Ask Ethan: Why Did Light Arrive 1.7 Seconds After Gravitational Waves In The Neutron Star Merger?
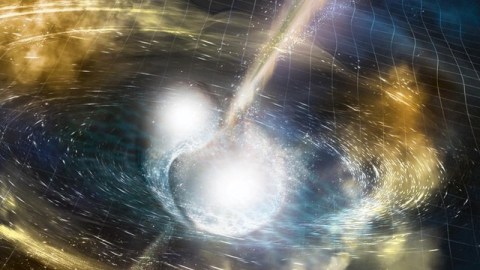
With a journey of 130 million light years, both signals should move at the speed of light. So why did one get here first?
On August 17, after a journey taking 130 million years, the gravitational wave signal of two neutron stars, spiraling inwards in the final stages of a merger, finally arrived at Earth. As the surfaces of the two stars collided, the signal abruptly ended, and then there was nothing. Although these stellar corpses, perhaps only 20 kilometers in diameter, were moving at some 30% the speed of light, we saw nothing in the immediate aftermath. Only 1.7 seconds later did the first signal arrive: light in the form of gamma rays. Why did this delay happen? It’s an incredible question, and what Joel Mills wants to know:
Please discuss significance of the 1.7 sec. difference in arrival time between GW and Gamma Ray burst for the recent Neutron star event.
Let’s take a look at what we saw, and try to figure out why this delay existed at all.
As the neutron stars inspiraled and merged, the gravitational wave signal got stronger and stronger. Unlike with merging black holes, there is no event horizon and no singularity at the core. For neutron stars, there’s a “hard” surface made mostly of neutrons (90%) with other atomic nuclei (and a few electrons) at the edge. When those two surfaces collide with one another, it’s anticipated that a severe, runaway nuclear reaction occurs, resulting in:
- the expulsion of a significant amount of matter, many times the mass of Jupiter,
- the formation of a central collapsed object, likely a black hole after no more than a few hundred milliseconds for these particular masses,
- and then the acceleration and ejection of material surrounding the merging objects.
We now know, thanks to observations collected from over 70 telescopes and satellites, from gamma rays all the way down to radio wavelengths, that this is where the majority of the heaviest elements in the periodic table come from. We know that a rapidly rotating neutron star likely formed at the core for a fraction of a second, then collapsed into a black hole. And we know that the first electromagnetic signal from this merger — the high-energy gamma rays — arrived just 1.7 seconds after the gravitational wave signals came to an end. Over a timescale of those 130 million years that the light travels, this means the gravitational wave and electromagnetic signals traveled at the same speed to better than one part in a quadrillion: 1-in-1015.
But why did the gamma rays get here afterwards? Why didn’t they simply arrive at the same time as the gravitational waves? There are two possible likely scenarios:
- The gamma rays weren’t emitted until 1.7 seconds after the first contact of the neutron star surfaces,
- Or the gamma rays were emitted almost immediately, and were delayed as they passed through the surrounding matter.
These two possibilities both contain the caveat that the true answer could be a combination of both factors or an unlikely alternative involving exotic physics (like a slightly different speed for gravitational waves and electromagnetic waves). Let’s examine how both scenarios could play out.
Delayed emission of gamma rays: when two neutron stars collide, we know they generate gamma rays. It’s long been the leading theory — for over 20 years — that the origin of short gamma rays bursts is with colliding neutron stars, a picture that’s been spectacularly confirmed by the GW170817 event. But are gamma rays generated:
- on the surface of the neutron stars,
- from the collision of ejected material with the surrounding matter,
- or within the cores of neutron stars?
If it’s either of the latter two options, these gamma rays ought to be delayed. It takes time for neutron stars to merge, to eject material, to have that material collide with the surrounding matter, and then for that highly-energetic material to emit gamma rays. If the material is a significant distance away from the neutron star, like tens or hundreds of thousands of kilometers away, that would very simply explain the delay.
Alternately, if the gamma rays are generated not on the surface, but in the interiors of the colliding neutron stars, we’d expect there would be a delay as the light took time to propagate to the neutron star’s surface, where it could then be released. Gravitational waves aren’t delayed by having to travel through dense matter, but light is. This would be extremely analogous to what we observed during the supernova we saw in 1987, where the neutrinos (which aren’t delayed by passing through matter) arrived four hours before the first light signals did, owing to the fact that the light was slowed by its need to pass through a large amount of matter. Either one of these explanations could cause a delay in the emission of gamma rays.
Immediate emission, but delayed arrival of gamma rays: this is the other major scenario. Even if gamma rays are emitted immediately, they still need to pass through the matter-rich environment surrounding the neutron star. It’s got to be matter-rich, because with neutron stars moving that quickly (close to the speed of light) through space, and with the intense magnetic fields they produce, material is bound to be ejected and stripped off as they inspiral and merge. This dance has been going on for a long time, and so there’s definitely large amounts of matter the light needs to travel through before it can arrive at our eyes. Is there enough matter there to cause that 1.7 second delay? There could be, and that’s the other major option.
The way we’ll arrive at the answer involves a combination of examining more events in a variety of mass ranges: below a combined mass of 2.5 solar masses (where you should get a stable neutron star), between 2.5 and 3 solar masses (like the event we saw, where you get a temporary neutron star that becomes a black hole), and above 3 solar masses (where you go directly to a black hole), and measuring the light signals. We’ll also learn more by catching the inspiral phase faster, and being able to point towards the anticipated source in advance of the merger. As LIGO/Virgo and other gravitational wave detectors both come online and get more sensitive, we’ll get better and better at this.
Exotic ideas, like a different speed for gravity and light, are completely unnecessary to explain this observation. Multiple different lines of thought involving conventional physics could be successful at describing why a small, 1.7 second delay occurred. While gravitational waves simply pass through matter unperturbed, light interacts with it electromagnetically, and that could make all the difference in the world. Unlike supernovae, however, the objects (neutron stars) that give rise to gamma ray bursts are tiny, and so wherever the solution lies, it will likely involve understanding a cataclysmic event on extremely short timescales. While theorists race to catch up, the data is already here. The next event could make all the difference in the world.
Submit your questions for Ask Ethan to startswithabang at gmail dot com!
Ethan Siegel is the author of Beyond the Galaxy and Treknology. You can pre-order his third book, currently in development: the Encyclopaedia Cosmologica.