Ask Ethan: What’s so ‘spooky’ about quantum entanglement?
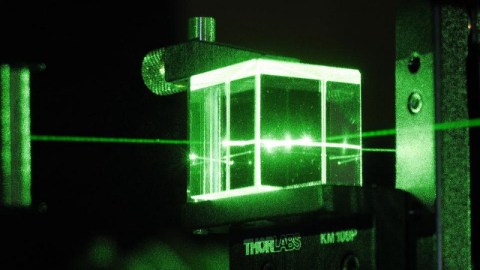
It might have puzzled Einstein right up until his death, but that doesn’t mean you can’t understand it!
“As far as the laws of mathematics refer to reality, they are not certain; and as far as they are certain, they do not refer to reality.” –Albert Einstein
There are a great many puzzles out there in quantum physics, which is notorious for defying our intuition. Particles seem to know whether you’re looking at them or not, displaying different behaviors if you watch them go through a double-slit versus if you don’t. Measuring one quantity, like a particle’s position, creates an inherent uncertainty in a complementary quantity, like momentum. And if you measure its spin in the vertical direction, you destroy information about its spin in the horizontal direction. But the “spookiest” of all quantum phenomena is quantum entanglement, where one particle somehow “knows” if its entangled partner is measured or not instantaneously, even from across the Universe. For this week’s Ask Ethan, we’ve got a question from Dana Doucet, who’s puzzled as to why this is a mystery at all.
[F]rom the photons’ point of view, they have traveled zero distance over zero time. So… what’s so spooky about that? Until one of them is measured, they are in the same place at the same time (if you believe their story) and so it’s not a mystery as to how they coordinate their states.
It’s a well-reasoned line of thinking: that time dilation for a fast-moving particle means that they can coordinate their states as fast as they want. But the mystery isn’t this easy to solve.
Let’s go over the issue of entanglement to start. The experiment is normally done with photons: you pass a single quantum of light through a specialized material (e.g., a down-conversion crystal) which splits it into two photons. These photons will be entangled in a particular sense, where one has a spin, or internal angular momentum, of +1, and the other has a spin of -1. But you don’t know which is which. In fact, there are some experiments you can do where, if you had large numbers of these photons, you’d see a difference between:
- the statistical results if the spin was +1,
- the statistical results if the spin was -1,
- or the statistical results if the spin was undetermined.
It’s very hard to visualize what results we’re talking about, but there’s an outstanding analogy in quantum mechanics: passing a particle through a double slit.
If you fire a particle through a double slit — that is, a screen with two very narrow slits very, very close together — and it passes through rather than being blocked by the screen, you can easily detect where it lands on the other side. If you fire many, many particles, one-at-a-time, through the double slit, you’ll find that the ones that pass through form an interference pattern. In other words, each particle doesn’t act as though it passed through one slit or the other; it acts as though it passed through both slits simultaneously, interfered with itself like a wave, and then continued on.
But this pattern, showcasing the strange quantum mechanical nature of the Universe for all particles, only emerges if you don’t determine which slit the particle goes through.
If you instead make a measurement of the particle as it goes through either slit — which you’re free to do by setting up a gate, a photon, a counter, etc. — you don’t get an interference pattern. You simply get a pile that corresponds to the ones that went through slit 1, and a pile that corresponds to the other that went through slit 2.
In other words, if you make a measurement that determines which path the particle takes, you change the outcome of which path the particle takes! For an individual particle, you’ll only be able to determine a likelihood of it passing through slit 1, slit 2, or having interfered with itself to pass through both. You need a large number of statistics to demonstrate which configuration your setup is truly in.
So now lets come back to the entangled photons. Or, for that matter, anyentangled particles. You create two entangled particles, where you know the sum total of their properties but not their individual ones. Spin is the simplest example — two photons would either be (+1 and -1) or (-1 and +1), two electrons would either be (+½ and -½) or (-½ and +½) — and you don’t know which is which until you measure it. Instead of slits, you can pass it through a polarizer. And the instant you measure one, you determine the other. In other words, you know it instantly.
The “spookiness” comes from the fact that nothing else comes instantly in physics. The fastest any sort of signal can be transmitted is c, the speed of light in a vacuum. Yet you can separate these two entangled particles by meters, kilometers, astronomical units or light years, and measuring one determines the state of the other instantaneously. It doesn’t matter whether the entangled particles move at the speed of light or not, whether they massless or not, whether they’re energetic or not, and whether you shield them from sending out photons to one another or not. There isn’t a loophole where the interaction speed in any reference frame can make up for it. In the late 1990s, experiments set up to separate-and-simultaneously-measure these particles determined that if any information is transmitted between the two particles, it must happen at speeds more than 10,000 times greater than c.
Of course, that can’t happen! In reality, there isn’t any information being transmitted. You can’t make a measurement of a particle in one place and use it to communicate anything to the particle a great distance away. In fact, there were a large number of clever schemes devised to try and use this property of nature to transmit information faster than light, butit was proven in 1993 that no information transfer would ever be possible by this mechanism. There’s actually a simple reason for this:
- If you measure “what is the state of the particle I have,” you learn the state of the other particle, but there isn’t anything that anyone can do with that information until either you reach the other particle or the other particle reaches you, and that communication has to happen at the speed of light or below.
- If you instead force the particle you have to “be in this specific state,” that doesn’t change the state of the entangled particle. Quite to the contrary, it actually breaks the entanglement, so you don’t even learn what the other particle is up to.
It’s a philosophical problem for the realists. It means that if the wavefunction of a particle — or the entangled wavefunction of multiple particles — is actually a real, physical thing that’s out there evolving through the Universe, but requires a huge number of ugly assumptions. You need to assume there are an infinite number of possible realities out there and that we live in only one, even though there’s no evidence for any others. If you’re an instrumentalist* (which is way easier and more practical), you don’t have that philosophical problem; you simply accept that the wavefunction is a calculational tool.
Stephen Weinberg, Nobel Laureate, co-founder of the Standard Model and a brilliant theoretical physicist for a number of reasons, recently decried the instrumentalist approach in Science News, stating that it’s:
“so ugly to imagine that we have no knowledge of anything out there — we can only say what happens when we make a measurement.“
But regardless of your philosophical prevarications, quantum mechanics works, and the wavefunction that entangles particles does enable the entanglement to be broken instantaneously, even across cosmic distances. It’s the only instantaneous thing we know of in the Universe, and that makes it very special indeed!
- — Disclosure: the author of this piece is an instrumentalist, and thinks that the realists allow their view of how the Universe ought to work to color their interpretation of how it’s actually working. Realists disagree.
Submit your questions for Ask Ethan to startswithabang at gmail dot com!
This post first appeared at Forbes, and is brought to you ad-free by our Patreon supporters. Comment on our forum, & buy our first book: Beyond The Galaxy!