Ask Ethan: Why Doesn’t The Afterglow Of The Big Bang Eventually Fade Away?

It happened 13.8 billion years ago, so why hasn’t the radiation all passed over us by now?
For the past 13.8 billion years, our Universe has been expanding, cooling, and gravitating. The hot Big Bang itself was, at least for our observable Universe, a one-time event that was the proverbial starting gun for everything that’s happened since. As we expanded and cooled, we formed atomic nuclei, neutral atoms, stars, galaxies, and eventually, rocky planets like Earth. Yet somehow, as we look out into the Universe, we can still see the leftover glow originating from the Big Bang — the Cosmic Microwave Background (CMB) — even today. How is this possible? That’s what Lothar Voigt wants to know, asking:
Why is the CMB washing over us continuously and not just as a one-time event at some point in our own past or future? If the Sun suddenly turned transparent, all the light would rush out and that’s then the end of it. Sunspots and all. What am I missing?
It’s a deep question, but it represents a great opportunity to learn how our Universe truly works. Let’s dive in.

When we look out in our Universe at any object that emits light, we’re not seeing that object as it exists today, right at this very moment, where the exact number of seconds have elapsed since the Big Bang as they have for us. Instead, we’re seeing that object as it was in the past: back when that light was emitted. That light is then required to journey through the Universe until it arrives at our eyes.
When we see our Sun, we’re not observing the light it’s emitting right now, but rather the light it emitted 8 minutes and 20 seconds ago: the amount of time it takes light to traverse the Earth-Sun distance.
When we look at a star that’s hundreds or thousands of light-years away, we’re seeing it as it was hundreds or thousands of years ago; perhaps Betelgeuse, at 640 light-years away, has gone supernova at some point in the past 640 years. But if it has, that light hasn’t arrived.
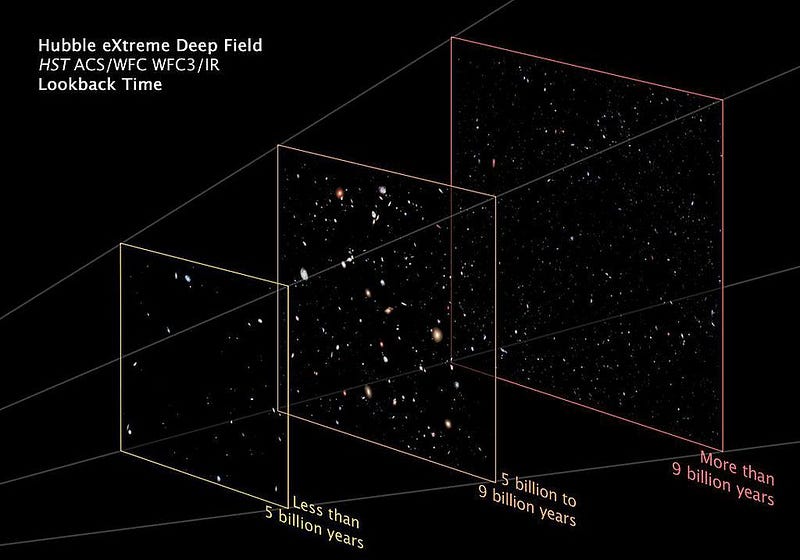
And when we look at a distant galaxy, we’re seeing light that’s millions or even billions of years old. That light was:
- generated millions or billions of years ago,
- travels millions or billions of years through the expanding Universe,
- and arrives at our eyes.
If a star in that galaxy goes supernova, we observe the supernova when the light arrives: not before and not after. If new stars form, we observe the light from the formation only when it arrives, not before or after, and the light from the stars only after they form and it has time to arrive. When those stars die, their light ceases to be emitted, and hence, once it passes by us, we’ll never see them again.
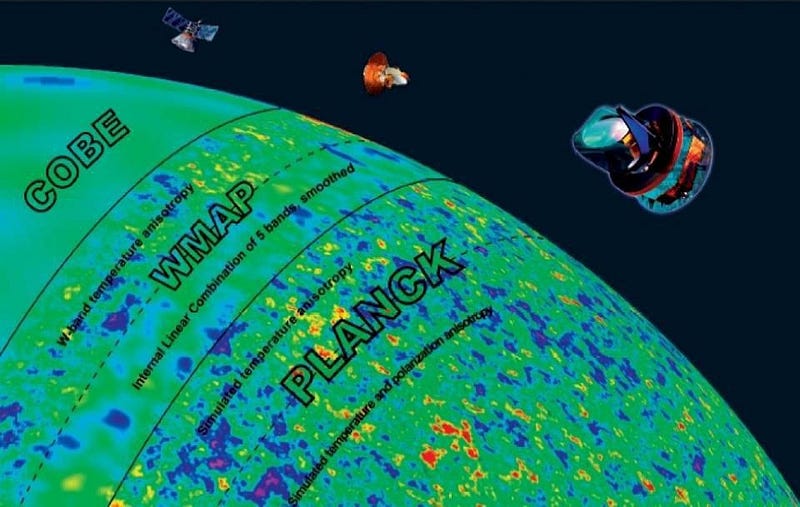
On the other hand, light from the Big Bang is still visible today, even though the Big Bang itself occurred 13.8 billion years ago. If we had been around just 1 million years after the Big Bang, we would have been able to see that light as well, although it would be at higher energies, since the Universe would have expanded by a smaller amount and the light would have shorter wavelengths and hence higher temperatures.
The more time that goes by, the more we see that leftover light:
- decrease in temperature,
- decrease in the number density of photons,
- and decrease in importance relative to matter and dark energy.
Despite all of these changes, and despite the fact that the Big Bang only occurred at one instant in time (a very long time ago), that leftover glow — once known as the primeval fireball and now known as the Cosmic Microwave Background (CMB) — continues to persist.
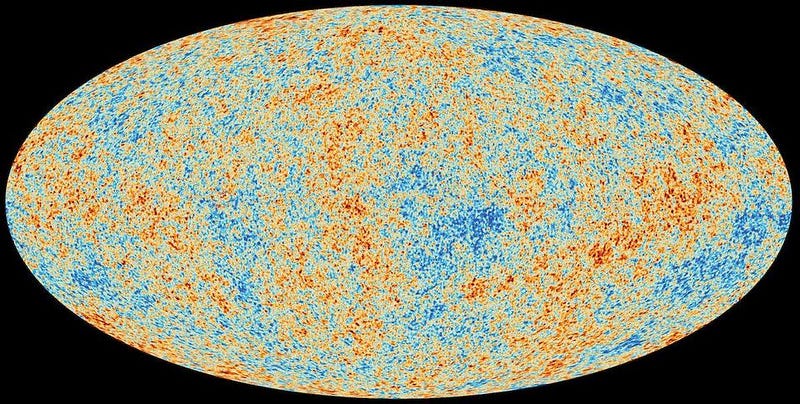
Rather than view this as a puzzle, we should treat this as an opportunity to understand how the light from the CMB is different from the light arriving from stars, galaxies, and individual astrophysical sources of light. For everything else in the Universe — everything that creates light — that light is:
- created at a particular location in space,
- created at a particular moment in time,
- travels away from the source, through the (expanding) Universe, at the speed of light,
- and arrives at our eyes, the observer, only for that one instant.
For stars, galaxies, supernovae, cataclysmic events, gas clouds, flares, and any other source of radiation, these things are all true. But for the Big Bang’s leftover glow, one very, very important thing is different. All of that radiation does come from a particular instant in time; it does travel through the Universe at the speed of light; it does arrive at our eyes at one particular instant. But it wasn’t created at just one location in space.
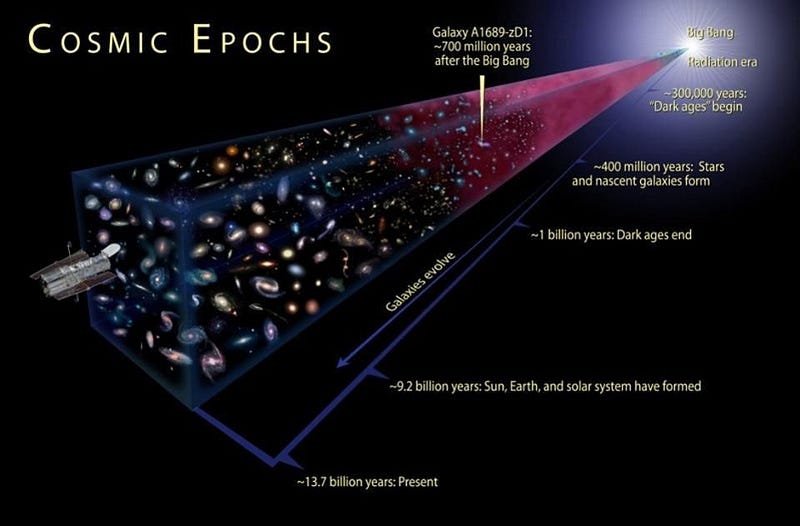
The biggest, hardest-to-understand difference about the Big Bang from everything else is that the Big Bang doesn’t have a point-of-origin. It’s not like a stellar event or explosion; there’s no location you can point to and say, “this is where the Big Bang happened: here, and nowhere else.” What makes the Big Bang so special is that it occurred everywhere at once.
The Big Bang represents a moment in time, 13.8 billion years ago, when the Universe was in an ultra-hot, ultra-dense state, filled with matter, antimatter, and radiation. Everything that’s occurred since that time has occurred in the aftermath of the Big Bang. The annihilation of antimatter (leaving just a tiny bit of normal matter behind), the formation of protons and neutrons, the fusion of light elements, the formation of neutral atoms, the first stars and galaxies, etc. All of that occurred everywhere throughout the Universe, but only as we move forward in time.
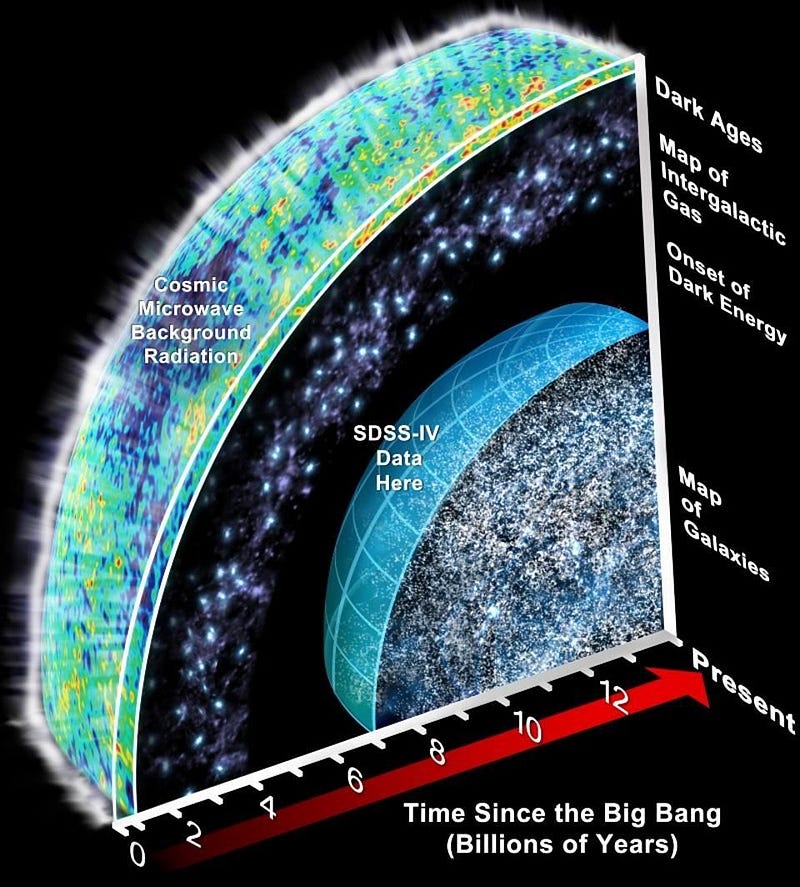
This is the key idea to understanding where this radiation comes from. When we see the Big Bang’s leftover glow, we are seeing the light that is only — right now — arriving at our eyes after a 13.8 billion year journey. The radiation we observe was emitted not at the instant of the Big Bang itself, but from a point in time that occurred 380,000 years later: when electrons finally were able to stably bind themselves to protons (and other atomic nuclei) without immediately being blasted apart again.
Prior to that time, radiation bounces back-and-forth off of all the free electrons populating the Universe. Put simply, photons (particles of light) and electrons interact frequently and easily; put technically, their cross-section is large. But once you form neutral atoms, and your light is low enough in energy, those neutral atoms then become transparent to that light.
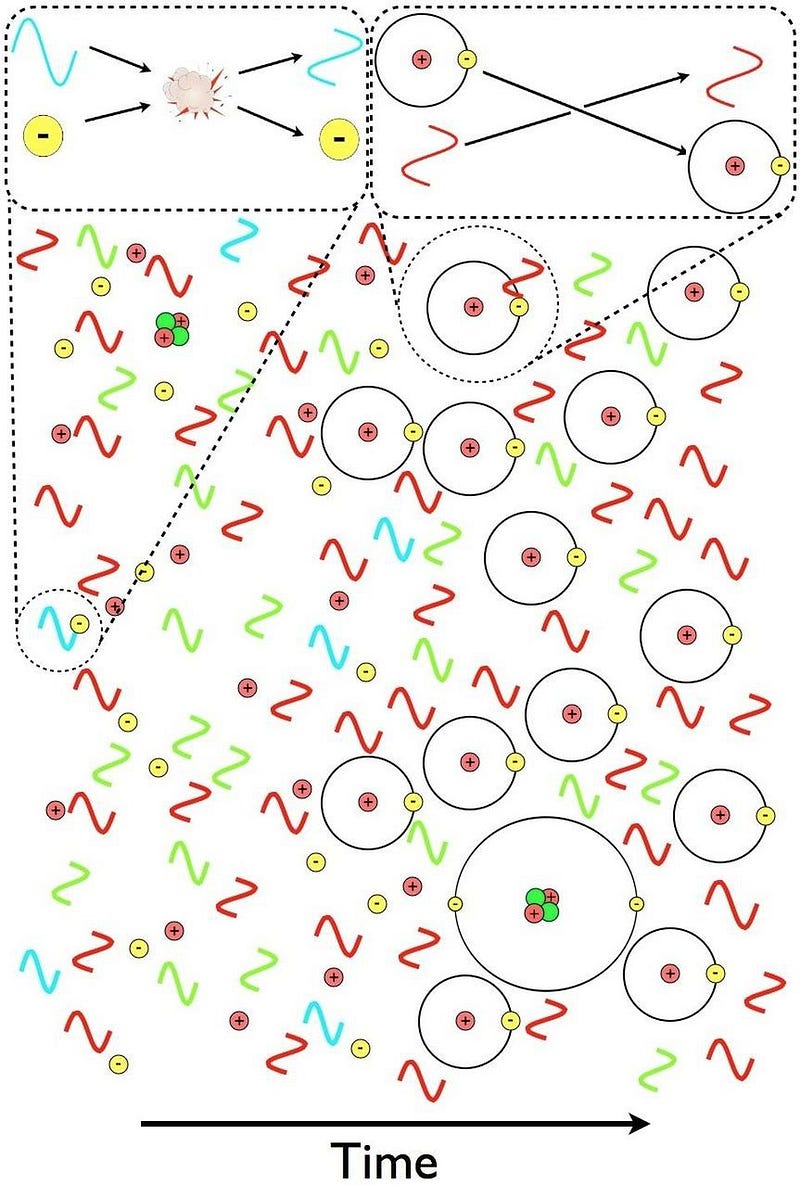
So what does that light do? The same thing all light does: it travels through the Universe, at the speed of light, until it reaches something for it to interact with.
But here’s the thing: that light is everywhere. That light — the light that we observe as making up the CMB — was emitted from all points in the Universe, everywhere, all at once, some 13.8 billion years ago. The light that was emitted from our location has been travelling away from us at the speed of light for the past 13.8 billion years, and owing to the expansion of the Universe, is now some 46 billion light-years away from us.
Similarly, the light that’s arriving at our eyes today was emitted 13.8 billion years ago, and the “surface” we see where the CMB originates from (from our perspective) is now 46 billion light-years away.
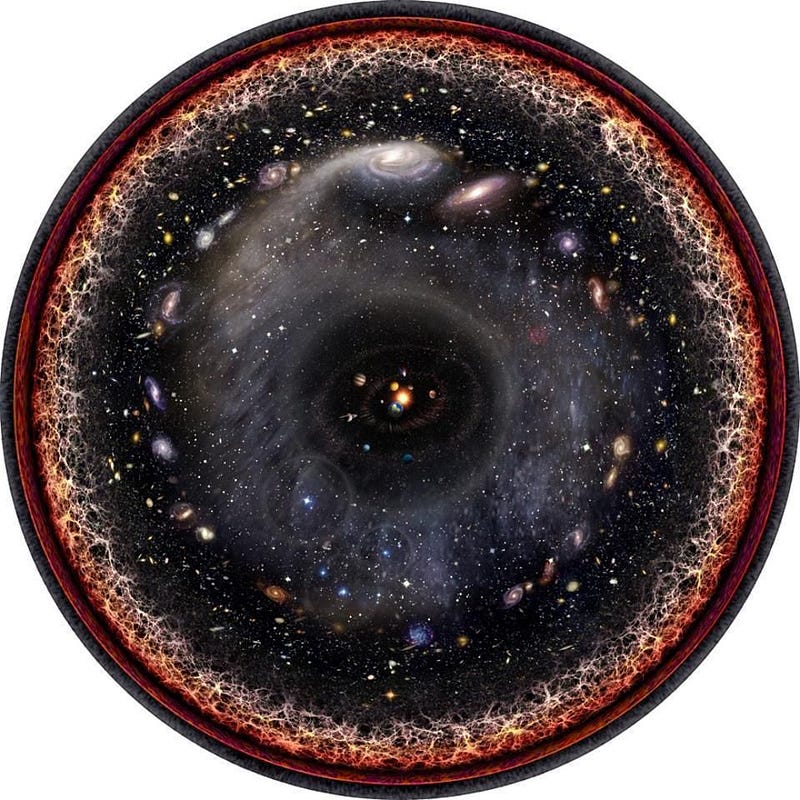
So what’s happening? The CMB light that arrived a second ago was emitted from a spherical surface that was slightly closer to us than the CMB light that’s arriving right now. The light that we observed the first time we detected the CMB more than half a century ago was even closer, while the light that we’ll observe in the far future is still on its way, coming to us from a point we can not yet see, since that light hasn’t arrived yet.
What this means is that the Universe, everywhere, right now, is filled with about 411 CMB photons for every cubic centimeter of space that we have. It also means, when we look at galaxies and other astronomical objects that are very far away, those objects were interacting with CMB photons that were:
- more numerous (because the Universe had expanded less),
- more energetic (because those photon wavelengths had been stretched less),
- and were at a higher temperature.
That last part is interesting, because radiation interacts with matter, and we can observe — and actually have observed — how the CMB was hotter in the past.

So what’s actually occurring? The CMB actually is washing over us right now, and this very moment is the only opportunity we’ll ever have to see those specific CMB photons that are arriving at Earth today. It took a 13.8 billion year journey across the expanding Universe to bring them to our eyes, but they’ve arrived after the most cosmic voyage of all: from the Big Bang to us.
But before those photons arrived, there were photons arriving from slightly closer locations. And after those photons are done arriving, they’ll be replaced by photons that are arriving from locations that are slightly further away. This will continue for all eternity, as while both the number density and energy of these photons will continue to drop, they’ll never go away completely. The Big Bang filled the entire Universe with this omnidirectional bath of radiation. As long as we exist in this Universe, the Big Bang’s leftover glow will always be with us.
Send in your Ask Ethan questions to startswithabang at gmail dot com!
Ethan Siegel is the author of Beyond the Galaxy and Treknology. You can pre-order his third book, currently in development: the Encyclopaedia Cosmologica.