Why Humans Should Be Thankful That Our Universe Has Dark Matter
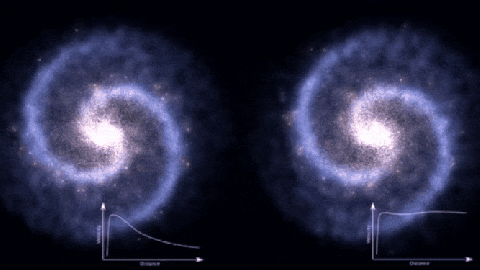
Without this one ingredient, there wouldn’t be enough ‘glue’ to hold the Universe together.
Of all the things in the Universe to be thankful for — the stars, planets, atoms, molecules, and more that came together and made our existence possible — it seems odd that dark matter would be included. Even here in our own Solar System, dark matter might be present, but even its gravitational effects are totally negligible, contributing less than the dwarf planet Ceres does to all the orbits of the planets, moons, asteroids and Kuiper belt objects.
And yet, without dark matter, the Universe as we know it wouldn’t exist the way it does. Stars would be extremely rare entities in the Universe, and large galaxies with Sun-like stars and Earth-like planets would be all but impossible. Dark matter enabled the Universe to give rise to us, and without it, we wouldn’t be here. Here’s the cosmic story that every one of us should be thankful for.
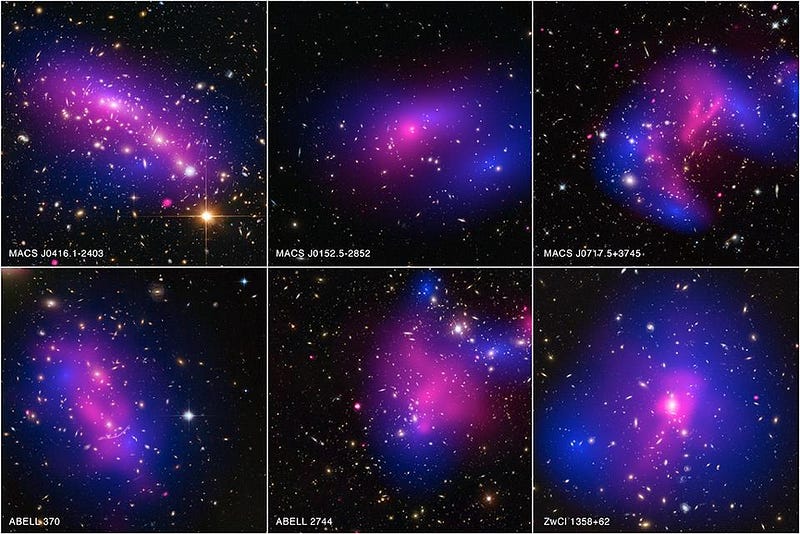
On astronomical scales, particularly on large, cosmic ones, the observational evidence for dark matter is overwhelming. Without its gravitational effects:
- galaxies wouldn’t rotate as they’re observed to,
- individual galaxies would move too quickly to remain in bound clusters,
- colliding clusters of galaxies (above) wouldn’t show a separation between the normal (X-ray emitting) matter and the gravitational lensing (driven by dark matter) signals,
- the cosmic web wouldn’t show the clustering properties it exhibits,
- and the fluctuation pattern in the cosmic microwave background would look tremendously different.
The suite of evidence supporting dark matter’s existence is overwhelming. But what isn’t generally appreciated is that if our Universe didn’t have dark matter, our galaxy couldn’t have held onto the raw ingredients that made life like humans and planets like Earth possible.
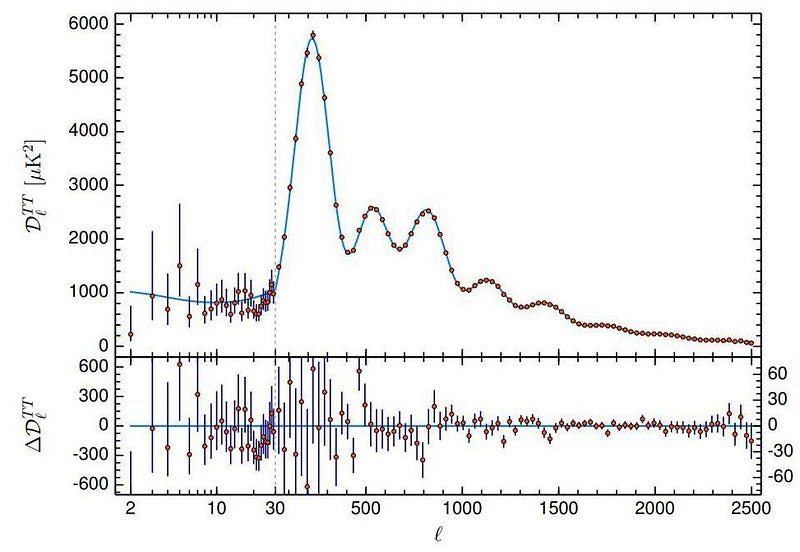
Whatever dark matter is, it must have existed in roughly the same amount over the course of nearly the entire Universe. Observationally, the signatures of dark matter that show up in the cosmic microwave background — which is required to explain the existence of about half of the “acoustic peak” features in the graph above — tell us that dark matter must have been present from when the Universe was a few thousand years old, at most.
Most models of making dark matter are only plausible in the first fraction-of-a-second after the Big Bang, even though it won’t become cosmologically important until later. But with the seeds of structure in place, arising from both normal matter and dark matter together, it’s only a matter of time and gravity until matter collapses into dense enough regions to form the first stars and proto-galaxies in the Universe.

Whether your Universe had dark matter or not wouldn’t matter all that much, from a high-level point of view, when it comes to the first stars. These stars will be form when large molecular clouds of (mostly hydrogen and helium) gas collapse, which takes tens to hundreds of millions of years in either case. The first stars, because of their utter lack of heavier elements, radiate and cool differently than modern stars; the average “first star” is about 25 times as massive as the average star formed today.
These massive stars will be bright and short-lived, burning through their core’s fuel perhaps a thousand times faster than our Sun does. When the core runs out of fuel, these stars will contract, heat up, and burn through increasingly heavier elements. At some critical moment, the radiation pressure will drop as the fuel runs low, and the core will collapse under its own gravity, triggering a supernova explosion.
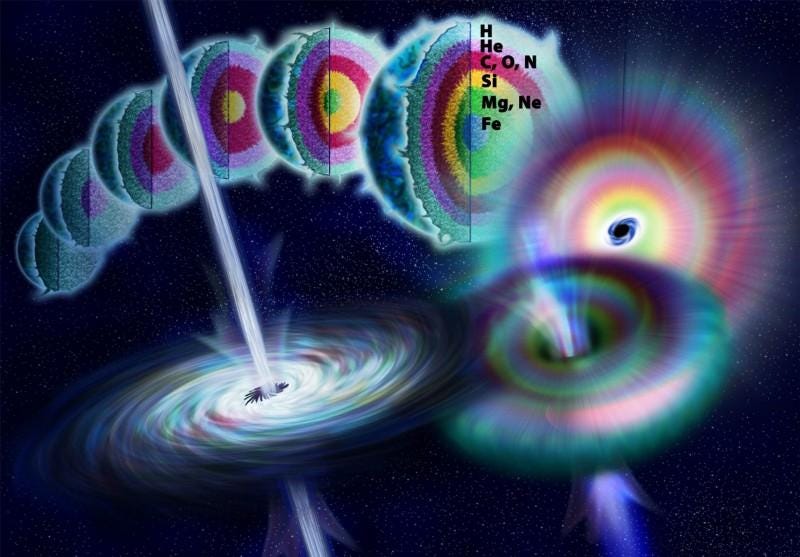
These supernovae happen rapidly and in waves wherever the first stars were. But this is where dark matter’s presence is so important to our existence: these first supernovae are where a huge percentage of our heavy elements come from. The elements required for biology, including carbon, oxygen, nitrogen, phosphorous and sulphur, require these massive stars to live, die, and recycle their cosmic insides into the next generation of stars.
In order to meaningfully contribute to forming rocky planets and organic compounds, those heavy elements need to not only be created (which they are in these stars and cataclysms, regardless of dark matter), but they need to be retained and put to good use. This is where, in these first, early star clusters, dark matter plays such a vital role.

We’ve observed supernova explosions in great detail, and one of the lessons we’ve learned is how quickly this material gets ejected from the stars that undergo their death throes in this manner. Typical speeds are substantial: on the order of 1,000 km/s, or a few tenths-of-a-percent of the speed of light. In fact, the Cassiopeia A supernova remnant, dating back to a 17th century explosion here in our Milky Way, has ejecta leaving it between 5,000 and 14,500 km/s!
For comparison, our own Sun orbits the Milky Way at a relatively paltry velocity of only 220 km/s, and if it were moving substantially faster (say, three times as fast), it would escape from our galaxy’s gravitational pull. The star clusters and early proto-galaxies in the young Universe are much less massive and much easier to gravitationally escape from. If we’re not careful, all the elements we worked so hard to create can get thrown out of these galaxies, knocking us back to square one in our Universe’s quest to form planets and life.
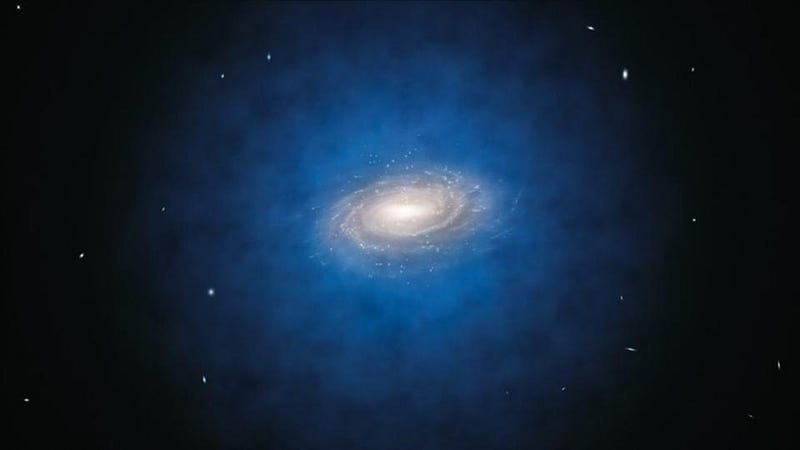
There’s other matter (like neutral gas) surrounding a supernova in the early Universe, but even the effect of smashing into all of that material is not enough to allow these young star clusters to hold onto most of their supernova ejecta. These low mass objects, tenuously bound together by their mutual gravity, will be unable to hold onto even modestly fast, energetic ejecta.
But now, if you add dark matter, the story changes dramatically. Just as our galaxy has an enormous, diffuse halo of dark matter surrounding it, so should all large, gravitationally-driven structures of all sizes and scales in the Universe. Because of the enormous gravitational influence of this dark matter, and the fact that it continues to act on normal matter that attempts to escape out to much larger distance scales than the normal matter itself extends for, dark matter gives the Universe a chance to hold its structures together.
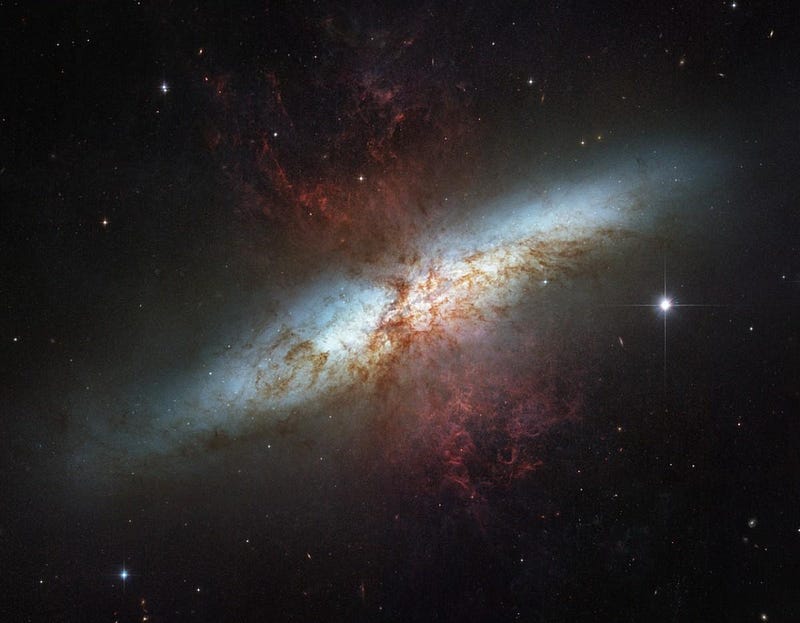
If it weren’t for the additional gravitation that a massive dark matter halo provides, the overwhelming amount of material ejected from a supernova would escape from galaxies forever. The same story would be true for neutron star mergers, white dwarf collisions, and other cataclysms that produce heavy elements in substantial quantities. Without these advanced building blocks remaining in what will become a galaxy, complex molecules and rocky planets would still remain impossible.
When the next generation of stars attempted to form, it would have only paltry amounts of heavy elements if dark matter weren’t present. Whereas in our dark matter-rich Universe, these heavy elements build up substantially over time — they comprise about 1–2% of the total normal matter at present — in a dark matter-free Universe, the abundance of heavy elements would be negligible.
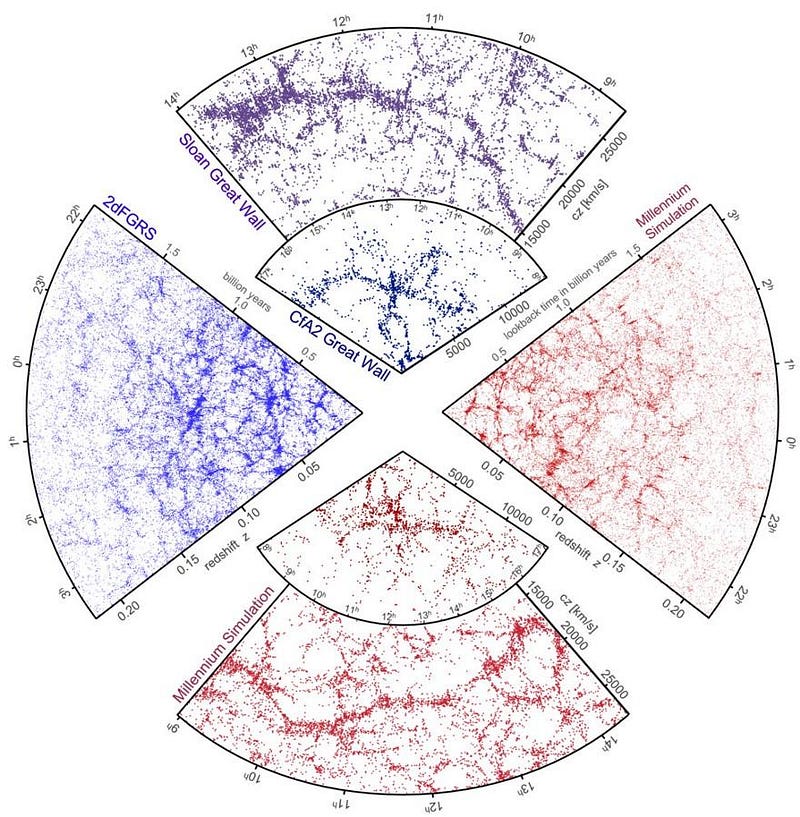
Furthermore, the standard scenario of a large-scale cosmic web growing and funneling matter into it wouldn’t work the same way either, as a Universe without dark matter doesn’t have that same material to drive the formation of structure. With normal matter alone, smaller-scale structures would be washed out, and the bigger galaxies that formed would be extremely sparse and few in number throughout the cosmos.
Even in these astronomical rarities, the occasional large galaxy would still be unable to hold onto its heavier elements, even in the most central regions in the most massive galaxies. Even if a small amount of these elements were retained, these environments are presently thought to be uninhabitable due to large amounts of cosmic radiation and nearby cataclysms.
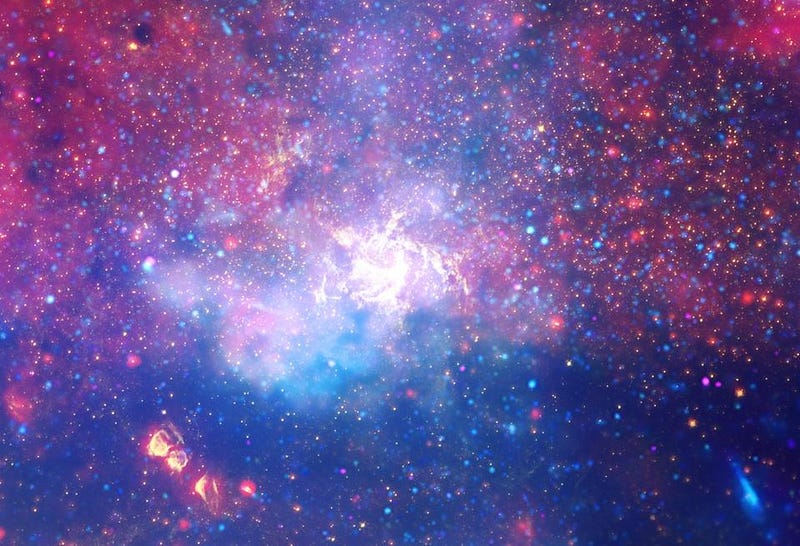
In a Universe without dark matter, we might still have stars and galaxies, but the only planets would be gas giant worlds, with no rocky ones to speak of. Without carbon, there are no organic molecules; without oxygen, there is no liquid water; without a whole slew of elements from the periodic table, biochemical life would be completely impossible.
Only with the presence of massive dark matter halos, surrounding galaxies and driving the growth of the cosmic web, can a planet like Earth or carbon-based life like we find terrestrially be formed. As we’ve come to understand what makes up our Universe and how it grew to be this way, one inescapable conclusion emerges: dark matter is fundamentally necessary for life to arise. Without it, the chemistry that underlies all life could never have occurred. Today and every day, we should be thankful for every part of the cosmic story that allowed us to exist. Even dark matter.
Ethan Siegel is the author of Beyond the Galaxy and Treknology. You can pre-order his third book, currently in development: the Encyclopaedia Cosmologica.