How Stephen Hawking’s Greatest Discovery Revolutionized Black Holes
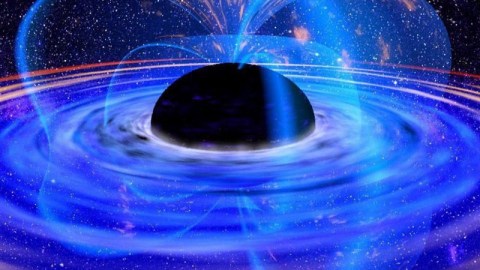
Before Hawking, black holes were just static points in the background of space. His greatest scientific legacy taught us just how dynamic they are.
In 1915, Albert Einstein published his General theory of Relativity, replacing our old Newtonian worldview with a unified concept of spacetime. On one side of Einstein’s equations, the matter and energy in the Universe told spacetime how to curve; on the other side, the curved fabric of spacetime told matter and energy how to move. The complicated nature of these equations ensured that exact solutions would be hard to find, as Einstein himself only ever found two: one for completely empty space and one for a single mass in the weak-field limit. The next year, Karl Schwarzschild found the first interesting solution, for a point mass over all of space. We now recognize this as the solution for a black hole, one of the few exact solutions known even today. While in Schwarzschild’s formulation, black holes were static objects, Hawking was the first to prove that it isn’t so. Black holes radiate over time, and as such, aren’t even completely black.
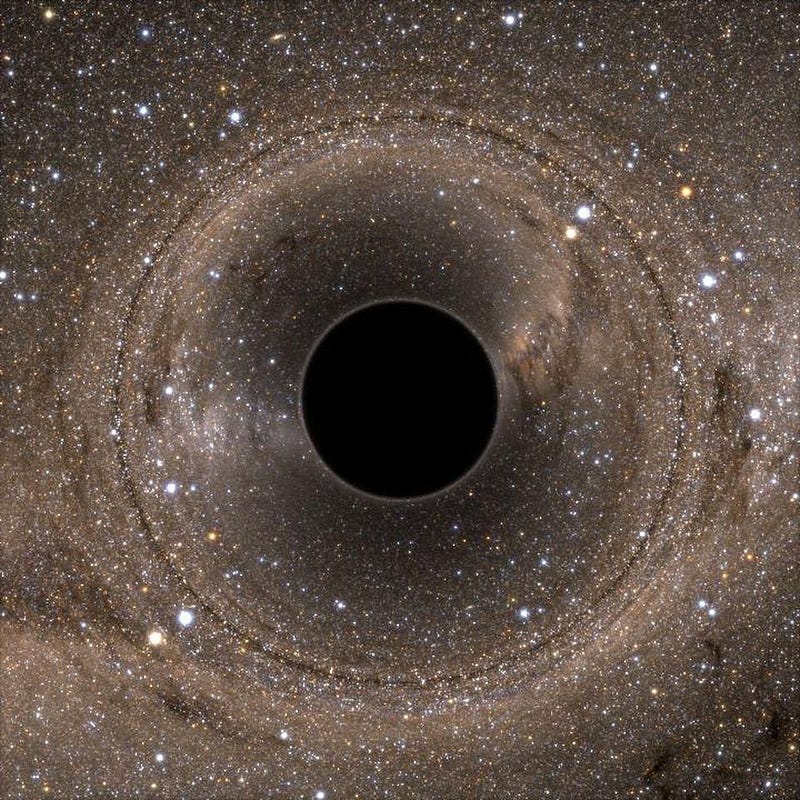
It’s been known for a long time that there are only a few properties that can describe a black hole. In Schwarzschild’s case, he simply assigned it mass, and solved for the curvature of spacetime. It was shown by others that you could add a charge (Reissner–Nordström black holes) or a spin (Kerr black holes), but that was it. What you couldn’t do was add information into a black hole: an electrically neutral, non-rotating human being contained as much information as an equivalent cloud of hydrogen gas once it entered a black hole. From a thermodynamic point-of-view, this was a disaster. You could throw a cloud of hydrogen gas with a temperature of absolute zero, and hence an entropy of zero, into the black hole, and it would have the same effect on the black hole as throwing an equivalent-energy human being in there. This simply didn’t make sense.
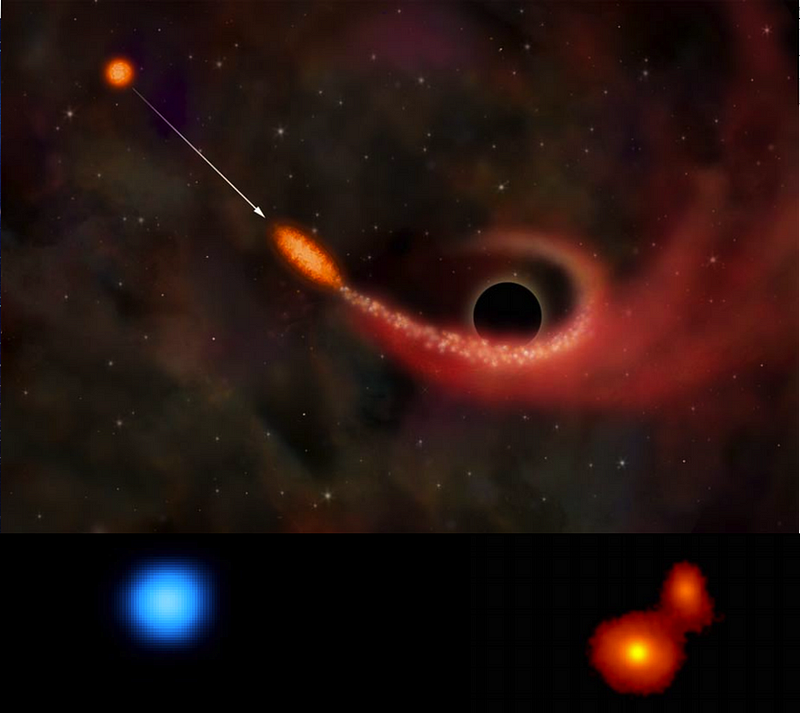
It meant that, contrary to the second law of thermodynamics, it meant we suddenly had a way to arbitrarily decrease the entropy of the Universe. A black hole, classically, should have an entropy of zero. If you could throw objects with real, positive, and large amounts of entropy into a black hole, you’d have a way to violate that law. Entropy always increases, as far as we know, and this was one of the things Hawking was thinking about when he was considering what was puzzling about black holes. There must be some way to define it for black holes, and that value ought to be both positive and large. Increasing entropy, over time, should be okay, but decreasing it should be forbidden. The only way to ensure that would be by forcing an increase in the black hole’s mass to cause entropy to go up by at least the largest amount you can imagine.
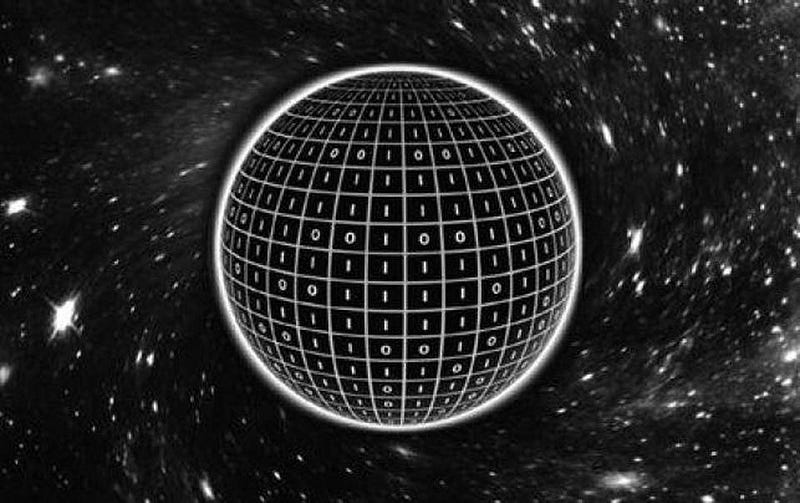
The way that people working on that problem — including Hawking — assigned an answer was to make entropy proportional to the surface area of a black hole. The more quantum bits of information you can fit on a black hole, the greater its entropy was. But that brought up a new problem: if you have entropy, then that means you have a temperature. And if you have a temperature, you have to radiate energy away. Originally called “black” because nothing, not even light, can escape, now it became clear it had to emit something after all. All of a sudden, a black hole isn’t a static system anymore; it’s one that changes over time.
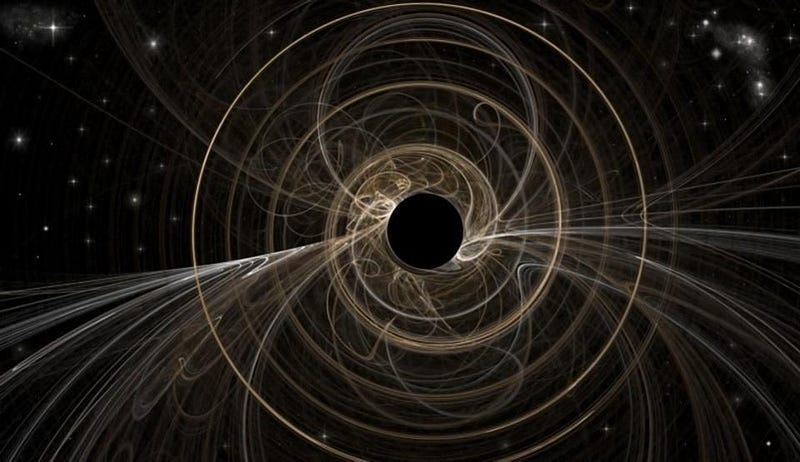
So if a black hole isn’t so black, and if it’s radiating, the big question now becomes how. How does a black hole radiate? Figuring out the answer to this conundrum was Hawking’s biggest contribution to physics. We know how to calculate, in quantum field theory, how the vacuum of empty space behaves when space is flat. That is, we can tell you properties of empty space when you’re very far away from any masses, like a black hole. What Hawking showed, for the first time, is how to do this in curved space: within a few radii of the event horizon. And what he found was that there was a marked difference in the behavior of the quantum vacuum when a mass was near.
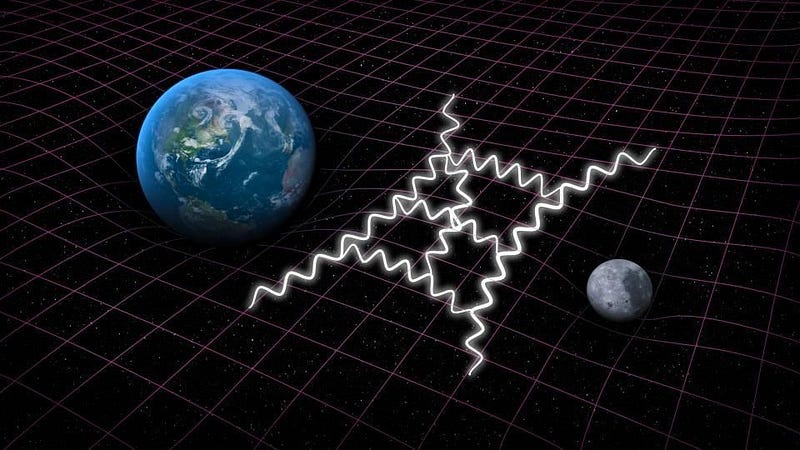
When he ran through the math, he found the following properties:
- When you’re far from the black hole, it looks like you get the thermal emission of blackbody radiation.
- The temperature of the emission is dependent on the black hole’s mass: the lower the mass, the higher the temperature.
- As the black hole emits radiation, it decreases in mass, in exact accord with Einstein’s E = mc². The higher the rate of radiation, the faster the mass loss.
- And as the black hole loses mass, it shrinks and radiates faster. The time a black hole can live is proportional to its mass cubed: the black hole at the Milky Way’s center will live some 10²⁰ times longer than a black hole of the Sun’s mass.
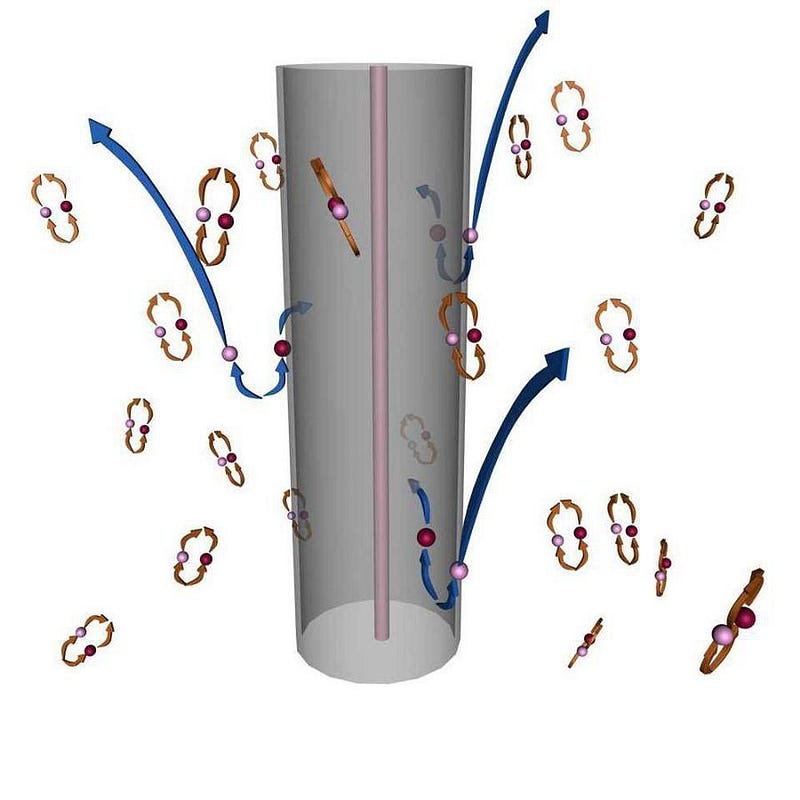
Originally, Hawking visualized this as particle/antiparticle pairs popping in-and-out of existence, annihilating away to produce radiation. That oversimplified picture was qualitatively good enough to describe the radiation far from the black hole, but it turns out to be incorrect close to the event horizon. It’s more accurate to think of the vacuum changing, and of the radiation as being emitted from wherever the curvature of space is relatively large: within a few radii of the black hole itself. Once you get far away, though, everything just appears to be this thermal, blackbody radiation.
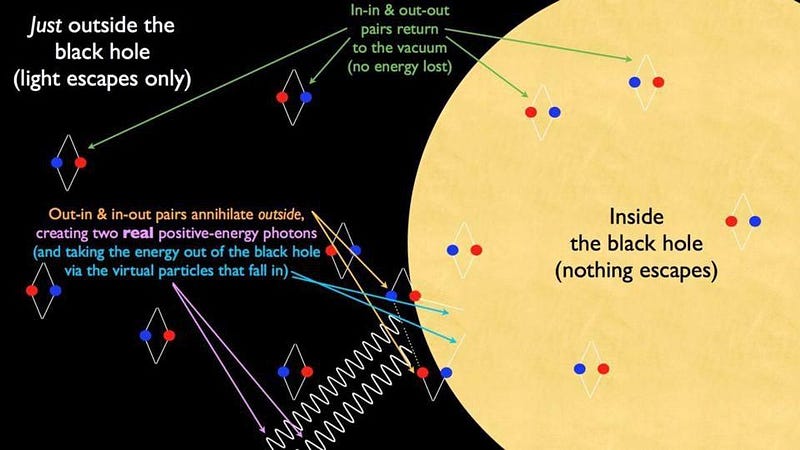
All at once, there was a revolution in black holes, and in understanding how quantum fields behave in highly curved space. It opened up the black hole information paradox, as we’re now asking where the information encoded on the black hole’s event horizon goes when a black hole evaporates? It opens up the (related) problem of black hole firewalls, asking why don’t objects get fried by radiation as they cross the event horizon, or whether they in fact do? It tells us there’s a relationship between what happens within a volume (in the space enclosed by the event horizon) and the surface encapsulating it (the event horizon itself), which is a potential example of the holographic principle in real life. And it opens the door to additional subtleties that may allow us, for the first time, to probe the effects of quantum gravity if there are any departures from the predictions of General Relativity.
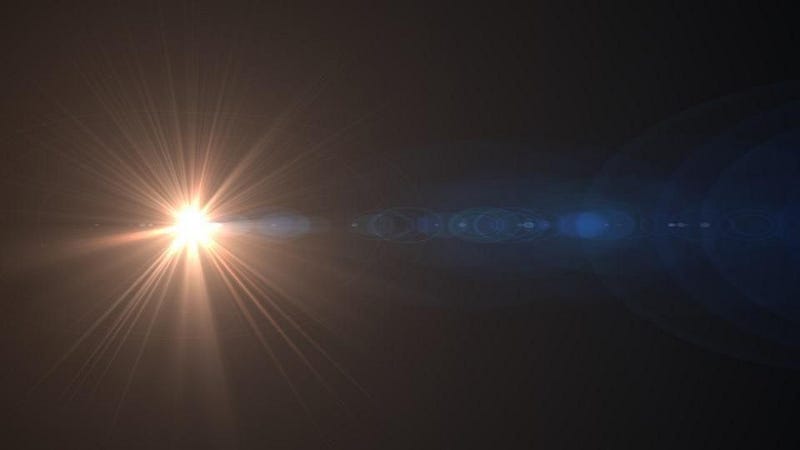
The paper that led to all this was simply titled Black Hole Explosions? and was published in Nature back in 1974. It would have been the crowning achievement of a lifetime of research, and Hawking published it when he was merely 32 years old. He had been researching singularities, black holes, baby universes, and the Big Bang for many years, having collaborated with titans like Gary Gibbons, George Ellis, Dennis Sciama, Jim Bardeen, Roger Penrose, Bernard Carr, and Brandon Carter, to name a few. His brilliant work didn’t come out of nowhere, but arose out of a combination of a brilliant mind thriving in a fertile academic environment. It’s a lesson to us all in how important it is, if we want to have these titanic theoretical advances, to create (and fund) these quality environments where research like this can come to life.
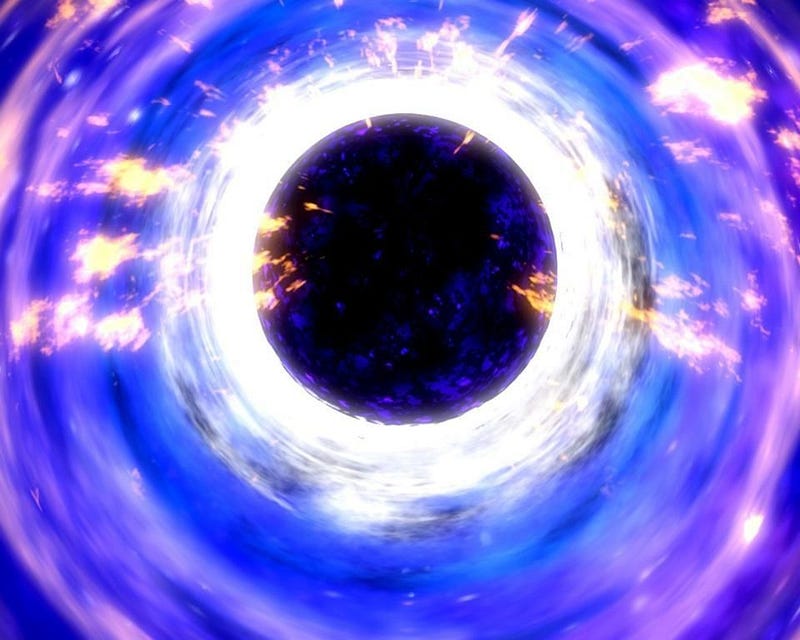
Nearly half a century later, the world mourns his passing, but the legacy of his research lives on. Perhaps this will be the century where there paradoxes are resolved, and the next titanic leaps forward in physics are taken. Regardless of what the future holds, Hawking’s legacy is secure, and the most any theorist can hope for is that their theories will be improved in time. As Hawking himself stated:
Any physical theory is always provisional, in the sense that it is only a hypothesis: you can never prove it. No matter how many times the results of experiments agree with some theory, you can never be sure that the next time the result will not contradict the theory.
While the world may have lost one of its great scientific luminaries with Hawking’s demise, his impact on our knowledge, understanding, and curiosity will echo throughout the ages.
Ethan Siegel is the author of Beyond the Galaxy and Treknology. You can pre-order his third book, currently in development: the Encyclopaedia Cosmologica.