LIGO Misses 100,000 Black Hole Mergers A Year
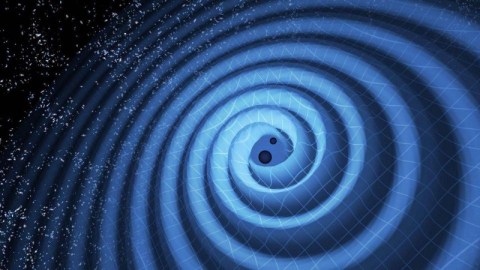
But if a radical new idea comes to fruition, maybe we can find them after all.
After decades of planning, building, prototyping, upgrading, and calibrating, the Laser Interferometer Gravitational-wave Observatory (LIGO) finally achieved it’s ultimate goal just a little over two years ago: the first direct detection of gravitational waves. Since 2015, LIGO has seen the ripples in spacetime or gravitational waves from no fewer than six separate events. Five (and possibly more) black hole-black hole pairs and one neutron star-neutron star inspiral-and-merger had their unique, unmistakable signatures detected by multiple gravitational wave detectors simultaneously, enabling us to confirm a key prediction of Einstein’s General Relativity that had eluded experimentalists for a century. But in theory, black hole-black hole mergers should occur every few minutes somewhere in the Universe; LIGO is missing more than 100,000 of these annually. For the first time, a team of scientists may just have figured out how to detect all the mergers that LIGO is currently missing.
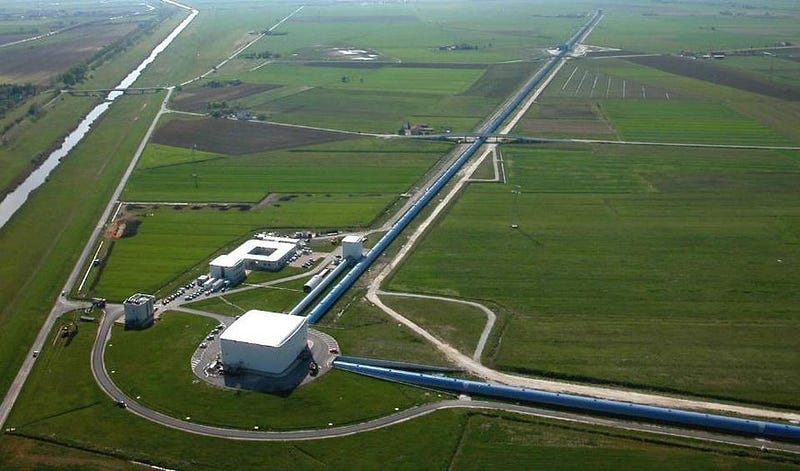
When two black holes orbit one another, they’re both radiating energy away, and doing so constantly. According to Einstein’s General Relativity, any time a mass moves and accelerates through a changing gravitational field, itself changing its momentum, it has to emit radiation inherent to space itself: gravitational radiation. Each of the two masses in their gravitational dance emits them, and part of the theoretical work behind LIGO was calculating in excruciating detail what the magnitude, duration, amplitude, and frequencies of gravitational waves would be emitted for any two arbitrary black hole masses and orientations.
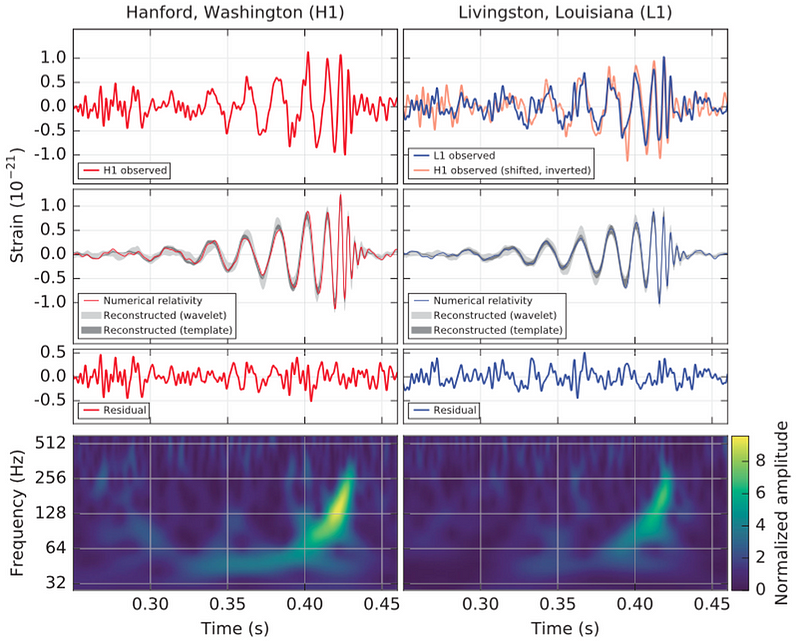
It was only from that sort of template creation and matching that we were able to detect these events at all. It was incredibly successful as well; the confirmations, when they occurred, were spectacular in their agreement with the predictions. But LIGO is only sensitive to those final few moments of a merger, where the amplitude of these gravitational waves is sufficient to contract-and-expand these enormous arms by a tiny fraction of a wavelength of light, enough so that after a thousand reflections, the light shifts by a barely-perceptible amount.
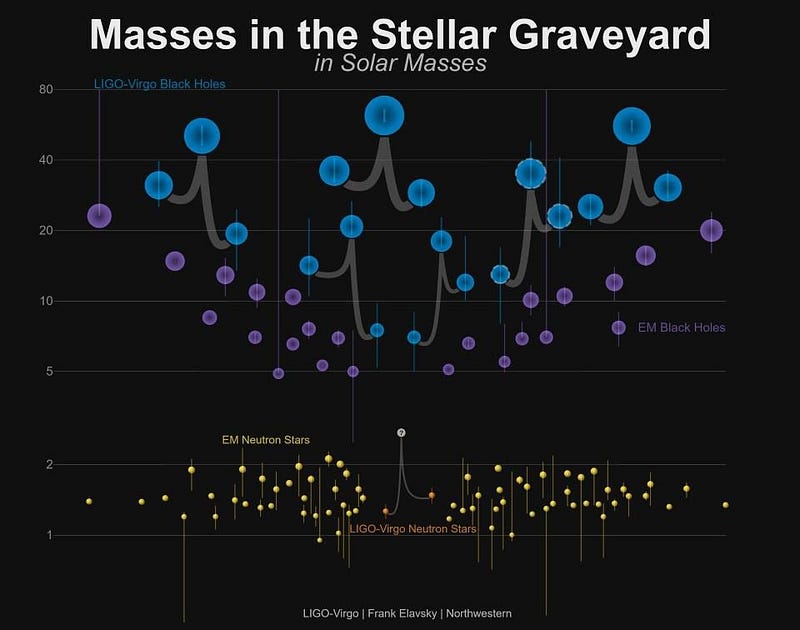
Over the time that LIGO’s been operational, it has seen six robust events: about 0.001% of the total number of mergers expected in the Universe. Sure, most of them are anticipated to be far away, oriented non-optimally, or to occur between low-mass, low-amplitude black holes. There’s a good reason LIGO hasn’t seen them; the current generation of ground-based gravitational wave detectors are severely limited in sensitivity and range.
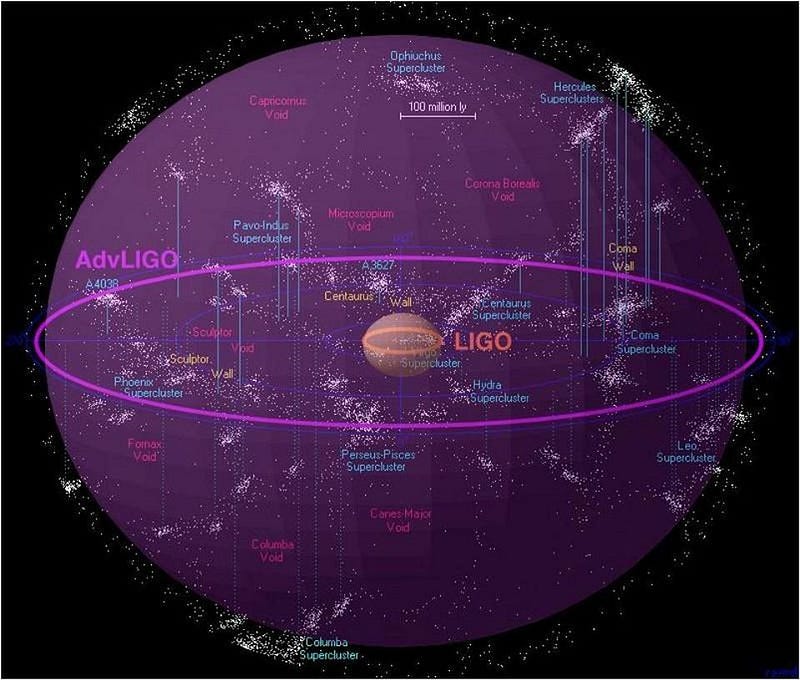
But with 100,000 black hole-black hole mergers occurring annually in the observable Universe, these gravitational wave signals are constantly passing through Earth and our detectors. They’re simply below the detectable threshold, meaning that they have an impact on an apparatus like LIGO or Virgo, but not one we can pull out and identify as a unique, unambiguous gravitational wave event. You may not be able to detect them individually, but with so many of them occurring, it may be possible to extract an aggregate signal. Rather than an individual chirp, these combined mergers should produce a gravitational wave background hum. These mergers are quick and shouldn’t overlap with one another, meaning that the background should look like a series of disconnected signals that are too faint to detect.
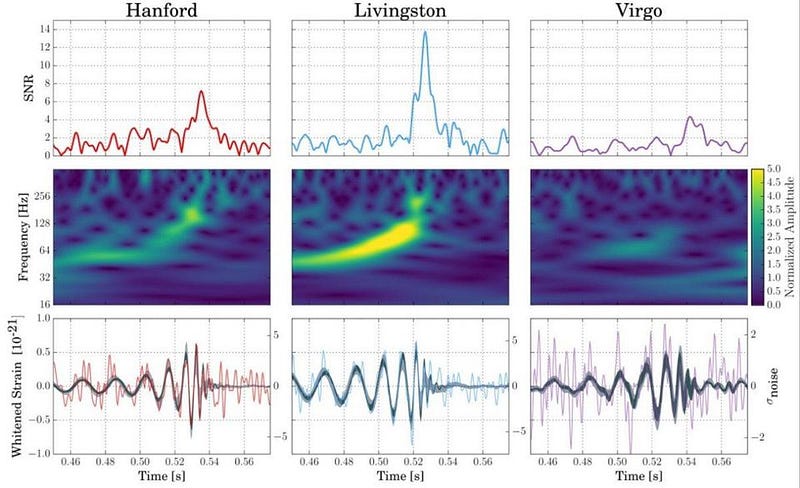
That is, they’re too faint to detect individually! But if you know what your signal looks like and you both build up enough statistics and apply enough computational power, you just might be able to tease it out of the noise. It won’t tell you how many individual events you have, but it can tell you how many total events there are over the time you observe it. In other words, rather than say, “we expect 100,000 of these a year,” we can actually observe the overall black hole-black hole merger rate in the Universe. More importantly, we can learn, for the first time, what the total number-and-mass density of black holes in the Universe actually is.
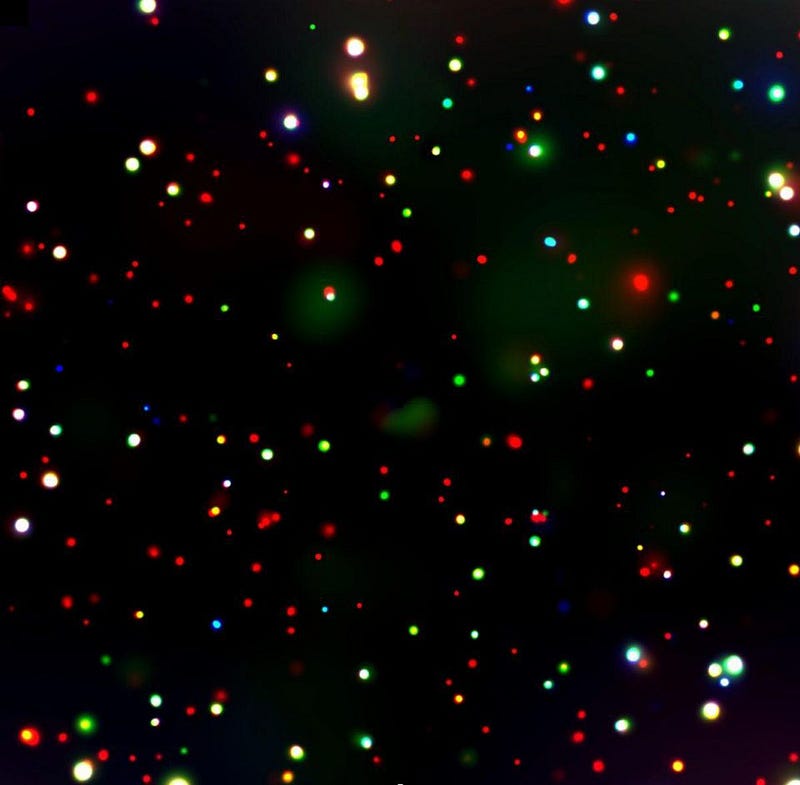
In a new paper entitled Optimal Search for an Astrophysical Gravitational-Wave Background, scientists Rory Smith and Eric Thrane propose to do exactly that. For every problem like this, there’s a computationally optimal way to approach it, and Smith and Thrane worked hard to come up with the answer. There are a number of interesting things the authors deduce they can learn from this computational exercise:
- You can derive the most sensitive possible search for this background of unresolved black holes.
- You can learn about the populations of black holes at earlier times in the Universe compared to the modern, nearby Universe.
- You can combine the results of this search with both confirmed detections and marginal, candidate detections to remove the bias inherent in seeing the largest-amplitude signals the most easily.
- If it’s successful, this method can be generalized to neutron stars, non-merging masses, and even potentially the gravitational wave background left over from the Universe’s birth.
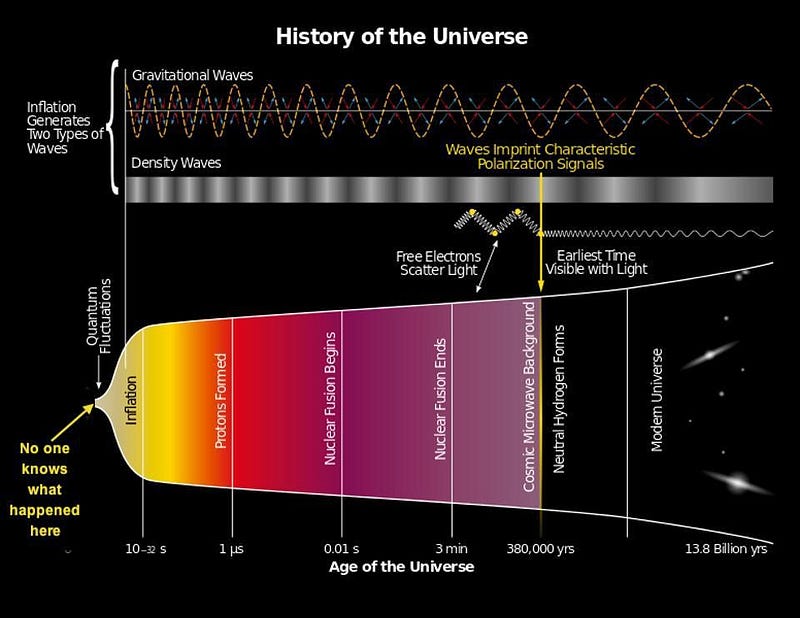
Best of all, their conclusions are incredibly optimistic for what the future holds for applying this supercomputer-based technique to the LIGO and Virgo data sets. Writing in the journal Physical Review X, they state:
Preliminary estimates suggest that advanced detectors, operating at design sensitivity, can detect a stochastic background from binary black holes in about 1 day. These estimates rely on extrapolation using Gaussian mixture modeling of our Bayesian evidence distributions. The next step is to carry out a mock data challenge in which we demonstrate the safety and efficacy of the search using ≈1 day of design sensitivity Monte Carlo data. Such a demonstration would allow us to verify the extrapolations made here with a modest computational cost ≈500 000 core hours.
In other words, they plan to demonstrate that this signal can be extracted from a noisy background by simulating it, blinding the computer, and then proving that the supercomputer, alone, can identify it.
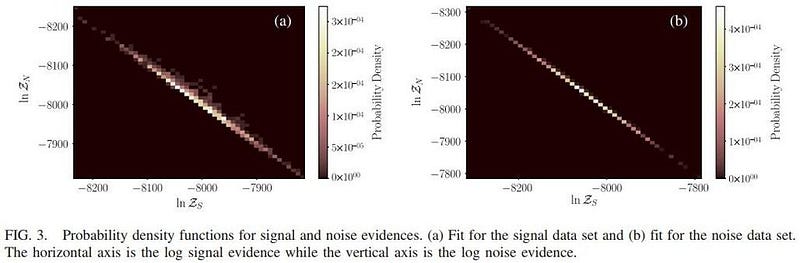
The era of gravitational wave astronomy is now upon us. Owing to the incredible capabilities of ground-based detectors like LIGO and Virgo, we have now detected six robust events over the past 2+ years, from black holes to merging neutron stars. But huge questions surrounding the black holes in the Universe, such as how many there are, what their masses are early on compared to today, and what percent of the Universe is made of black holes, still remain to be answered. The direct efforts have gotten us a very long way, but the indirect signals matter, too, and can potentially teach us even more if we’re willing to make inferences that follow the physics and math. LIGO may be missing upwards of 100,000 black hole-black hole mergers a year. But with this new proposed technique, we might finally learn what else is out there, with the potential to apply this to neutron stars, non-merging black holes, and even the leftover ripples from our cosmic birth. It’s an incredible time to be alive.
Ethan Siegel is the author of Beyond the Galaxy and Treknology. You can pre-order his third book, currently in development: the Encyclopaedia Cosmologica.