universe, it was shockingly confirmed in the mid-1960s with the discovery of the “primeval fireball” that remained from that early, hot-and-dense state: today known as the Cosmic Microwave Background.
For more than 50 years, the Big Bang has reigned supreme as the theory describing our cosmic origins, with an early, inflationary period preceding it and setting it up. Both cosmic inflation and the Big Bang have been continually challenged by astronomers and astrophysicists, but the alternatives have fallen away each time that new, critical observations have come in. Even 2020 Nobel Laureate Roger Penrose’s attempted alternative, Conformal Cyclic Cosmology, cannot match the inflationary Big Bang’s successes. Contrary to recent headlines and Penrose’s assertions, there is no evidence of “a Universe before the Big Bang.”
The Big Bang is commonly presented as though it were the beginning of everything: space, time, and the origin of matter and energy. From a certain archaic point of view, this makes sense. If the Universe we see is expanding and getting less dense today, then that means it was smaller and denser in the past. If radiation — things like photons — is present in that Universe, then the wavelength of that radiation will stretch as the Universe expands, meaning it cools as time goes on and was hotter in the past.
At some point, if you extrapolate back far enough, you’ll achieve densities, temperatures, and energies that are so great that you’ll create the conditions for a singularity. If your distance scales are too small, your timescales are too short, or your energy scales are too high, the laws of physics cease to make sense. If we run the clock backwards some 13.8 billion years towards the mythical “0” mark, those laws of physics break down at a time of ~10^-43 seconds: the Planck time.
If this were an accurate depiction of the Universe — that it began hot and dense and then expanded and cooled — we’d expect a large number of transitions to occur in our past history.
- All the possible particles and antiparticles would get created in great numbers, with the excess annihilating away to radiation when it gets too cool to continually create them.
- The electroweak and Higgs symmetries break when the Universe cools below the energy at which those symmetries are restored, creating four fundamental forces and particles with non-zero rest masses.
- Quarks and gluons condense to form composite particles like protons and neutrons.
- Neutrinos stop interacting efficiently with the surviving particles.
- Protons and neutrons fuse to form the light nuclei: deuterium, helium-3, helium-4, and lithium-7.
- Gravitation works to grow the overdense regions, while radiation pressure works to expand them when they get too dense, creating a set of oscillatory, scale-dependent imprints.
- And approximately 380,000 years after the Big Bang, it becomes cool enough to form neutral, stable atoms without them being instantly blasted apart.
When this last stage occurs, the photons permeating the Universe, which had previously scattered off of the free electrons, simply travel in a straight line, lengthening in wavelength and diluting in number as the Universe expands.
Some ~55 years ago, this background of cosmic radiation was first detected, catapulting the Big Bang from one of a few viable options for our Universe’s origin to the only one consistent with the data. While most astronomers and astrophysicists immediately accepted the Big Bang, the strongest proponents of the leading alternative Steady-State theory — people like Fred Hoyle — came up with progressively more and more absurd contentions to defend their discredited idea in the face of overwhelming data.
But each idea failed spectacularly. It couldn’t have been tired starlight. Nor reflected light, nor dust that was heated up and radiating. Each and every explanation that was tried was refuted by the data: the spectrum of this cosmic afterglow was too perfect a blackbody, too equal in all directions, and too uncorrelated with the matter in the Universe to line up with these alternative explanations. While science moved on to the Big Bang becoming part of the consensus, i.e., a sensible starting point for future science, Hoyle and his ideological allies worked to hold back the progress of science by advocating for scientifically untenable alternatives.
Ultimately, science moved on while the contrarians became more and more irrelevant, with their trivially incorrect work fading into obscurity and their research programme eventually ceasing upon their deaths.
In the meantime, from the 1960s up through the 2000s, the sciences of astronomy and astrophysics — and particularly the sub-field of cosmology, which focuses on the history, growth, evolution, and fate of the Universe — grew spectacularly.
- We mapped out the large-scale structure of the Universe, discovering a great cosmic web.
- We discovered how galaxies grew and evolved, and how their stellar populations inside changed with time.
- We learned that all the known forms of matter and energy in the Universe were insufficient to explain everything we observe: some form of dark matter and some form of dark energy are required.
And we were able to further verify additional predictions of the Big Bang, such as the predicted abundances of the light elements, the presence of a population of primordial neutrinos, and the discovery of density imperfections of exactly the necessary type to grow into the large-scale structure of the Universe we observe today.
At the same time, there were observations that were no doubt true, but that the Big Bang had no predictive power to explain. The Universe allegedly reached these arbitrarily high temperatures and high energies at the earliest times, and yet there are no exotic leftover relics that we can see today: no magnetic monopoles, no particles from grand unification, no topological defects, etc. Theoretically, something else beyond what is known must be out there to explain the Universe we see, but if they ever existed, they’ve been hidden from us.
The Universe, in order to exist with the properties we see, must have been born with a very specific expansion rate: one that balanced the total energy density exactly, to more than 50 significant digits. The Big Bang has no explanation for why this should be the case.
And the only way different regions of space would have the same exact temperature is if they’re in thermal equilibrium: if they have time to interact and exchange energy. Yet the Universe is too big and has expanded in such a way that we have many causally disconnected regions. Even at the speed of light, those interactions couldn’t have taken place.
This presents a tremendous challenge for cosmology, and for science in general. In science, when we see some phenomena that our theories cannot explain, we have two options.
- We can attempt to devise a theoretical mechanism to explain those phenomena, while simultaneously maintaining all the successes of the prior theory and making novel predictions that are distinct from the prior theory’s predictions.
- Or we can simply assume that there is no explanation, and the Universe was simply born with the properties necessary to give us the Universe we observe.
Only the first approach has scientific value, and therefore that’s the one that must be tried, even if it fails to yield fruit. The most successful theoretical mechanism for extending the Big Bang has been cosmic inflation, which sets up a phase before the Big Bang where the Universe expanded in an exponential fashion: stretching it flat, giving it the same properties everywhere, matching the expansion rate with the energy density, eliminating any prior high-energy relics, and making the new prediction of quantum fluctuations — leading to a specific type of density and temperature fluctuations — superimposed atop an otherwise uniform Universe.
Although inflation, like the Big Bang before it, had a large number of detractors, it succeeds where all the alternatives fail. It solves the “graceful exit” problem, where an exponentially expanding Universe can transition into a matter-and-radiation-filled Universe that expands in a way that matches our observations, meaning it can reproduce all the successes of the hot Big Bang. It imposes an energy cutoff, eliminating any ultra-high-energy relics. It creates a uniform Universe to an enormously high degree, where the expansion rate and the total energy density match perfectly.
And it makes novel predictions about the types of structure and the initial temperature and density fluctuations that should appear, predictions that have subsequently been borne out to be correct by observations. Inflation’s predictions were largely teased out in the 1980s, while the observational evidence that validated it has come in a trickling stream over the past ~30 years. Although alternatives abound, none are as successful as inflation.
Unfortunately, Nobel Laureate Roger Penrose, although his work on General Relativity, black holes, and singularities in the 1960s and 1970s was absolutely Nobel-worthy, has spent a large amount of his efforts in recent years on a crusade to overthrow inflation: by promoting a vastly scientifically inferior alternative, his pet idea of a Conformal Cyclic Cosmology, or CCC.
The biggest predictive difference is that the CCC pretty much requires that an imprint of “the Universe before the Big Bang” show itself in both the Universe’s large-scale structure and in the cosmic microwave background: the Big Bang’s leftover glow. Contrariwise, inflation demands that anywhere where inflation ends and a hot Big Bang arises must be causally disconnected from, and cannot interact with, any prior, current, or future such region. Our Universe exists with properties that are independent of any other.
The observations — first from COBE and WMAP, and more recently, from Planck — definitively place enormously tight constraints (to the limits of the data that exists) on any such structures. There are no bruises on our Universe; no repeating patterns; no concentric circles of irregular fluctuations; no Hawking points. When one analyzes the data properly, it is overwhelmingly clear that inflation is consistent with the data, and the CCC is quite clearly not.
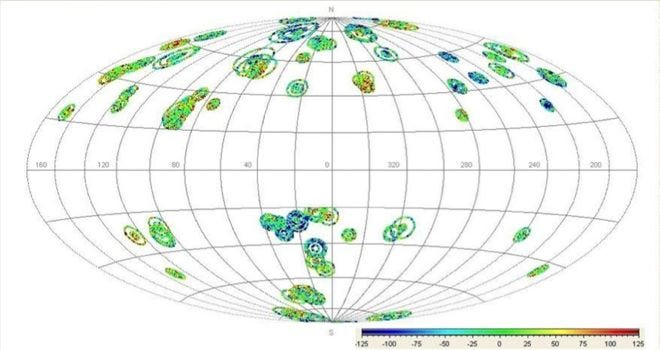
Although, much like Hoyle, Penrose isn’t alone in his assertions, the data is overwhelmingly opposed to what he contends. The predictions that he’s made are refuted by the data, and his claims to see these effects are only reproducible if one analyzes the data in a scientifically unsound and illegitimate fashion. Hundreds of scientists have pointed this out to Penrose — repeatedly and consistently over a period of more than 10 years — who continues to ignore the field and plow ahead with his contentions.
Like many before him, he appears to have fallen so in love with his own ideas that he no longer looks to reality to responsibly test them. Yet these tests exist, the critical data is publicly available, and Penrose is not just wrong, it’s trivially easy to demonstrate that the features he claims should be present in the Universe do not exist. Hoyle may have been denied a Nobel Prize despite his worthy contributions to stellar nucleosynthesis because of his unscientific stances later in life; although Penrose now has a Nobel, he has succumbed to the same regrettable pitfall.
While we should laud the creativity of Penrose and celebrate his groundbreaking, Nobel-worthy work, we must guard ourselves against the urge to deify any great scientist, or the work they engage in that isn’t supported by the data. In the end, regardless of celebrity or fame, it’s up to the Universe itself to discern for us what’s real and what’s merely an unsubstantiated hypothesis, and for us to follow the Universe’s lead, regardless of where it takes us.
Starts With A Bang is written by Ethan Siegel, Ph.D., author of Beyond The Galaxy, and Treknology: The Science of Star Trek from Tricorders to Warp Drive.