Ask Ethan: Is Dark Matter The ‘Aliens’ Of Astrophysics?
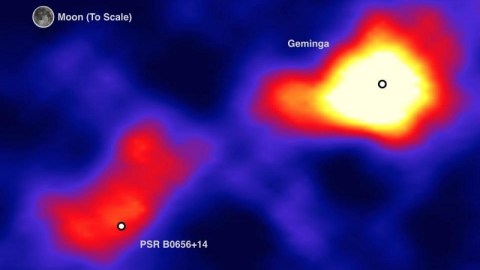
If you can’t explain the astrophysical signal that you see and cry ‘dark matter,’ chances are you’re not thinking hard enough.
Despite our knowledge of the laws of physics, and the successes of the Standard Model and General Relativity, there are a number of observations out there in the Universe that still lack a complete explanation. From star formation to high-energy cosmic rays, the Universe still has its mysteries. Although we’ve discovered a great deal about space, we still don’t know it all. For example, we know that dark matter exists, but we don’t know what it’s properties are. Does that mean we can attribute anyunknown effect to dark matter? An anonymous reader wants to know.
There are SO many things I want to know… dark matter. The standard statement: [it] doesn’t interact with matter except gravitationally. So, much like the old conundrum about Black holes — something that sucks up everything — how DO you find it? Then I read it is detectable (at least somewhat) by means other than gravitational lensing. What is the annihilation process? Similar to positron/electron [annihilation]?
There are a lot of mysteries out there, as well as a lot of evidence for dark matter. But blaming dark matter for the other mysteries is not only short-sighted, it’s a terrific example of showing what happens when scientists run out of good ideas.
Dark matter is everywhere throughout the Universe. First posited in the 1930s to explain the rapid motions of individual galaxies within galaxy clusters, it came about because it was realized that all the normal matter out there — the stuff made of protons, neutrons, and electrons — was insufficient to explain the total amount of gravity. This includes stars, planets, gas, dust, interstellar and intergalactic plasma, black holes, and everything else we can measure. The lines of evidence supporting dark matter are numerous and overwhelming.
They don’t merely include galaxies within clusters, although certainly every cluster containing galaxies exhibits this need. Dark matter is necessary for:
- the rotational properties of individual galaxies,
- the formation of galaxies of many different sizes, from giant ellipticals to Milky Way-sized galaxies to the tiny, dwarf galaxies around us,
- the interactions between pairs of galaxies,
- the clustering properties of galaxies and galaxy clusters on large scales,
- the cosmic web, including its filamentary structure,
- the spectrum of fluctuations in the cosmic microwave background,
- the observed gravitational lensing effects of distant masses, and
- the observed separation between the effects of gravity and the presence of normal matter in colliding galaxy clusters.
From the small scales of individual galaxies up to that of the entire Universe, dark matter is necessary.
Putting all of this in context with the rest of cosmology leads us to believe that every galaxy, including our own, contains a massive, diffuse halo of dark matter surrounding it. Unlike the stars, gas, and dust in our galaxy, which exists primarily in a disk, the dark matter halo is expected to be spherical, as the overwhelming evidence is that unlike normal (atom-based) matter, dark matter doesn’t go “splat” when you smash it into either itself or normal matter. Furthermore, the dark matter should be densest around the galactic center, decreasing in density as it goes out, and extending perhaps ten times as far as the stars in the galaxy itself. Finally, there should be small clumps of dark matter that exist throughout each and every halo.
In order to reproduce the full suite of observations listed above, as well as others, dark matter doesn’t need to have any properties other than the following: it needs to have mass; it needs to interact gravitationally; it needs to be moving slowly relative to the speed of light from very early times; and it needs to not interact too much through any of the other forces. That’s it. Any other interactions are severely constrained, but not ruled out.
So why is it, every time there’s an astrophysical observation where there’s an excess of some type of normal particle — photons, positrons, antiprotons, etc. — people’s first instinct is to blame dark matter?
Earlier this week, a team looking at gamma-ray sources around pulsars published their results in Science, attempting to better understand where our observed positron excess comes from. Positrons, the antimatter counterpart of electrons, are naturally produced in a variety of ways: by accelerating normal matter particles to high enough energies that, when they collide with other matter particles, they can produce electron-positron pairs via Einstein’s E = mc2. We create these pairs routinely in particle physics experiments, and we can also see the evidence of positron creation astrophysically, both directly in cosmic ray searches and indirectly, by looking for the telltale energy sign of electron-positron reannihilation.
These astrophysical positron signatures are seen around the galactic center, focused on point sources like microquasars and pulsars, located in a mysterious region of our galaxy known as the great annihilator, and seen as part of a diffuse background whose origin is unknown. One thing is for certain, though: we see more positrons than we expect, overall. We’ve known this for years; PAMELA measured it, Fermi measured it, and the Alpha Magnetic Spectrometer aboard the ISS measured it. Most recently, the High-Altitude Water Cherenkov Observatory (HAWC) measured very high energy, TeV-level gamma rays, showing there are extremely accelerated particles coming from around middle-aged pulsars. But, unfortunately, it isn’t enough to explain the positron excess we need.
But for some reason, with every measurement of the positron excess, or every observation of an astrophysical source that can’t account for it, the narrative immediately becomes, “we can’t explain it, so therefore it’s due to dark matter.” Which is too bad, because there are many candidate astrophysical sources that don’t require anything exotic, including:
- secondary production of positrons and gamma rays from other particles,
- microquasars or other feeding black holes,
- very young or very old pulsars, including magnetars,
- and supernova remnants.
This list isn’t exhaustive, either, but merely a set of examples for what could be creating this excess.
Many working in the field have favored dark matter, largely because it would be revolutionary and groundbreaking if dark matter did annihilate and produce gamma rays and normal matter particles. It would be the dream scenario for astrophysical dark matter hunters. But wishful thinking never made something true, and as far as we can tell, the dark matter-dark matter annihilation cross-section is still indistinguishable from zero. Although dark matter is always emphasized as a possibility in explaining the positron excess, it’s no more likely than aliens are to explain Tabby’s star.
After reaching out to Brenda Dingus, the principal investigator of HAWC, I received the following comment:
There is no doubt there are other sources of positrons. However, positrons don’t travel far from their sources and there aren’t many sources nearby. The two best candidates were detected by HAWC, and we now know the number of positrons they produce. We also know how those positrons diffuse away from their sources and its slower than previously assumed. Therefore, while we confirmed nearby positron sources, we discovered that the positrons are very slow to move away from their origin and therefore are not making the positron excess at Earth.
When you rule out one possibility, that makes the other possibilities more probable. However, it doesn’t mean that the positrons MUST come from dark matter. We did not mean to imply that.
It’s true and remarkable that the positrons implied by the HAWC data explain only 1% of the positrons observed by the other experiments, indicating that something else is responsible. When you see an observation that our conventional ideas can’t account for, like an excess of astrophysical positrons, keep in the back of your head that it might be dark matter, exhibiting the long-sought-after interaction properties that have eluded us so far. But it’s far more likely that some other astrophysical process is accelerating conventional, known particles to produce these effects. When you have a mystery in science, keep your mind open to a revolution, but place your bets on the mundane. And never, ever believe the hype that claims otherwise.
Starts With A Bang will be taking a one-week hiatus to attend the Frontiers of Fundamental Physics conference in Orihuela, Spain. We’ll return on December 4th, but as always, send in your Ask Ethan questions to startswithabang at gmail dot com!
Ethan Siegel is the author of Beyond the Galaxy and Treknology. You can pre-order his third book, currently in development: the Encyclopaedia Cosmologica.