The Big Bang Wasn’t The Beginning, After All
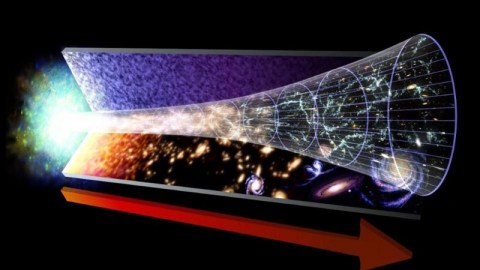
Why you can’t extrapolate back to a singularity.
“Despite its name, the big bang theory is not really a theory of a bang at all. It is really only a theory of the aftermath of a bang.” –Alan Guth
The Universe began not with a whimper, but with a bang! At least, that’s what you’re commonly told: the Universe and everything in it came into existence at the moment of the Big Bang. Space, time, and all the matter and energy within began from a singular point, and then expanded and cooled, giving rise over billions of years to the atoms, stars, galaxies, and clusters of galaxies spread out across the billions of light years that make up our observable Universe. It’s a compelling, beautiful picture that explains so much of what we see, from the present large-scale structure of the Universe’s two trillion galaxies to the leftover glow of radiation permeating all of existence. Unfortunately, it’s also wrong, and scientists have known this for almost 40 years.
The idea of the Big Bang first came about back in the 1920s and 1930s. When we looked out at distant galaxies, we discovered something peculiar: the farther away from us they were, the faster they appeared to be receding from us. According to the predictions of Einstein’s General Relativity, a static Universe would be gravitationally unstable; everything needed to either be moving away from one another or collapsing towards one another if the fabric of space obeyed his laws. The observation of this apparent recession taught us that the Universe was expanding today, and if things are getting farther apart as time goes on, it means they were closer together in the distant past.
An expanding Universe doesn’t just mean that things get farther apart as time goes on, it also means that the light existing in the Universe stretches in wavelength as we travel forward in time. Since wavelength determines energy (shorter is more energetic), that means the Universe cools as we age, and hence things were hotter in the past. Extrapolate this back far enough, and you’ll come to a time where everything was so hot that not even neutral atoms could form. If this picture were correct, we should see a leftover glow of radiation today, in all directions, that had cooled to just a few degrees above absolute zero. The discovery of this Cosmic Microwave Background in 1964 by Arno Penzias and Bob Wilson was a breathtaking confirmation of the Big Bang.
It’s tempting, therefore, to keep extrapolating backwards in time, to when the Universe was even hotter, denser, and more compact. If you continue to go back, you’ll find:
- A time where it was too hot to form atomic nuclei, where the radiation was so hot that any bound protons-and-neutrons would be blasted apart.
- A time where matter and antimatter pairs could spontaneously form, as the Universe is so energetic that pairs of particles/antiparticles can spontaneously be created.
- A time where individual protons and neutrons break down into a quark-gluon plasma, as the temperatures and densities are so high that the Universe becomes denser than the inside of an atomic nucleus.
- And finally, a time where the density and temperature rise to infinite values, as all the matter and energy in the Universe are contained within a single point: a singularity.
This very final point — this singularity that represents where the laws of physics break down — also is understood to represent the origin of space and time. This was the ultimate idea of the Big Bang.
Of course, everything except that last point has been confirmed to be true! We’ve created quark-gluon plasmas in the lab; we’ve created matter-antimatter pairs; we’ve done the calculations for which light elements should form and in what abundances during the early stages of the Universe, made the measurements, and found that they match with the Big Bang’s predictions. Coming forward even farther, we’ve measured the fluctuations in the cosmic microwave background and seen how gravitationally bound structures like stars and galaxies form and grow. Everywhere we look, we find a tremendous agreement between theory and observation. The Big Bang looks like a winner.
Except, that is, in a few regards. Three specific things you would expect from the Big Bang didn’t happen. In particular:
- The Universe doesn’t have different temperatures in different directions, even though an area billions of light-years away in one direction never had time (since the Big Bang) to interact with or exchange information with an area billions of light-years in the opposite direction.
- The Universe doesn’t have a measurable spatial curvature that’s different from zero, even though a Universe that’s perfectly spatially flat requires a perfect balance between the initial expansion and the matter-and-radiation density.
- The Universe doesn’t have any leftover ultra-high-energy relics from the earliest times, even though the temperatures that would create these relics should have existed if the Universe were arbitrarily hot.
Theorists thinking about these problems started thinking of alternatives to a “singularity” to the Big Bang, and rather of what could recreate that hot, dense, expanding, cooling state while avoiding these problems. In December of 1979, Alan Guth hit upon a solution.
Instead of an arbitrarily hot, dense state, the Universe could have begun from a state where there was no matter, no radiation, no antimatter, no neutrinos, and no particles at all. All the energy present in the Universe would rather be bound up in the fabric of space itself: a form of vacuum energy, which causes the Universe to expand at an exponential rate. In this cosmic state, quantum fluctuations would still exist, and so as space expanded, these fluctuations would get stretched across the Universe, creating regions with slightly-more or slightly-less than average energy densities. And finally, when this phase of the Universe — this period of inflation — came to an end, that energy would get converted into matter-and-radiation, creating the hot, dense state synonymous with the Big Bang.
This was regarded as a compelling-but-speculative idea, but there was a way to test it. If we were able to measure the fluctuations in the Big Bang’s leftover glow, and they exhibited a particular pattern consistent with inflation’s predictions, that would be a “smoking gun” for inflation. Furthermore, those fluctuations would have to be very small in magnitude: small enough that the Universe could never have reached the temperatures necessary to create high-energy relics, and much smaller than the temperatures and densities where space and time would appear to emerge from a singularity. In the 1990s, 2000s, and then again in the 2010s, we measured those fluctuations in detail, and found exactly that.
The conclusion was inescapable: the hot Big Bang definitely happened, but doesn’t extend to go all the way back to an arbitrarily hot and dense state. Instead, the very early Universe underwent a period of time where all of the energy that would go into the matter and radiation present today was instead bound up in the fabric of space itself. That period, known as cosmic inflation, came to an end and gave rise to the hot Big Bang, but never created an arbitrarily hot, dense state, nor did it create a singularity. What happened prior to inflation — or whether inflation was eternal to the past — is still an open question, but one thing is for certain: the Big Bang is not the beginning of the Universe!
Ethan Siegel is the author of Beyond the Galaxy and Treknology. You can pre-order his third book, currently in development: the Encyclopaedia Cosmologica.