Why doesn’t dark matter form black holes?
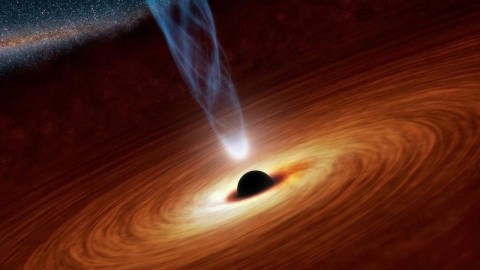
They’re the darkest, most massive things in the Universe. How come they have nothing to do with each other?
“All enterprises that are entered into with indiscreet zeal may be pursued with great vigor at first, but are sure to collapse in the end.” –Tacitus
Dark matter is the most abundant form of mass in our Universe. If you were to add up all the stars, planets, lifeforms, gas, dust, plasma and more — all the known, “normal” matter in our Universe — it would only account for about 15-to-17% of the total gravitation that we see. The remaining mass, outclassing the normal matter by a 5:1 ratio, must be completely invisible, meaning it doesn’t absorb or emit light at all. Yet it must interact gravitationally, enabling it to form large-scale structure in the Universe and to hold galaxies together. So why, then, can’t it form black holes?
Black holes aren’t the only thing dark matter can’t form; it also can’t create dark matter stars, planets or dark atoms. Imagine the Universe as it might have been back in the very, very early stages, before there were any black holes, stars, planets or atoms.
All we had was a hot, dense, expanding “sea” of matter and radiation of all the different types allowed. By time the Universe has aged to be a few minutes old, the atomic nuclei are there, all the electrons are there, all the neutrinos and photons are there, and all the dark matter is there, too.
They’re all flying around at incredible speeds, sure, but they’re also all exerting forces on one another. It’s true that they all feel the gravitational force (even photons, thanks to Einstein’s energy-mass equivalence), but gravity isn’t the only thing that matters here.
Photons and electrons have it the worst: they interact very frequently through the electromagnetic force, scattering and “bouncing” off of one another, exchanging energy, momentum and colliding at an alarming rate. Nuclei fare only a little better: they’re much more massive, so their interaction rate is lower, and they pick up (or lose) less momentum with each collision.
Neutrinos are much luckier: they don’t have an electric charge, and so they don’t interact through the electromagnetic force at all. Instead, they can only interact (besides gravity) through the weak force, which means collisions are incredibly infrequent. But dark matter gets it the best in terms of freedom: as far as we can tell, it only interacts through gravity. There are no collisions at all, and so all dark matter can do is be attracted to the other sources of matter.
This might, you worry, make things worse! While normal matter has collisions and interactions preventing it from collapsing gravitationally, forming denser clumps, etc., the dark matter density begins to grow in the overdense regions. But this doesn’t happen the way you think of “collapse” happening. When a gas cloud collapses to form stars, what happens?
The gas interacts through the gravitational force, becoming denser, but the matter that makes up that gas sticks together, allowing it to reach a denser state. That “stickiness” only happens thanks to the electromagnetic force! This is why things can collapse down to produce bound objects like stars, planets and even atoms.
Without that stickiness? You’d just end up with a diffuse, loosely held together, “fluffy” structure bound together only through gravity. That’s why you hear of dark matter halos on galaxy and cluster scales, of dark matter filaments on even larger scales, and of no other dark matter structures.
Now, these diffuse, fluffy halos are incredibly important: they represent the seeds of all the bound structure in the Universe today. This includes dwarf galaxies, normal galaxies, galaxy groups, galaxy clusters, superclusters and filaments, as well as all the substructure that makes these objects up. But without that extra force — without some “sticky” force to hold it together, to exchange energy and momentum — the dark matter is destined to remain in this fluffy, diffuse state. The normal matter can form the tightly-bound structures you’re used to, but the dark matter has no way to collide inelastically, to lose momentum or angular momentum, and hence, it has to remain loosely bound and “halo-like.”
It’s a little disconcerting to think that it’s not the gravitational force that leads to planets, stars, black holes and more, but gravity is just part of the equation. To really drive this point home, imagine that you took a ball of some type and launched it, with the ball — as you know — made out of atoms. What’s the ball going to do?
Of course, it’ll move in a parabolic path (neglecting air resistance), rising up to a maximum height and falling down until it finally strikes the Earth. On a more fundamental scale, the ball moves in an elliptical orbit with the center-of-mass of the Earth as one focus of the ellipse, but the ground gets in the way of that ellipse, and so the portion we see looks like a parabola. But if you magically turned that ball into a clump of dark matter, what you’d get would surprise you greatly.
Without the electromagnetic force, a whole bunch of terrible things happen:
- There’s no interaction, other than gravity, between the particles making up the ball and the atoms of the Earth. Instead of making a parabola, the dark matter clump goes all the way through the layers of the Earth, swinging around the center in an (almost-perfect) ellipse (but not quite, due to the layers and non-uniform density of the Earth), coming out near where it entered, making a parabola again and continue to orbit like that interminably.
- There are also no interactions holding this clump together! So while atoms in a ball do have some random motions, they are held together by the electromagnetic force, keeping that ball-like structure to it. But if you remove that electromagnetic force, the random motions of the dark matter particles will work to unbind this from being a clump, since the gravitation of the clump itself is insufficient to keep it bound together.
- This means that over time (and many orbits), the dark matter gets stretched into a long ellipse, and that ellipse gets more and more diffuse, similar to the particles that make up the debris stream from a comet, only even morediffuse!
Dark matter can’t form black holes or other tightly-bound structures because gravity alone isn’t enough to bind something tightly together. Because the force of gravity is so weak, it can only bind it loosely, which means huge, diffuse, very massive structures. If you want a “clump” of something — a star, a planet, or even an atom — you need a force that’s stronger than gravity to make it happen.
There may yet be one! It is possible that dark matter self-interacts (or interacts with matter or radiation, at some level), but if it does, we only have constraints on how weak that interaction is. And it is very, very weak, if it’s even non-zero at all.
So even though we think of gravity as the only force that matters on the largest scales, the truth is when we think about the structures that we see — the ones that give off light, that house atoms and molecules, that collapse into black holes — it’s the other forces, in concert with gravity, that allow them to exist at all. You need some type of inelastic, sticky collision, and dark matter doesn’t have the right interactions to make that possible. Because of that, dark matter can’t make a galaxy, a star, a planet or a black hole. It takes more than gravity alone to do the job.
This post first appeared at Forbes, and is brought to you ad-free by our Patreon supporters. Comment on our forum, & buy our first book: Beyond The Galaxy!