NASA Mission To Set A ‘Depth Perception’ Record, And You Can Help

NASA’s New Horizons is the most distant technologically advanced observatory ever. And that makes all the difference.
When you look at an object that’s very distant from you, how well can you tell how far away it truly is? Our ability to do this is known as depth perception. Although some of our depth perception arises due to things like relative motions, apparent sizes, texture gradients, and other things you can observe with a single eye, the most universal visual cue comes from our binocular vision: two eyes that are located in different places from one another.
The separation between our eyes is key to three-dimensional imaging, or our sense of depth perception. In astronomy, this gets taken to the extreme, as two telescopes can be extremely well-separated in distance: the diameter of the Earth simultaneously, or more if they’re in space. The most distant operational telescope in communication with Earth is aboard NASA’s New Horizons, out past Pluto. On April 22 and 23, New Horizons will team up with Earth to produce the longest-baseline parallax measurement ever, and you can help. Here’s how, and the science behind it.
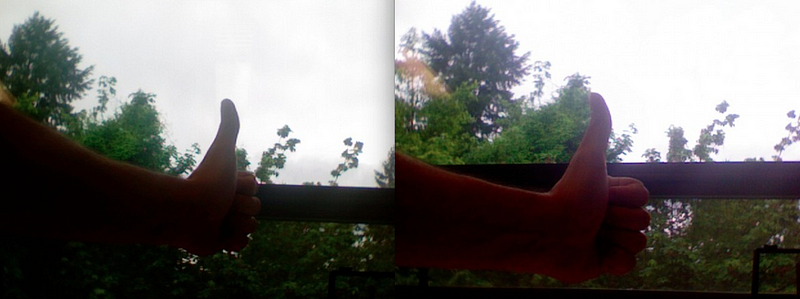
When you only have one eye open, you see the outside world similarly to a photograph: the three-dimensional world compressed down into a two-dimensional snapshot. Different objects truly are different distances away, but you cannot tell, on the basis of a single snapshot, whether objects are larger/brighter and far away, or whether they’re smaller/fainter and close by.
But if you have a second eye in a different location, it’s easy to visualize that you’re getting two sets of information for your brain to put together. The best way to “see” this for yourself is to hold up your thumb with your arm extended all the way in front of you, in front of a relatively distant background. As you switch between your left eye and your right eye, leaving only one open at a time, you’ll see the apparent position of your thumb move relative to the background.
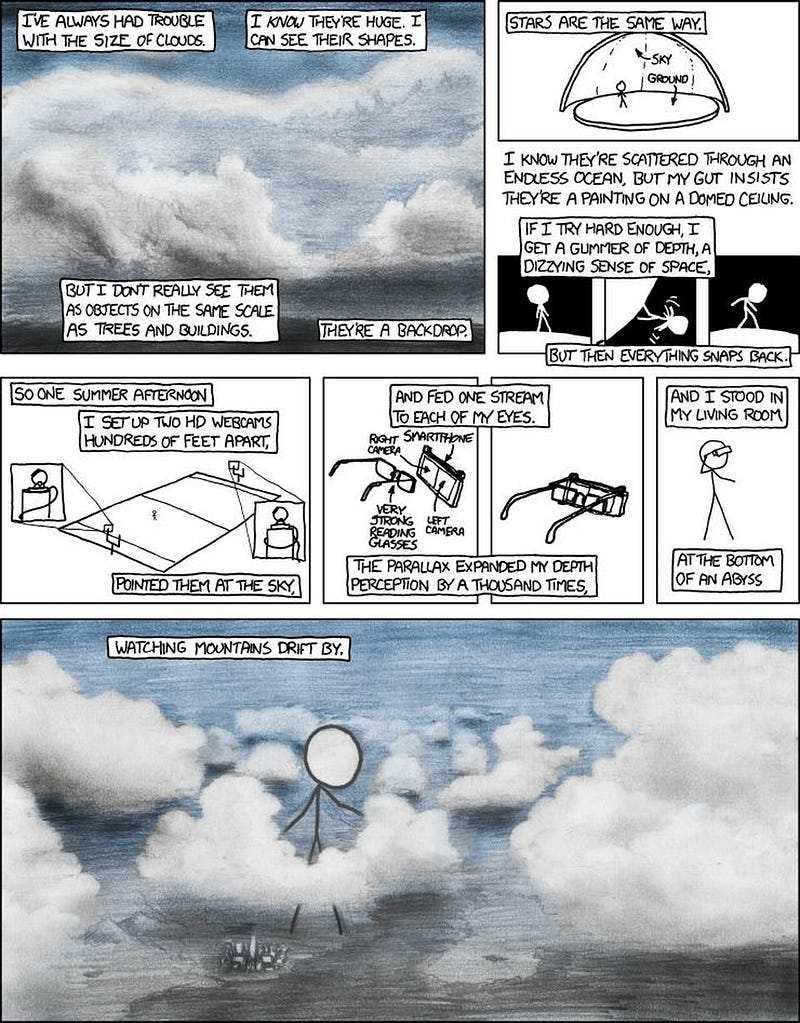
The reason your thumb appears to move is simple: the line-of-sight that you see with your left eye places your thumb in a different relative position to the line-of-sight of your right eye. Mathematically, your eyes make a narrow triangle with whatever object you’re looking at, and the closer that object is, the larger the narrow angle at the object gets. The farther away the object is, the angle gets so low that you cannot observe it.
If the object is infinitely far away, the angle drops to zero, which is why you cannot tell, with a snapshot from your eyes alone, whether the Moon or the planets or the stars are more distant than one another. But if the object is close enough that you can tell there’s an angular difference between your left eye’s view and your right’s, you’ll see what’s known in astronomy as a parallax.
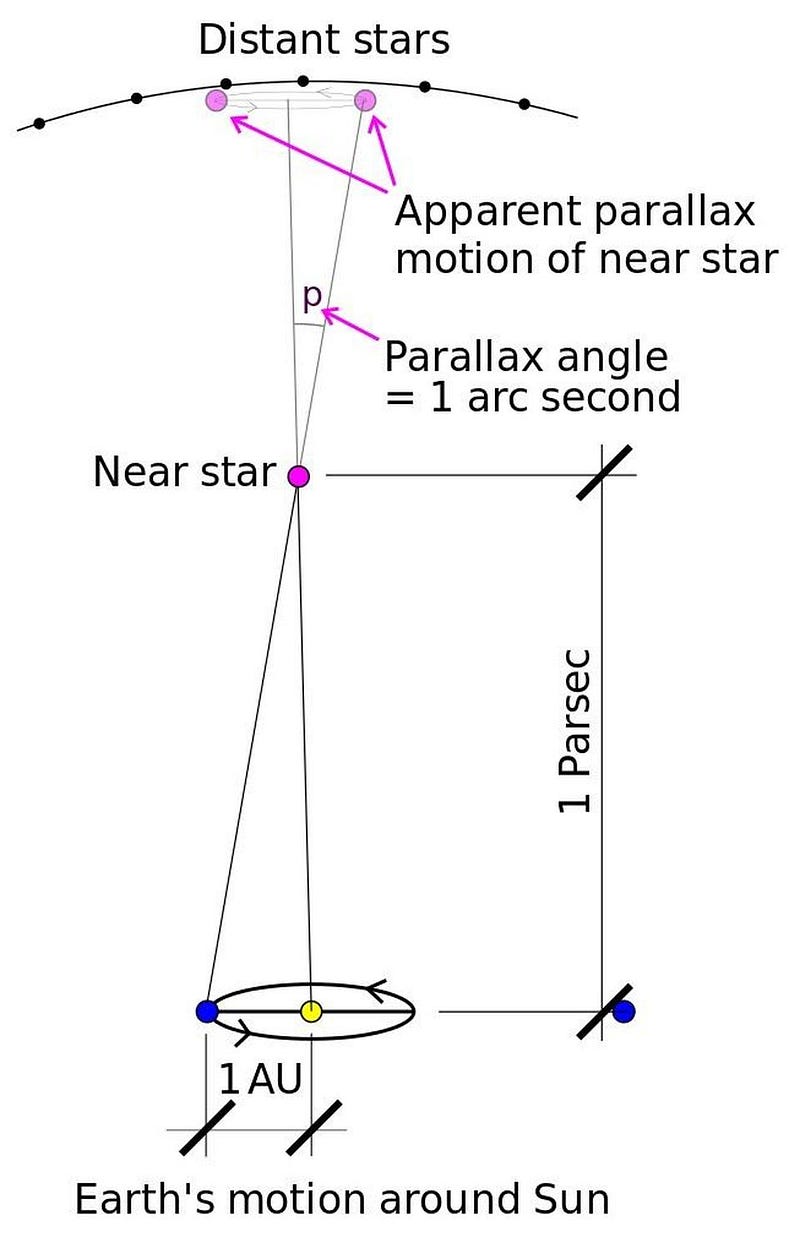
The parallax angle that a distant object appears to make, geometrically, is entirely dependent on just two distances:
- the separation distance between your two “eyes,”
- and the distance to that object.
While for most of us, the distance between our eyes might be only a few inches (around 6 or 7 cm), we’re not restricted to using our eyes alone for astronomy. We can set up telescopes all over the world, with a maximum baseline distance of Earth’s diameter: around 12,700 km. Although this might seem like an enormous distance, you have to compare it with the distances to the stars, which are measured in light years, or tens of trillions of kilometers.
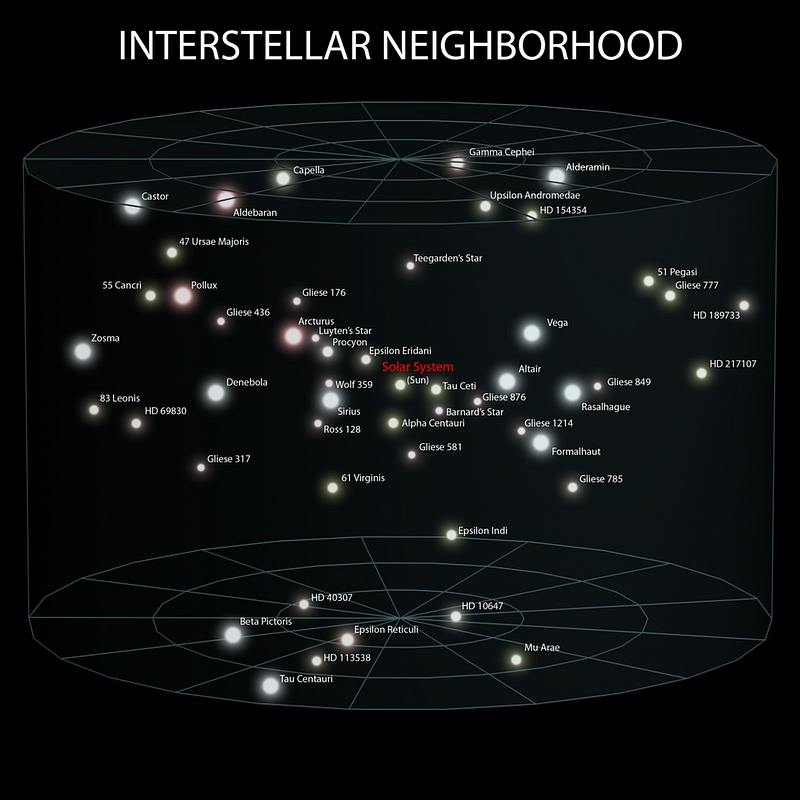
For many centuries, there was no such parallax observed, with the leading explanation being that the stars must be very, very far away. If even the closest stars were so distant that they wouldn’t appear to change their position relative to the more distant stars, even across the diameter of Earth, then we’d have only two options:
- to build telescopes with higher resolutions, capable of measuring positions down to smaller and more precise angles,
- and/or to try and contrive a way to measure longer-baseline distances than even the diameter of the Earth.
The second part got an enormous boost in the 16th and 17th centuries, with the rise of the heliocentric model of the Solar System. If the Earth orbited the Sun, then rather than a baseline of 12,700 kilometers from sunrise to sunset (a 180° rotation about Earth’s axis), we could get a baseline that was much larger, of about 300 million kilometers, from winter solstice to summer solstice (a 180° revolution of the Earth’s orbit around the Sun).
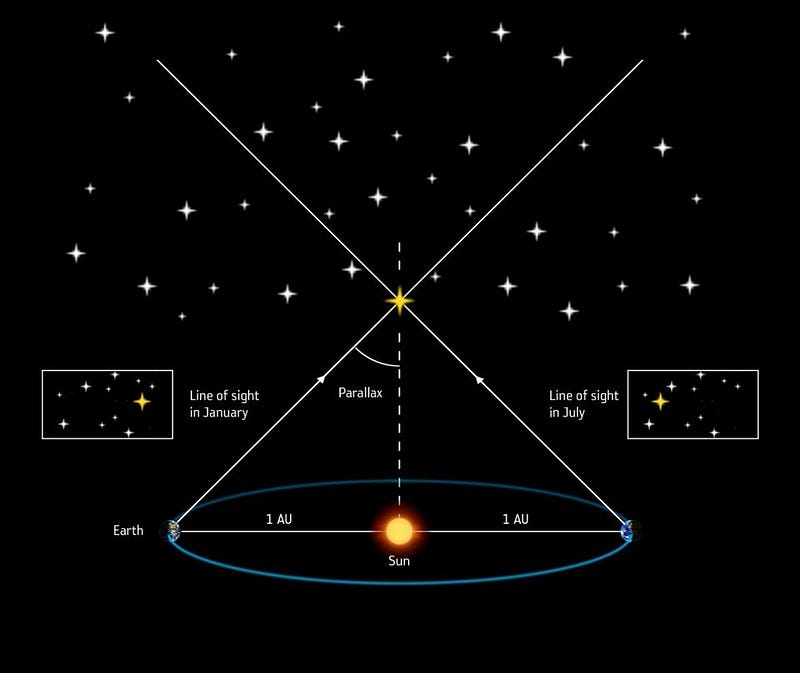
Beginning in the mid-1800s, astronomy had improved sufficiently that the closest stars could begin to have their parallaxes revealed. In 1838, Friedrich Bessel announced the parallax of the star 61 Cygni: the first star known (and quickly confirmed) to have a parallax. Almost immediately afterwards, Friedrich Struve published the parallax of (and hence, also, the distance to) Vega, and Thomas Henderson followed suit with a distance to Alpha Centauri: the brightest member of the closest star system to Earth.
The larger the distance is between your two “eyes,” even if they’re astronomical telescopes instead of your physical eyes, the better job you can do of measuring depth, distance, and seeing the Universe as it truly is: in three-dimensions, rather than as a two-dimensional snapshot. Even today, parallax measurements are the best method we have for uncovering the distance to the nearest stars, with ESA’s Gaia mission the most precise observatory for this method to date.
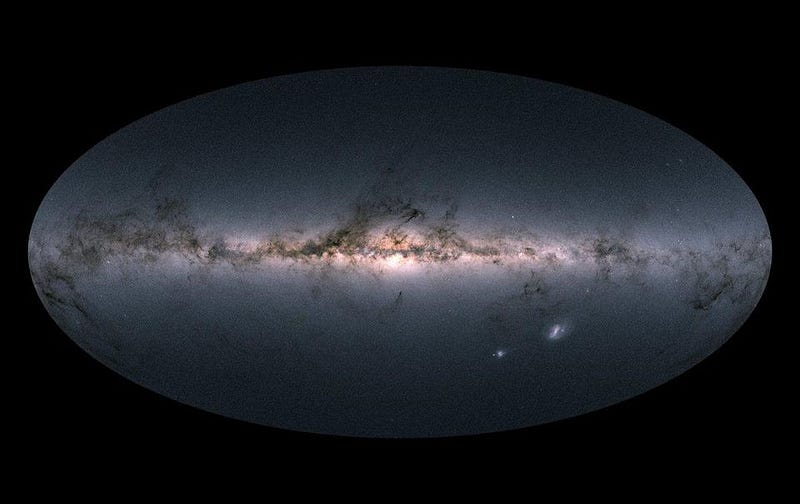
But even Gaia is only in the same orbit that Earth is around the Sun, meaning its maximum baseline for parallax measurements is just 2 AU, where “AU” means astronomical unit, or the average Earth-Sun distance.
What would be far superior, at least in terms of baseline, is if we had an observatory that was very far from Earth, and could measure the stars from an entirely different perspective from us. By extending that baseline to larger distances, across or even beyond the Solar System, we could make the greatest parallax measurements of all-time. By making observations on Earth simultaneously (or as simultaneous as you can get in a Universe governed by relativity), we could minimize a confounding effect that standard parallax measurements suffer from: the fact that the distant stars themselves move over time, even over periods as short as a few months.
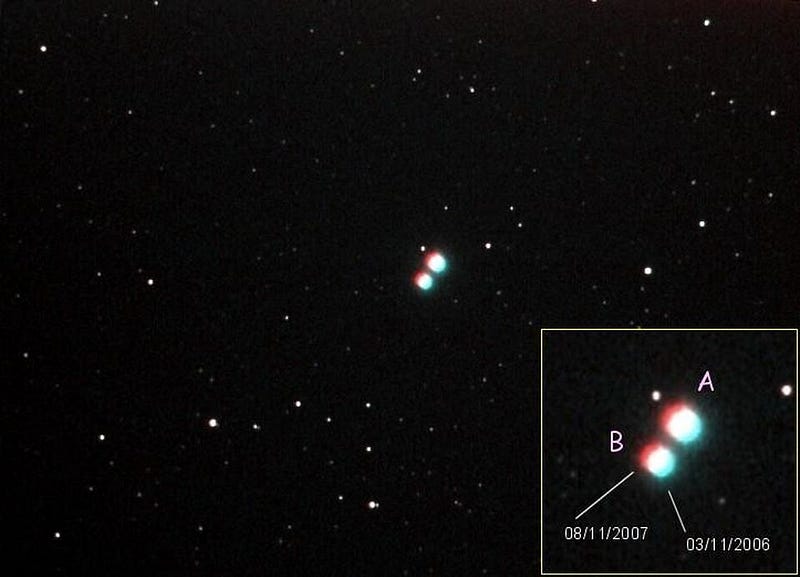
While there are four spacecraft that are very distant from the Sun — Voyager 1 and 2 and Pioneer 10 and 11 — they no longer have the ability to successfully target a distant star and send the data back to Earth. The fifth most distant, however, is NASA’s New Horizons: the spacecraft that famously few past Pluto (and its moons) and, later, the tiny Kuiper belt object, Arrokoth.
In April of 2020, New Horizons will be more than 46 AU from the Sun: close to 8 billion kilometers (5 billion miles). From its perspective, the nearest stars to Earth should appear at a significantly different position in the sky than they do from our terrestrial perspective. If we can make simultaneous measurements of those stars from New Horizons and from Earth, we should be able to detect the largest astronomical parallaxes ever seen in the history of science.
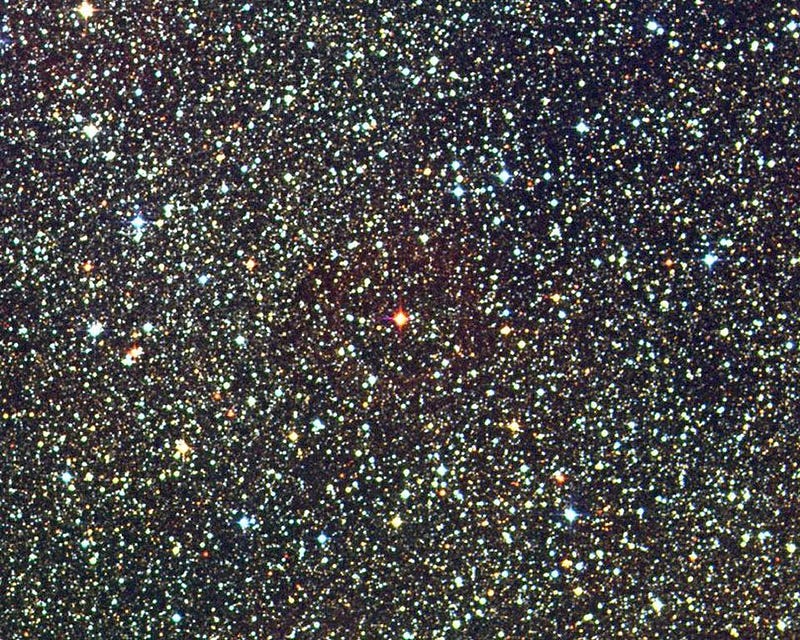
In an exciting moment for science, not only is this actually going to occur, but citizen scientists with large enough telescopes and digital cameras will be able to participate in the experiment itself. On April 22 and 23, New Horizons will point at and take images of two of the nearest faint stars to Earth: Proxima Centauri (at 4.24 light-years) and Wolf 359 (at 7.9 light-years).
If you have a camera-equipped telescope that has an aperture of 6″ (15 cm) or more, chances are you’ll be able to observe these stars. By combining the terrestrial data that ground-based astronomers get with the New Horizons data, the longest-baseline 3D images ever will be constructed. The result, according to astronomer Tod Lauer, will be spectacular.
For all of history, the fixed stars in the night sky have served as navigation markers. As we voyage out of the solar system and into interstellar space, how the nearer stars shift can serve as a new way to navigate. We will see this for the first time with New Horizons.
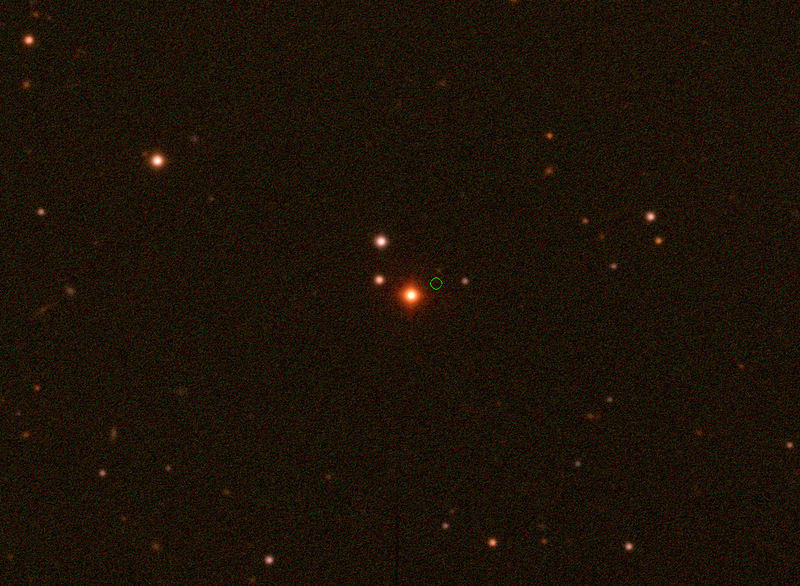
By imaging two of the nearest stars to Earth from our planet and NASA’s New Horizons spacecraft, humanity will construct 3D images of the stars as though we had two eyes that were nearly 5 billion miles (8 billion kilometers) apart from one another. Not only will it spectacularly demonstrate how far NASA’s New Horizons has traveled, but it will give us a tiny glimpse into the humbling fact of our insignificant view of the cosmos.
We all know that the relative positions of stars that we see here on Earth are unique to our current perspective: our place in space and time. From any other vantage point, the stars and constellations would look dramatically different, as every solar system has a different night sky. For the first time, we’ll get to see the Universe with the depth perception of an unprecedented giant: one whose eyes are larger than the Sun-Pluto distance. The images, slated for release in May, will give us a view of the Universe as never before.
Ethan Siegel is the author of Beyond the Galaxy and Treknology. You can pre-order his third book, currently in development: the Encyclopaedia Cosmologica.