The Drake Equation Is Broken; Here’s How To Fix It
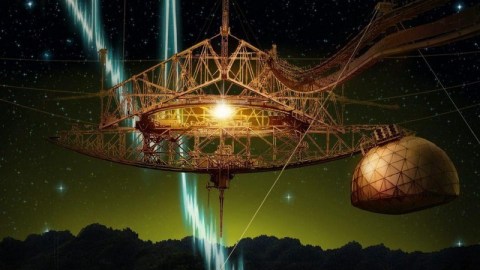
In the aftermath of everything we’ve learned about what’s in the Universe, we can make much better estimates of how many alien civilizations are out there.
In 1961, scientist Frank Drake wrote down a simple-looking equation for estimating the number of active, technologically-advanced, communicating civilizations in the Milky Way. From first principles, there was no good way to simply estimate a number, but Drake had the brilliant idea of writing down a large number of parameters that could be estimated, which you would then multiply together. If your numbers were accurate, you’d arrive at an accurate figure for the number of technologically advanced civilizations that humanity could communicate with, within our own galaxy, at any given moment. It’s a brilliant idea in concept, but one that’s become less and less useful as we’ve learned more about our Universe. As it stands today, the Drake equation is broken, but we know enough about the Universe to construct an even better framework.
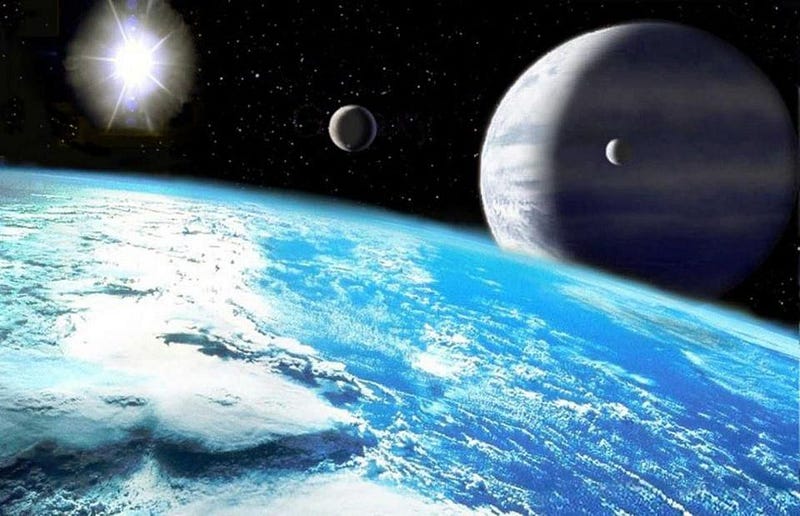
The Drake equation, to be specific, said that the number of civilizations (N) we have at any given time within our galaxy, is equal to the product of seven different unknown quantities from astronomy, geology, biology, and anthropology, each of which build off of the previous element. They are:
- R_∗, the average rate of star formation,
- f_p, the fraction of stars with planets,
- n_e the average number stars-with-planets that have one that could support life,
- f_l, the fraction of those planets that developed life,
- f_i, the fraction of life-bearing planets that developed intelligent life,
- f_c, the fraction of these intelligence-having planets that are technologically communicative across interstellar space, and
- L, the length of time such a civilization can broadcast-or-listen.
Multiply these numbers all together, in theory, and that will give you the number of technologically advanced, broadcasting civilizations we have in the Milky Way today.
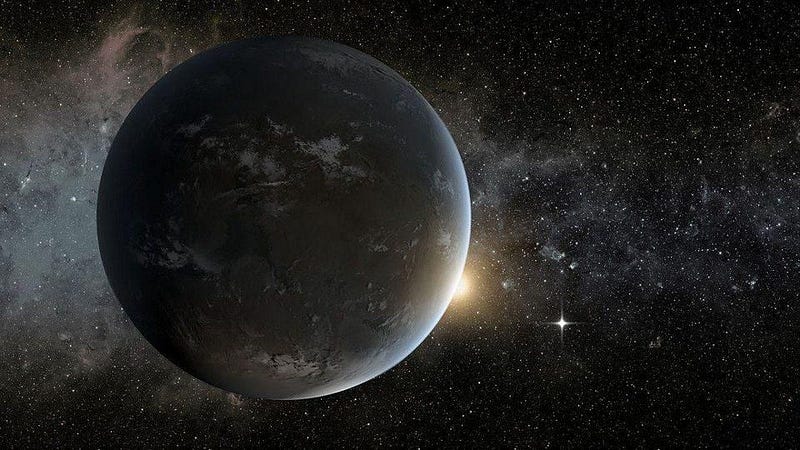
Only, there are huge problems with this setup. There are a number of unspoken assumptions that simply writing down the equation this way makes, that simply don’t reflect reality. Problems for its modern-day usefulness include:
- The fact that the equation was written before the Big Bang was validated and the Steady State model was disfavored.
- The equation assumes that only one planet per star system could support life.
- That intelligent, technologically advanced life will never spread to other worlds.
- And that broadcasting-and-listening-for radio signals is the method by which an intelligent species would choose to communicate across interstellar space.
That last assumption, in particular, was the motivation for SETI — the search for extraterrestrial intelligence (with radio dishes) — which has, of course, come up empty.
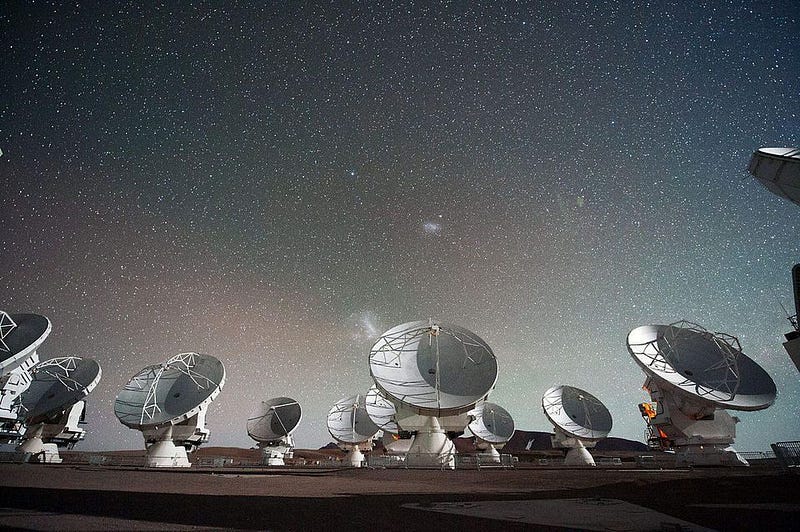
This doesn’t mean, however, that there aren’t other worlds out there with intelligent life on them! Despite our uncertainties about what’s out there or whether/how they might attempt to search for or contact us, the possibility of intelligent, communicative, or spacefaring extraterrestrials is one of tremendous interest to not only scientists, but all of humanity. Many of the steps of the Drake equation may be problematic, and they contain the major issue that there are huge uncertainties associated with them: so large that they render any conclusion about N, the number of civilizations within our galaxy, meaningless. But it’s 2018 now, and there are a huge number of things we know about our galaxy and our Universe that we didn’t know in 1961. Here’s a better approach.
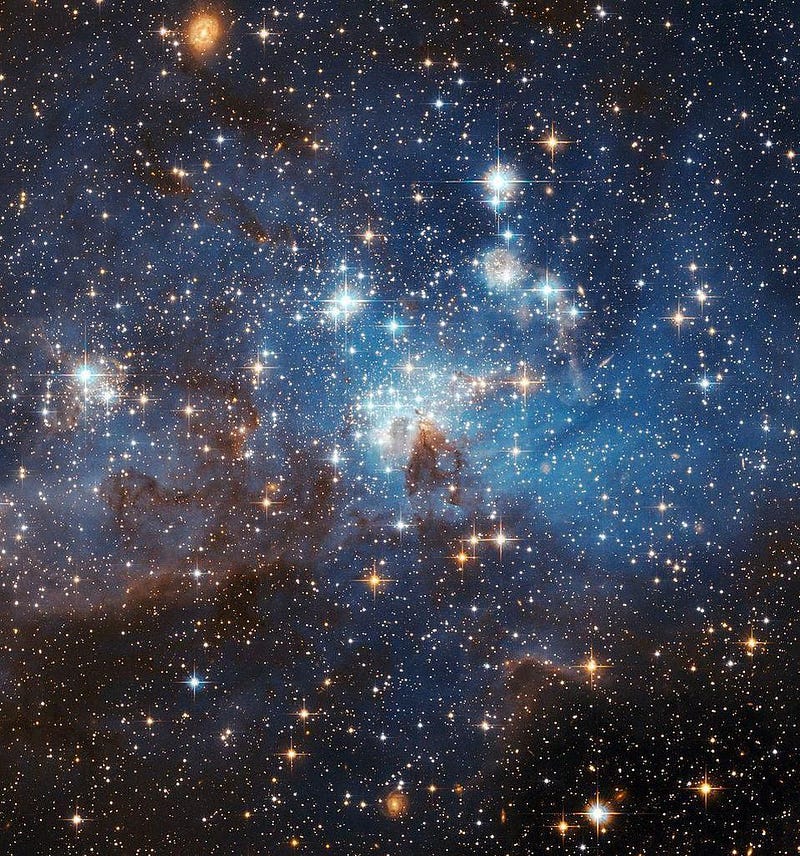
1.) N_s: the number of stars in our galaxy. Why estimate the rate of star formation when we can simply look at the number of stars we have today? We know how large our galaxy is, how thick it is, how large the central bulge is, and what their mass distribution is. Based on what we can observe with extremely powerful all-sky and pencil-beam (where you look at one narrow region very deeply) surveys, we can simply state that there are between 200 and 400 billion stars in our galaxy. An uncertainty that’s only a factor of 2 is pretty good, and tells us that we have a very optimistic starting point: each star has a chance for success. Let’s pick the larger number here.
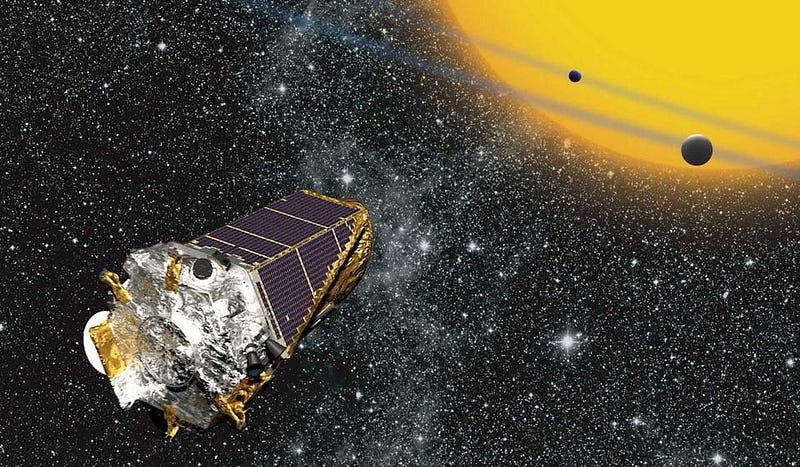
2.) f_p: the fraction of stars with planets. This is one we can keep from the original Drake equation, but in the aftermath of Kepler, it isn’t all that interesting. Why? Because it’s close to 100%! The fraction of stars with planets around them, based on the number of stars we’ve surveyed and what we’ve learned about them, is somewhere in the ballpark of at least 80%. To say “the fraction of stars with planets” is 1 is a nice, easy victory for the optimists out there.
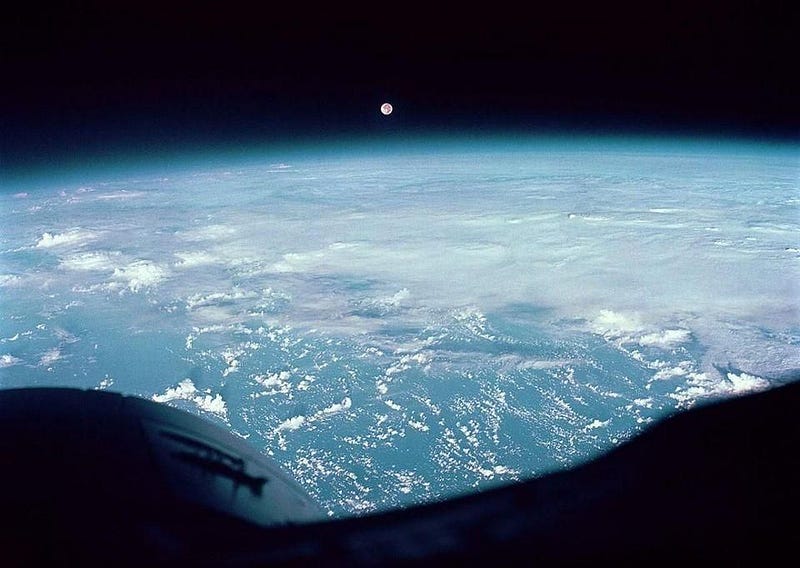
3.) f_H: the fraction of stars with the right conditions for habitability. This gets more interesting now! Of the major classes of stars, how many of them have worlds that could support life? A star like our Sun — with our Sun’s mass, radius, and lifetime — could do it, as evidenced by our existence. But what about a more massive star? At some point, they’ll be massive enough to burn through their fuel too quickly, and intelligent life could never arise.
On the other end, a low-mass star may be too unstable, flaring and blowing off a planet’s atmosphere, or with little enough ultraviolet light that life cannot arise. We might worry about if there are enough heavy elements to support life on a world, or if a certain location in the galaxy renders the environment too chaotic for life. These may be unknowns, but we can probably safely say that at least a quarter, or 25%, of stars in our galaxy can have a potentially habitable planet.
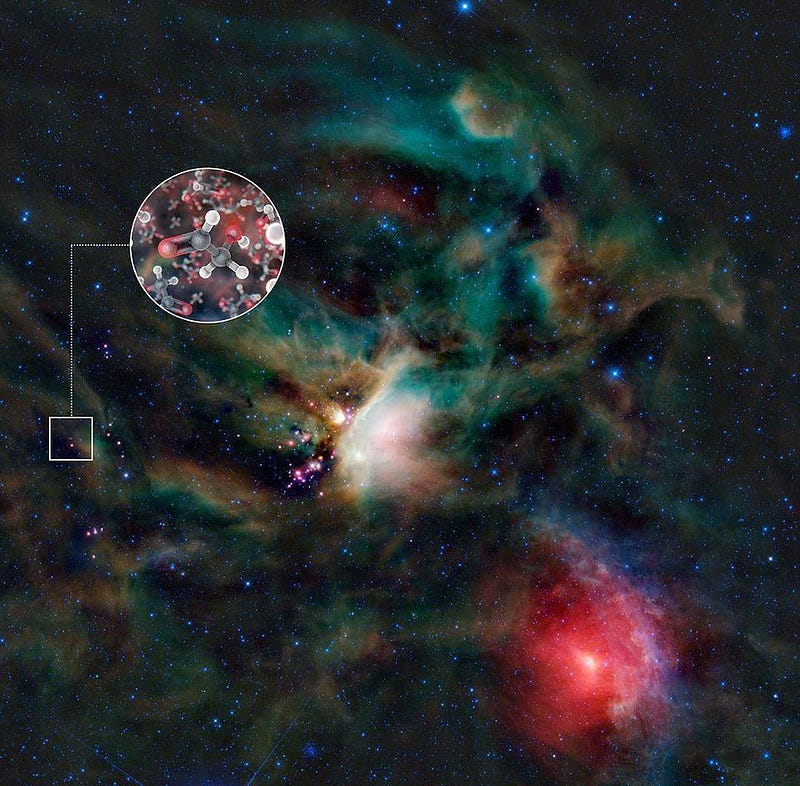
4.) n_p: the number of worlds around habitable stars with the right conditions for life. This is something we’ve learned a tremendous amount about from our exoplanet studies, but tremendous questions remain. What makes a world habitable? In the early solar system, Venus, Earth, and Mars all had similar conditions. In the outer Solar System, worlds like Enceladus and Europa, with sub-surface oceans, may have underwater life. In systems with gas giants at Earth-like locations, large moons could see life arise on them. Although the uncertainties are very large here, I think it’s a fair estimate to say that of the stars which can have a potentially habitable world, on average there will be one world that clearly has the best chance for life. That’s the world we’re interested in, and so we’ll say n_p= 1.
At this point, by the way, we can multiply those first four numbers together to get an estimate for the number of worlds with good chances at life within our galaxy: 100 billion. That’s a promising start.
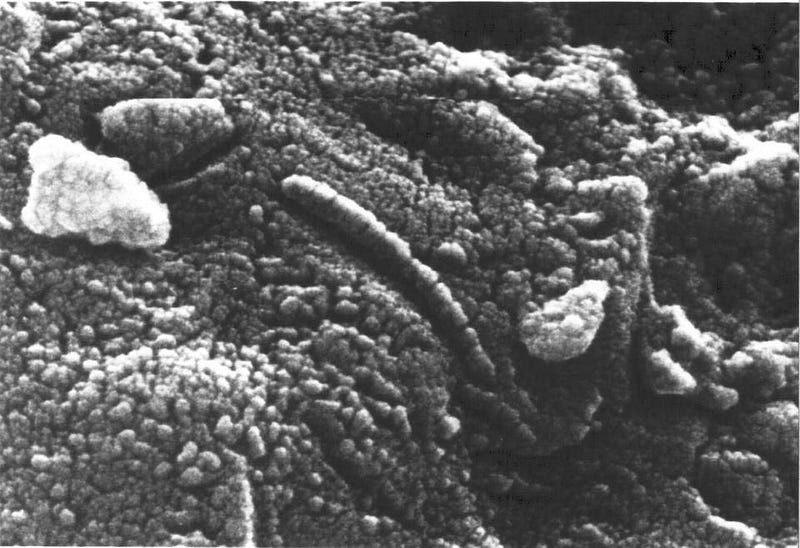
5.) f_l: the fraction of these worlds where life arises. This is a great time to line up with Drake again, because this is one of the great unknown questions in the search for life beyond Earth. Of all the potentially habitable worlds, how many of them take that first incredible step, where life arises from non-life? Or, if primitive life originates in interstellar space, how many worlds see life take hold on the surface, in the oceans, or in the atmosphere? We don’t even know the answer for our own Solar System, where it’s arguable that we may have as many as 8 other worlds where life arose at some point. Life may be common; optimistically, it may have a 10% chance of arising from non-life. Or, alternatively, it could be exceedingly rare: a one-in-a-million shot or worse.
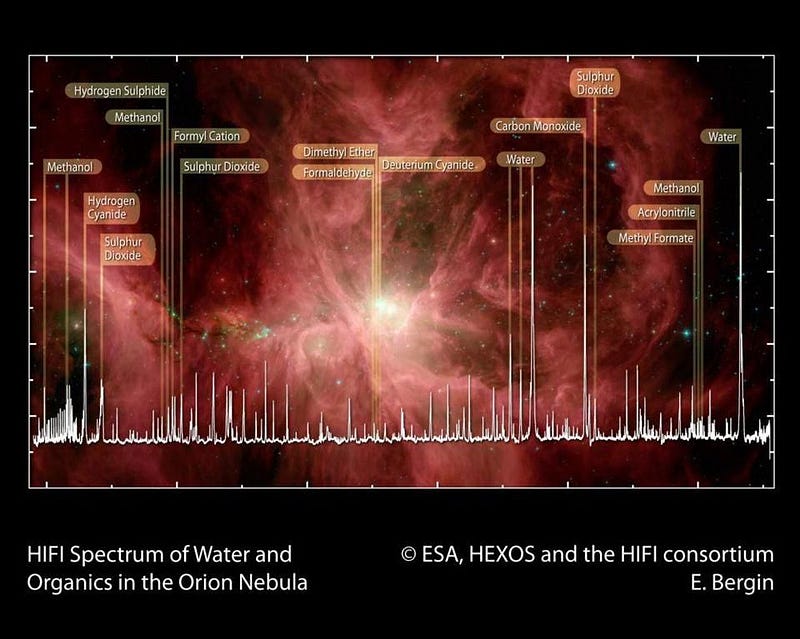
The uncertainties here are huge, and any number that you can pick is as ill-motivated as any other. Someday in the future, we’ll have the capability of performing our first tests, however. When our telescope technology enables us to determine the atmospheric contents of worlds, we can look for the presence or absence of biosignatures like methane, molecular oxygen, and carbon dioxide. It will be indirect evidence, but it should be an incredible step towards inferring whether worlds have life on them or not. If we say there’s a 1-in-10,000 chance that a potentially habitable world has life on it, as good a guess as any, that means there are 10 million worlds in the Milky Way where life exists.
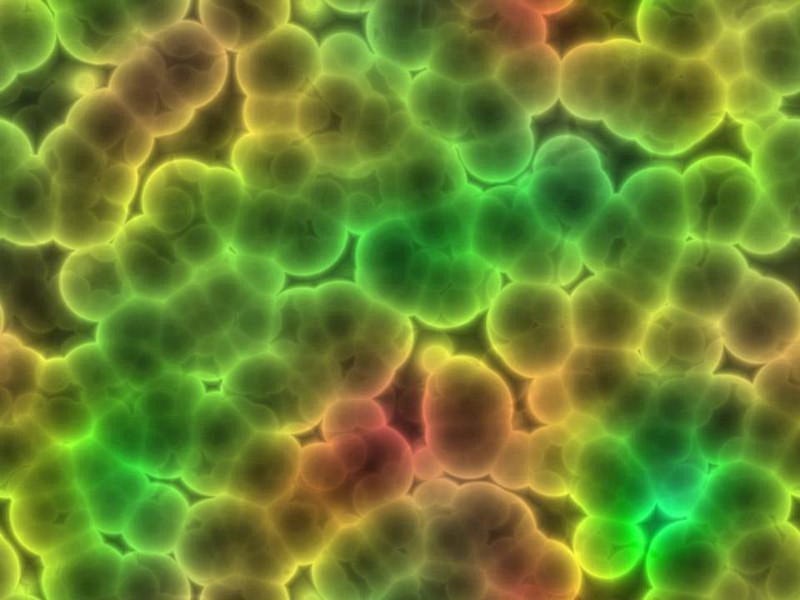
6.) f_x: the fraction of life-having worlds with complex, differentiated organisms. Defining life as “intelligent” or not is a hazy prospect at best, as even the top scientists still argue over the classification of dolphins, great apes, octopi, and many other organisms as intelligent or not. What no one will argue about, however, is whether an organism is complex and differentiated: with different body parts with different functions and structures, in a macroscopic, multicellular arrangement. It took billions of years of life thriving on Earth until we evolved the first multicellular organism, and then hundreds of millions of years more until we developed gender in reproduction; without both, out-competing single-celled life would be impossible, as they’d out-evolve the larger forms of life.
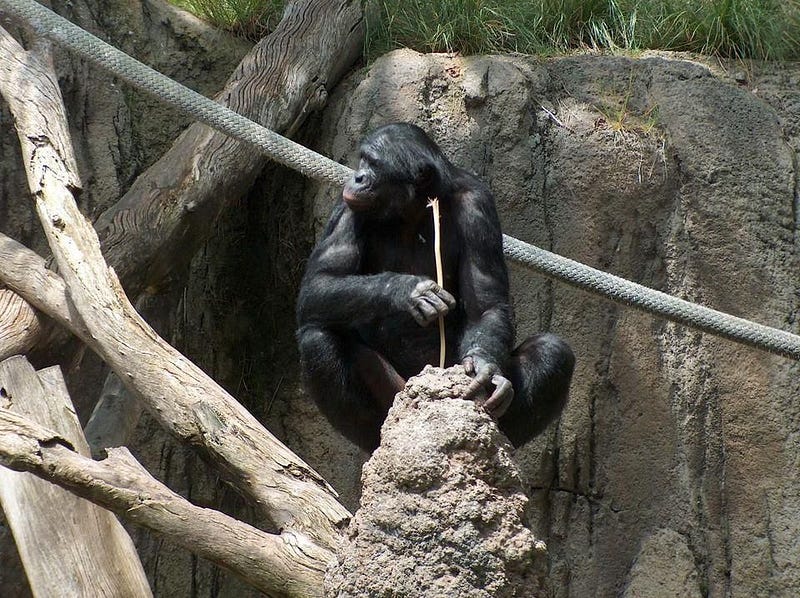
Again, Earth is our only laboratory for this, but let’s be optimistic in the absence of evidence, and assume there’s a 1-in-1,000 chance that a world that starts with a primitive, replicating, information-encoding strand of life can lead to something like the Cambrian explosion. That gives us 10,000 worlds in the Milky Way teeming with diverse, multicellular, highly differentiated forms of life. Given the distance between the stars, that means there’s likely another planet where this has occurred just a few hundred light years away.
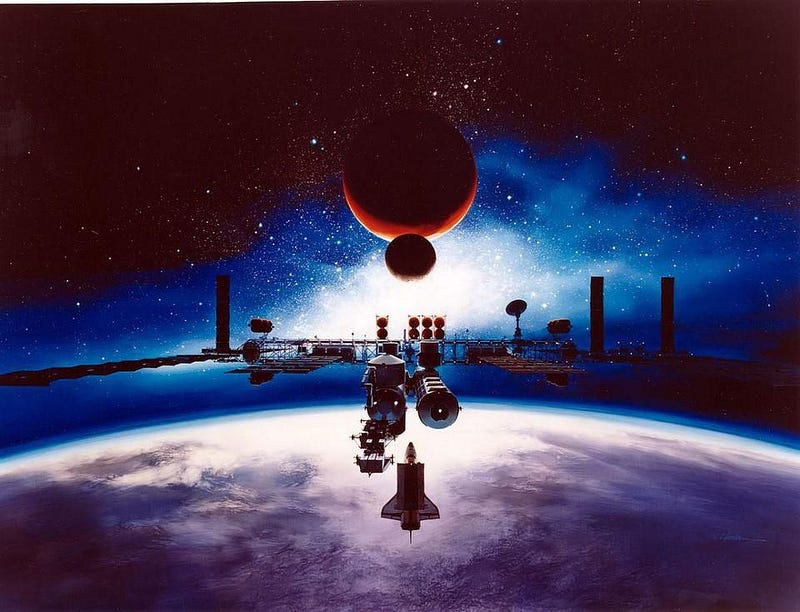
7.) f_t: the fraction of those worlds which presently house a scientifically/technologically advanced civilization. This is a superior question to the ones asked by the Drake equation. Who cares if this is the first or the tenth time a technologically advanced civilization arose? Who cares if they’re using radio waves? Who cares if they blow themselves up or self-extinct, or whether they have spacefaring ambitions or not? The big question is whether there are extraterrestrials who are intelligent the way we’re intelligent, and that means scientifically and technologically advanced.
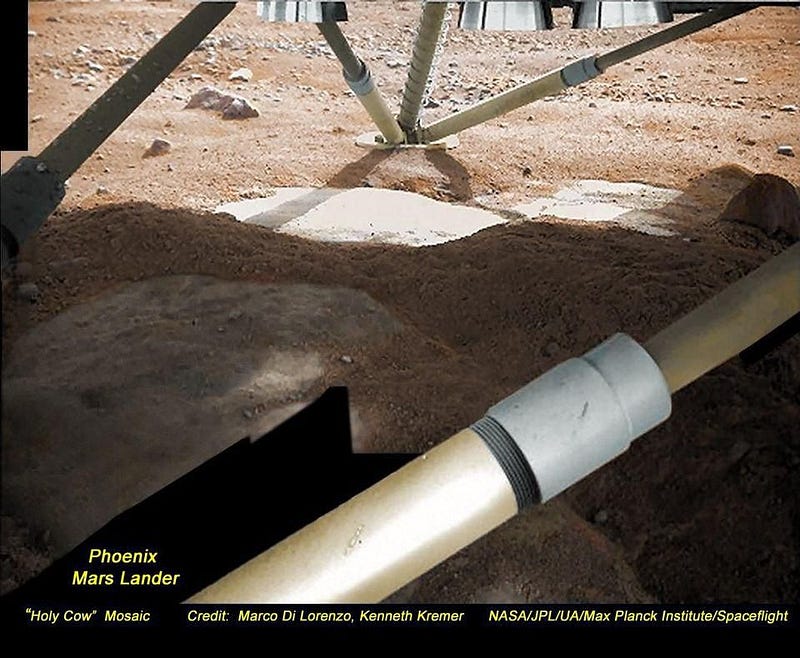
There’s no evidence for this anywhere other than Earth, of course, which means there’s a huge range of possibilities. It could be easy, like 1% of them get there, or it could be a freak coincidence that humanity arose at all, and the odds could be more like one-in-a-billion. Here on Earth, it’s been about 500,000,000 years since the Cambrian explosion, and we’ve only had a technologically advanced species on the planet for less than 1,000 years. Assuming humanity lasts for a few thousand more in this state, that means that Earth will have spent 1-in-100,000 of our time with complex, differentiated organisms in a technologically advanced state.
Even with 10,000 such worlds in the Milky Way, there’s only approximately a 10% chance, under these estimates, that another scientifically/technologically advanced civilization exists at the same time as us.
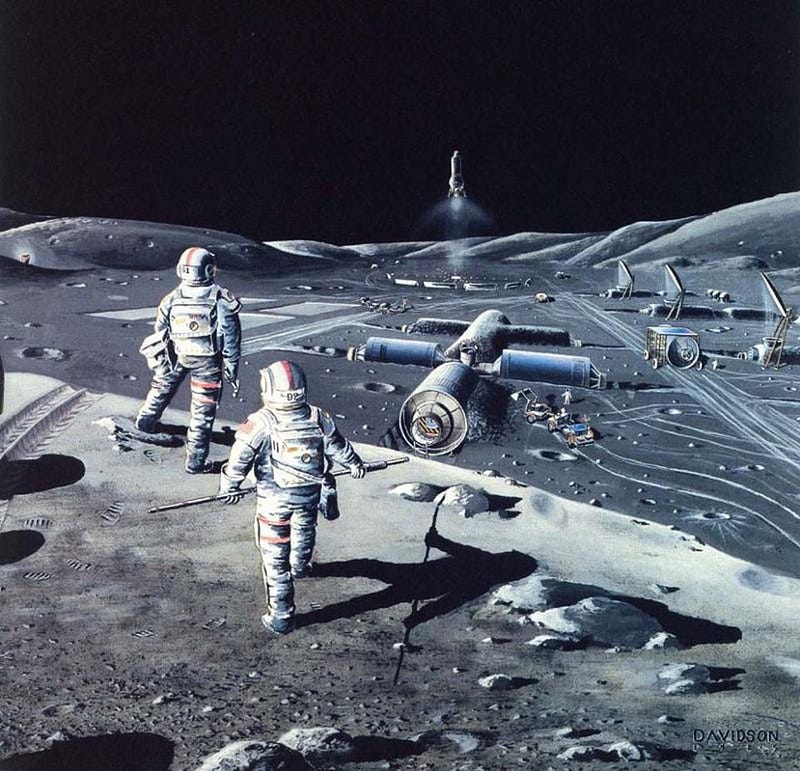
But with all that said, it’s those last three numbers — f_l, f_x, and f_t — that have such large uncertainties that make accurate estimates an impossibility right now.
Knowing how many worlds there are out there in the Milky Way with life on them, and finding even one, would have tremendous implications for our existence, and for understanding our place in the Universe. Taking even the next step, and learning that there were complex, differentiated, large organisms on a world, like we have with the fungal, animal, and plant kingdoms on Earth, would revolutionize what’s possible. And finally, the chance we’d have to have communication, visitation, and a knowledge exchange with a scientifically or technologically advanced alien species would forever alter the course of humanity. It’s all possible, but there’s so much more we need to know if we ever want to find out. We must take these steps; the rewards are too great if there’s even a chance of learning these answers.
Ethan Siegel is the author of Beyond the Galaxy and Treknology. You can pre-order his third book, currently in development: the Encyclopaedia Cosmologica.