At Last, Scientists Have Found The Galaxy’s Missing Exoplanets: Cold Gas Giants

Our outer Solar System, from Jupiter to Neptune, isn’t unique after all.
In the early 1990s, scientists began detecting the first planets orbiting stars other than the Sun: exoplanets. The easiest ones to see had the largest masses and the shortest orbits, as those are the planets with the greatest observable effects on their parent stars. The second types of planets were at the other extreme, massive enough to emit their own infrared light but so distant from their star that they could be independently resolved by a powerful enough telescope.
Today, there are over 4,000 known exoplanets, but the overwhelming majority either orbit very close to or very far from their parent star. At long last, however, a team of scientists has discovered a bevy of those missing worlds: at the same distance our own Solar System’s gas giants orbit. Here’s how they did it.
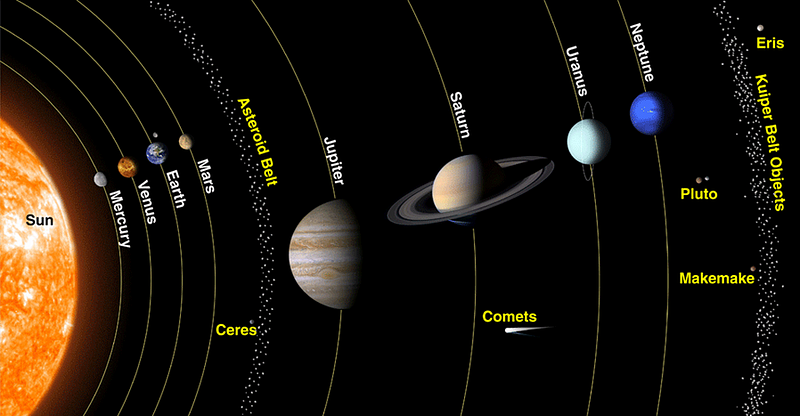
When you look at a star, you’re not simply seeing the light it emits from one constant, point-like surface. Instead, there’s a lot of physics going on inside that contributes to what you see.
- the star itself isn’t a solid surface, but emits the light you see for many layers going down hundreds or even thousands of kilometers,
- the star itself rotates, meaning one side moves towards you and the other away from you,
- the star has planets that move around it, occasionally blocking a portion of its light,
- the orbiting planets also gravitationally tug on the star, causing it to periodically “wobble” in time with the planet orbiting it,
- and the star moves throughout the galaxy, changing its motion relative to us.
All of these, in some way, matter for detecting planets around a star.
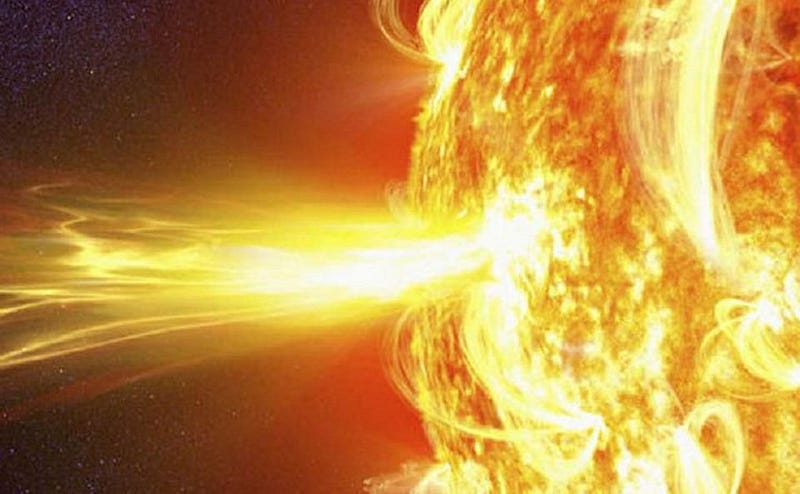
That first point, which might seem the least important, is actually vital to the way we detect and confirm exoplanets. Our Sun, like all stars, is hotter towards the core and cooler towards the limb. At the hottest temperatures, all the atoms inside the star are fully ionized, but as you move to the outer, cooler portions, electrons remain in bound states.
With the energy relentlessly coming from its environment, these electrons can move to different orbitals, absorbing a portion of the star’s energy. When they do, they leave a characteristic signature in the star’s light spectrum: an absorption feature. When we look at the absorption lines of stars, they can tell us what elements they’re made of, what temperature they’re emitting at, and how quickly they’re moving, both rotationally and with respect to our motion.
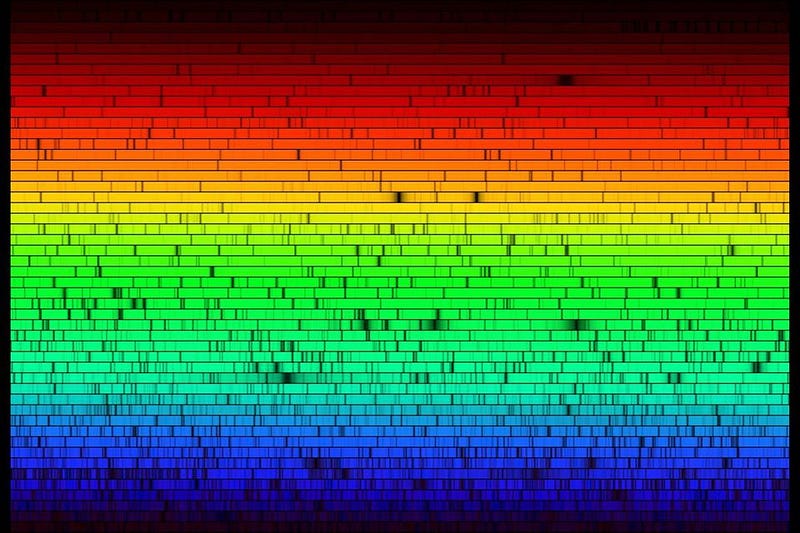
The more accurately you can measure the wavelength of a particular absorption feature, the more accurately you can determine the star’s velocity relative to your line-of-sight. If the star you’re observing moves towards you, that light gets shifted towards shorter wavelengths: a blueshift. Similarly, if the star you’re monitoring is moving away from you, that light will be shifted towards longer wavelengths: a redshift.
This is simply the Doppler shift, which occurs for all waves. Whenever there’s relative motion between the source and the observer, the waves received will either be stretched towards longer or shorter wavelengths compared to what was emitted. This is true for sound waves when the ice cream truck goes by, and it’s equally true for light waves when we observe another star.
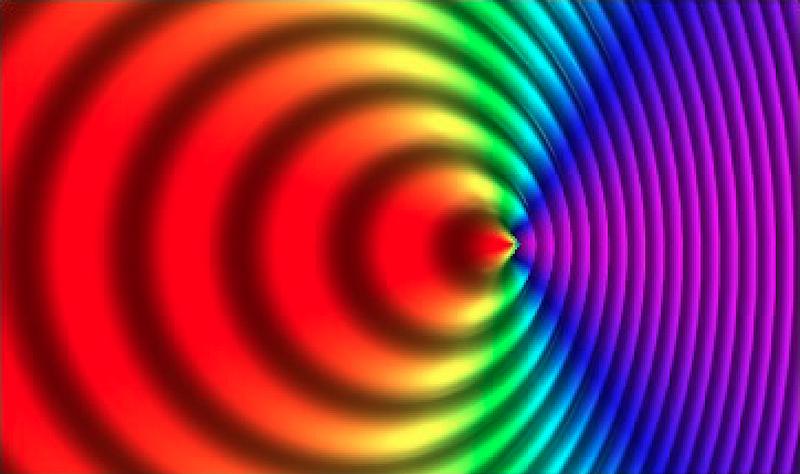
When the first detection of exoplanets around stars was announced, it came from an extraordinary application of this property of matter and light. If you had an isolated star that moved through space, the wavelength of these absorption lines would only change over long periods of time: as the star we were watching moved relative to our Sun in the galaxy.
But if the star weren’t isolated, but rather had planets orbiting it, those planets would cause the star to wobble in its orbit. As the planet moved in an ellipse around the star, the star would similarly move in a (much smaller) ellipse in time with the planet: keeping their mutual center-of-mass in the same place.
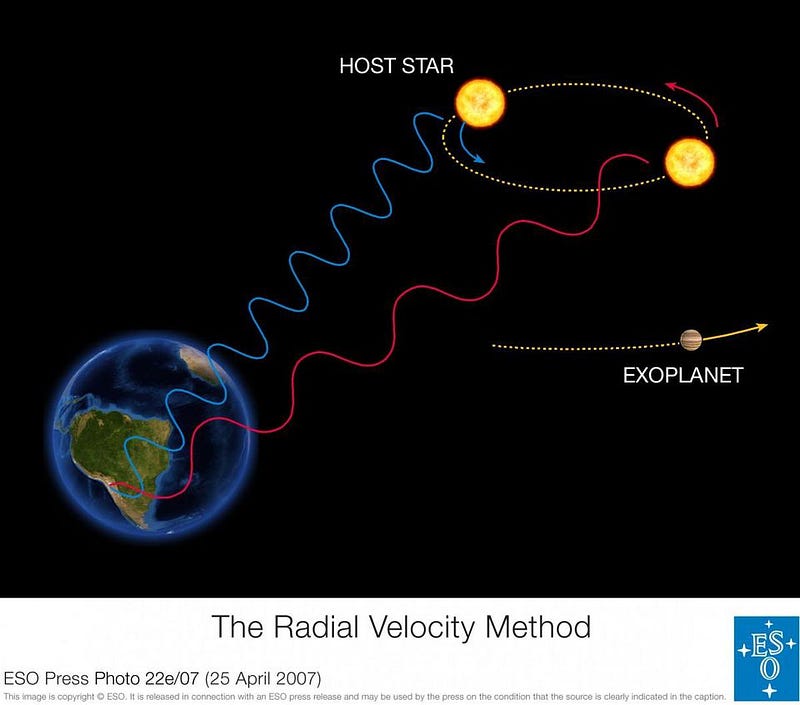
In a system with multiple planets, these patterns would simply superimpose themselves atop one another; there would be a separate signal for every planet you could identify. The strongest signals would come from the most massive planets, and the fastest signals — from the planets orbiting most closely to their stars — would be the easiest to identify.
These are the properties that the very first exoplanets had: the so-called “hot Jupiters” of the galaxy. They were the easiest to find because, with very large masses, they could change the motion of their stars by hundreds or even thousands of meters-per-second. Similarly, with short periods and close orbital distances, many cycles of sinusoidal motion could be revealed with only a few weeks or months of observations. Massive, inner worlds are the easiest to find.
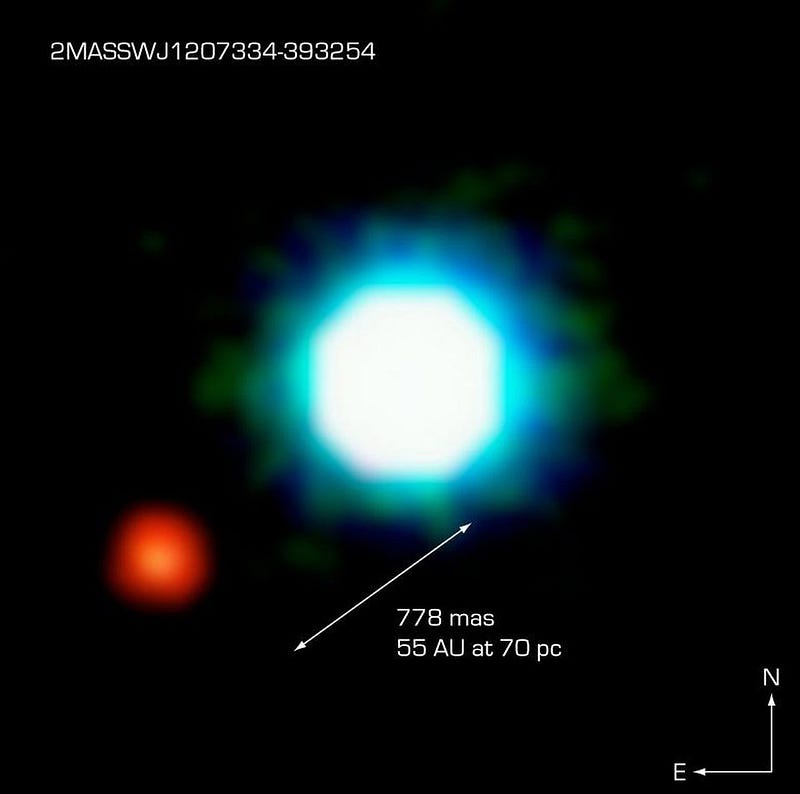
On the complete opposite end of the spectrum, some planets that are equal to or greater than Jupiter’s mass are extremely well-separated from their star: more distant than even Neptune is from the Sun. When you encounter a system such as this, the massive planet is so hot in its core that it can emit more infrared radiation than it reflects from the star it orbits.
With a large enough separation, telescopes like Hubble can resolve both the main star and its large planetary companion. These two locations — the inner solar system and the extreme outer solar system — were the only places where we had found planets up until the explosion of exoplanets brought about by NASA’s Kepler spacecraft. Until then, it was only high-mass planets, and only in the places where they aren’t found in our own Solar System.
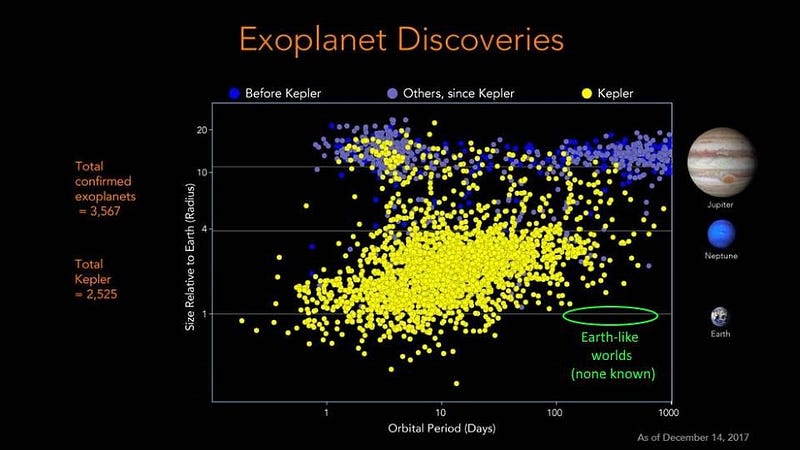
Kepler brought about a revolution because it used an entirely different method: the transit method. When a planet passes in front of its parent star, relative to our line-of-sight, it blocks a tiny portion of the star’s light, revealing its presence to us. When the same planet transits its star multiple times, we can learn properties like its radius, orbital period, and the orbital distance from its star.
But this was limited, too. While it was capable of revealing very low-mass planets compared to the earlier (stellar wobble/radial velocity) method, the primary mission only lasted for three years. This meant that any planet that took longer than about a year to orbit its star couldn’t be seen by Kepler. Ditto for any planet that didn’t happen to block its star’s light from our perspective, which you’re less likely to get the farther away from the star you look.
The intermediate distance planets, at the distance of Jupiter and beyond, were still elusive.
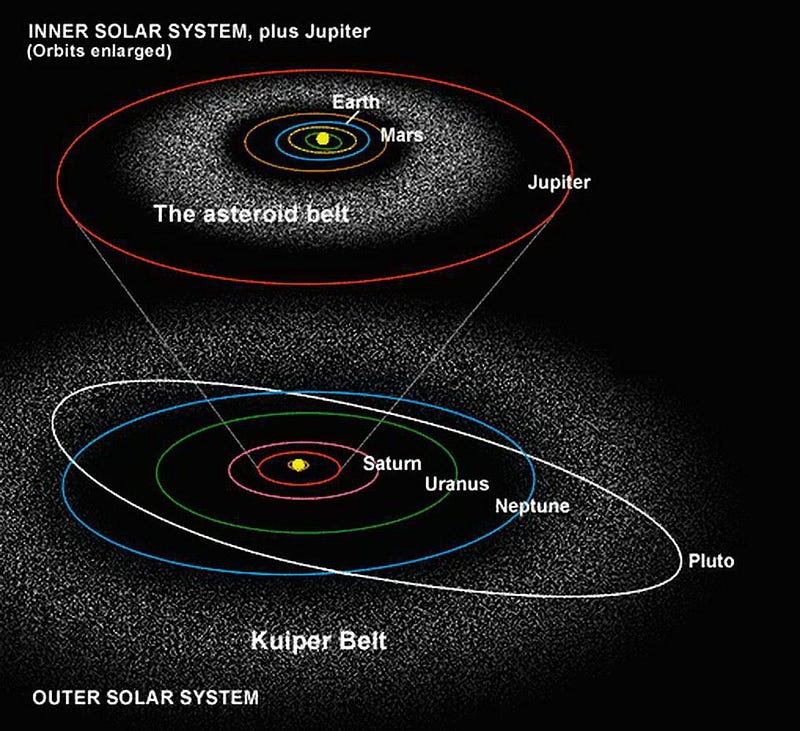
That’s where a dedicated, long-period study of stars can come in to fill in that gap. A large team of scientists, led by Emily Rickman, conducted an enormous survey using the CORALIE spectrograph at La Silla observatory. They measured the light coming from a large number of stars within about 170 light-years on a nearly continuous basis, beginning in 1998.
By using the same instrument and leaving virtually no long-term gaps in the data, long-term, precise Doppler measurements finally became possible. A total of five brand new planets, one confirmation of a suggested planet, and three updated planets were announced in this latest study, bringing the total number of Jupiter-or-larger planets beyond the Jupiter-Sun distance up to 26. It shows us what we’d always hoped for: that our Solar System isn’t so unusual in the Universe; it’s just difficult to observe and detect planets like the ones we have.
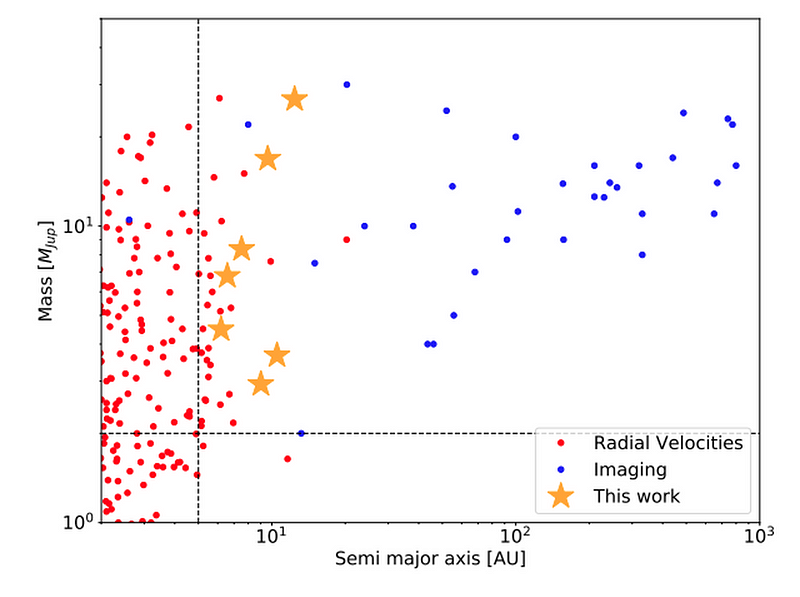
Even with these latest results, however, we still aren’t sensitive to the worlds we actually have in our Solar System. While the periods of these new worlds range from 15 to 40 years, even the smallest one is nearly three times as massive as Jupiter. Until we develop more sensitive measurement capabilities and make those observations over decadal timescales, real-life Jupiters, Saturns, Uranuses and Neptunes will remain undetected.
Our view of the Universe will always be incomplete, as the techniques we develop will always be inherently biased to favor detections in one type of system. But the irreplaceable asset that will open up more of the Universe to us isn’t technique-based at all; it’s simply an increase in observing time. With longer and more sensitive observations of stars, closely tracking their motions, we can reveal lower-mass planets and worlds at greater distances.
This is true of both the stellar wobble/radial velocity method and also the transit method, which hopefully will reveal even smaller-mass worlds with longer periods. There is still so much to learn about the Universe, but every step we take brings us closer to understanding the ultimate truths about reality. Although we might have worried that our Solar System was in some way unusual, we now know one more way we’re not. Having gas giant worlds in the outer solar system may pose a challenge for detections, but those worlds are out there and relatively common. Perhaps, then, so are solar systems like our own.
Ethan Siegel is the author of Beyond the Galaxy and Treknology. You can pre-order his third book, currently in development: the Encyclopaedia Cosmologica.