LIGO’s executive director explains what it’s like to find a gravitational wave
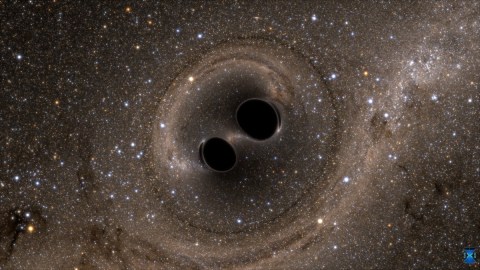
I scored an exclusive interview with Dave Reitze, the executive director of LIGO. Take a trip inside his Universe.
“When I was in high school, I was certain that being an astronaut was my goal. It was a very important time — Sally Ride was making her first flight into space and she had a real impact on me. Those ‘firsts’ kind of stick in your head and really become inspirations for you.” –Karen Nyberg, astronaut
On September 14th, 2015, less than 72 hours after it began operation at it’s current sensitivity, an incredible event unfolded at each of the twin LIGO detectors in Washington and Louisiana: an event consistent with a gravitational wave signal from the merger of two massive black holes was observed! This direct detection — the very first for gravitational waves of any type — ushered in the dawn of a new kind of astronomy. It was the first time black holes of these masses, 29 and 36 solar masses, merging to form one of 62 solar masses, was ever observed. And it was a convincing, robust detection at greater than a 5-sigma significance match in each detector, independently. The fact that both detectors saw the exact same thing leaves very little doubt that this was, in fact, a gravitational wave signal.
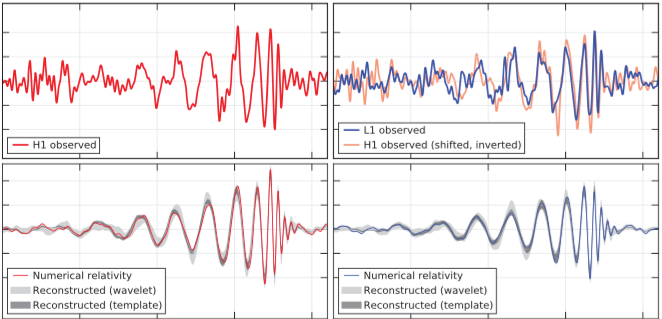
While there’s plenty to say about this, there’s simply no substitute for going straight to the source. In this case, that means going directly to Dr. Dave Reitze, scientist, professor and the executive director of LIGO!
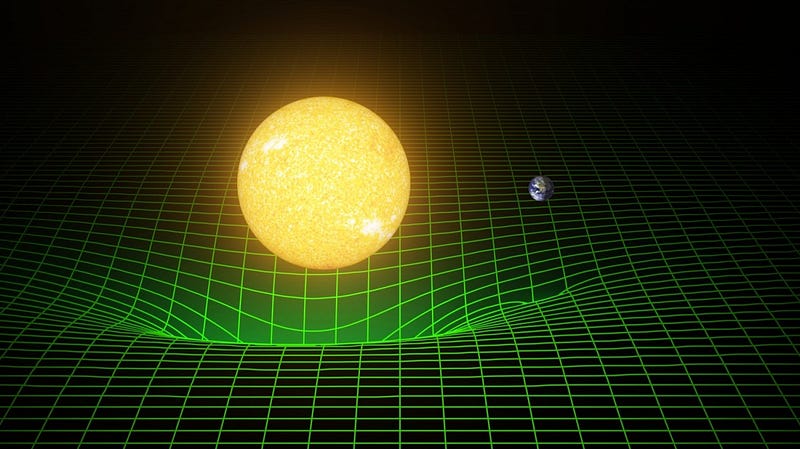
Ethan Siegel: A lot has been written about this discovery, but it must have been very different back in September when this signal first showed up just a few days after it started taking data. When these waves first came in, was it what you expected to see, or was it a surprise?
Dave Reitze: It was a surprise in terms of its amplitude: this was a very strong, loud signal. It was black holes, very few people would’ve predicted that binary black holes would’ve been the first thing we would’ve detected. It was black holes that are heavier than any other stellar mass black holes that have been observationally recorded. There are so many elements that are just, sort of, so out there!
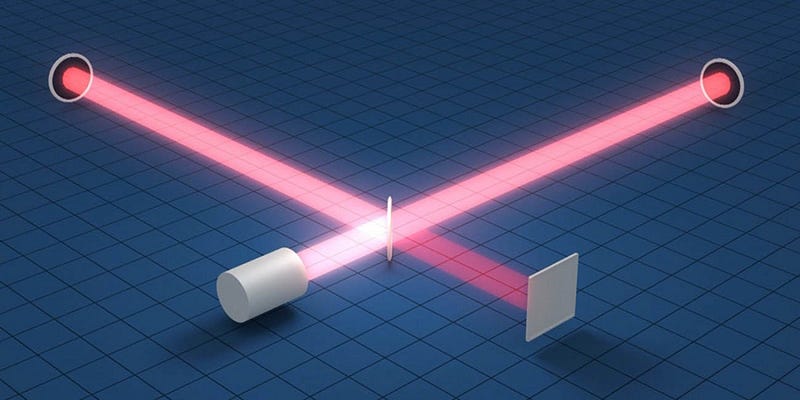
ES: What do you wish everybody knew about LIGO that hasn’t been given its due yet?
DR: I think one of the things that hasn’t gotten as much airplay as it should’ve is not so much about LIGO, but is about other detectors that are coming online and the roles they’re going to play. There are other detectors coming online: one is in Italy, the VIRGO detector, which hopefully will be online sometime this year, there’s a detector in the Kamioka mines [in Japan] called KAGRA coming online hopefully in 2019, and then India announced that they wanted to build a gravitational wave detector, which is something we’ve been pursuing for about four years.
Having those detectors come online will be crucial, because it will be the things that allows us to couple gravitational wave astronomy with [traditional astronomy done in the] electromagnetic. That’s the next step: to simultaneously see [gravitational waves] with three, four or five interferometers, localize it quickly, within minutes, and have other observatories catch it instantly, and catch it in the optical or the X-ray bands. That’s going to provide a whole new understanding in these cataclysmic events. It’s not just what happens now, it’s how much more rich this discovery space will be once these detectors come online. LIGO is great, but when all these detectors come online, that’s something that’s really going to be super great.
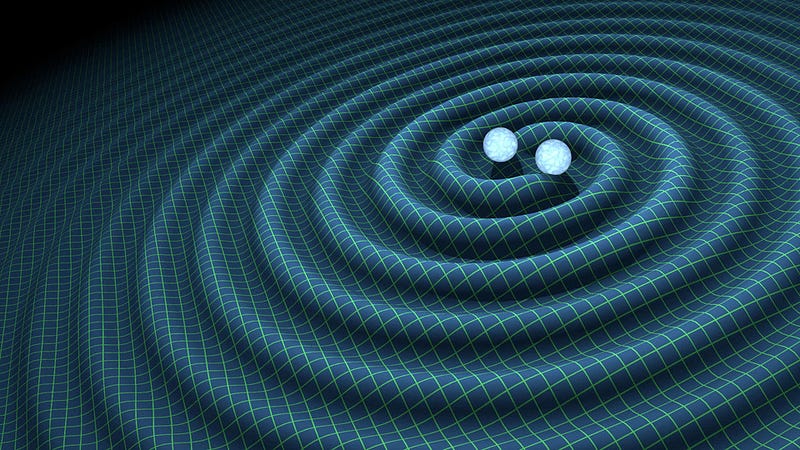
ES: The Advanced LIGO upgrade isn’t complete yet. When do you expect it to be finished, and how much more sensitive will it be than it currently is?
DR: We have a science design goal for our sensitivity as a function of frequency. By some measure, we’re roughly a third of the way from most of that design goal over different frequency spaces. We have this metric we call the binary neutron star inspiral range, the range at which we could see the binary merger of a neutron star, and where we’re operating now we’re somewhere between 70 and 80 Mpc. We want to get to 200 Mpc. Where I think the tough part is, in terms of making the detectors work right, is that at low frequency we have probably a factor of 10–15–20 (to improve) depending on where you are, and that opens up a whole new spectrum of black holes we could detect. And that probably is pushed out to 2018–2019–2020 in terms of reaching that design sensitivity. It turned out that nature was very kind, and there appear to be many of these black holes in the Universe and we were lucky enough to see one.
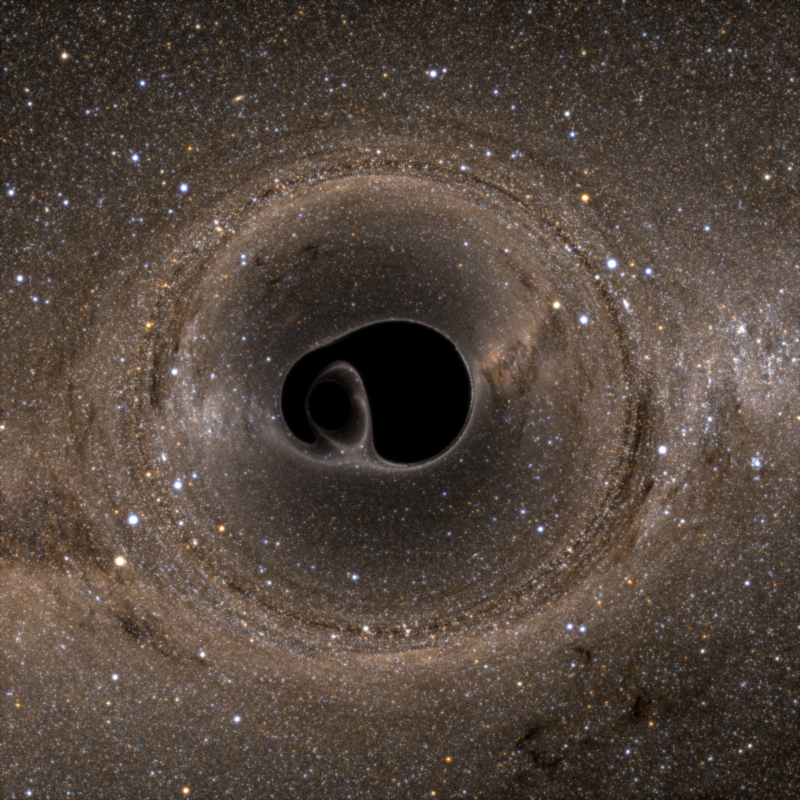
ES: The first announced event was estimated to have occurred at a distance of 1.3 billion light years. How far can LIGO realistically reach?
DR: With advanced LIGO, for these stellar mass black holes, we should be able to see out beyond 2 or even 3 Gigaparsecs, so call that 9 or 10 billion light years. For 100, 200 or 300 solar mass black holes, that range falls down again, because we’re losing sensitivity as the frequency gets lower. The neutron stars are higher frequencies, and those are also less sensitive: out to about 700 million light years. What do we do next? If we can make our instruments, say, ten times more sensitive over Advanced LIGO, we could see ten times as far.
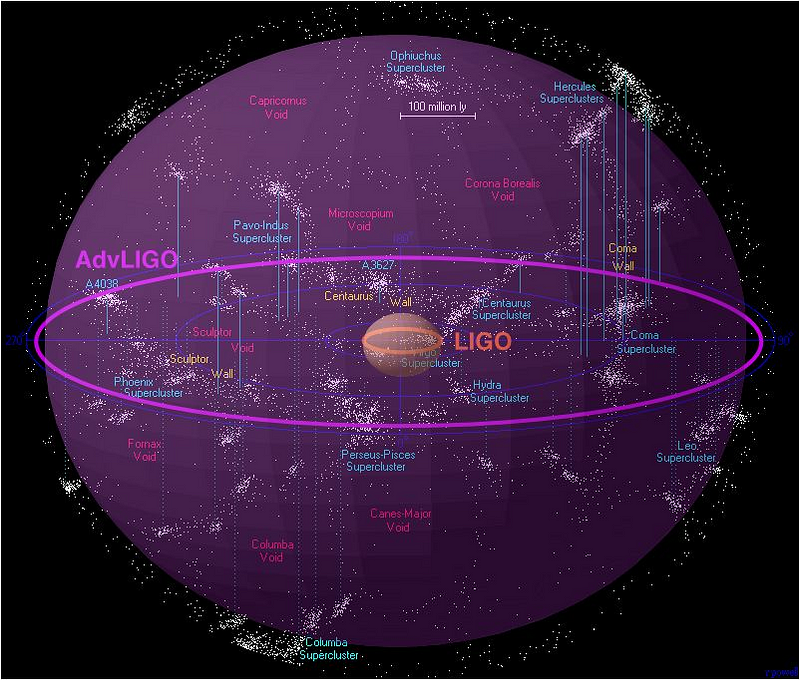
ES: What are the prospects for probing to the limits of the observable Universe (~46 billion light years)?
DR: For a future detector that could see a factor of ten over Advanced LIGO, you could pretty much see the whole Universe in terms of black holes, and could see neutrons stars merging out for billions of light years, out to near where the first stars formed. There are plans in place where we’re trying to build detectors — they’re at least 15 years away — but the prospects are good for building in the next generation of detectors. I think the future’s bright.
ES: People don’t typically appreciate the precision of the lasers, the vacuum through which they travel, the cooling apparatus or the insulation from noise that needs to occur for LIGO to work. What can you tell us about them?
DR: LIGO is a tour de force both in precision measurement and also in engineering. Being able to do experiments to demonstrate that you can measure things to the limits of a tiny, tiny fraction the diameter of a proton, to engineer that so you can do it day in and day out robustly, that’s a whole other level of effort. The interferometer is made up of different sub-systems: you need a laser, you need the mirrors, the beam splitter, a vacuum to put the interferometer in, the control systems to sense and control the positions of the mirrors, and then the angle, how you position the laser light so it’s aligned. There are also seismic isolation systems, because you have to filter out by about a factor of a trillion the seismic noise, both from the Earth’s natural movement and because there’s manmade noise.
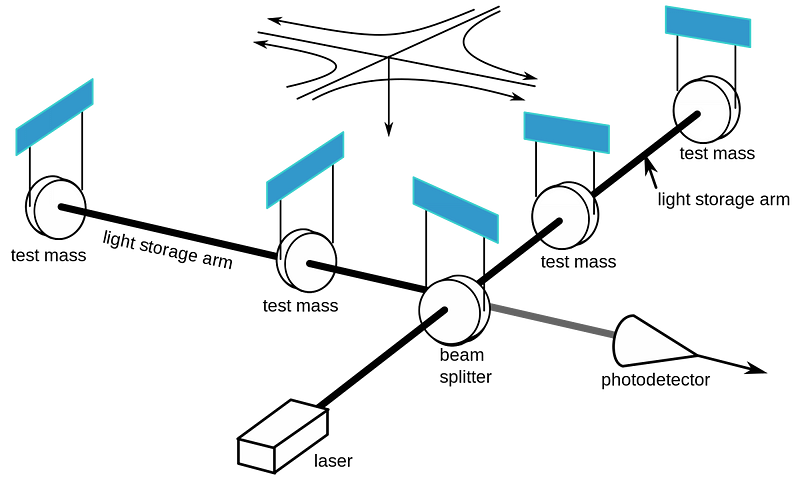
So let me pick one and talk about the input optics. The input optics is basically the first part of the optics for the interferometer, and it plays a very special role. The laser that we use is very stable, it’s the most stable laser in the world. But you can’t just put the laser light into the interferometer, because the laser beam isn’t the right size, it’s still too noisy — everybody thinks of laser light as being the purest light you can get but it’s not; there are different levels of purity — and to do the interferometry and measure those displacements of 10^-18/10^-19 meters, we need to do further purification. And we also have to change the character of the laser and put something called “side bands” on, so instead of having one monochromatic laser we have slightly different colors to have sensing light to read out some of the positions of the mirrors. You have to blow the beam up from the thickness of a pencil to maybe 6–7 cm, and then at the heart of it there’s something called the mode cleaner. It makes the light more stable in terms of frequency, amplitude and also in something called pointing, which control the angular fluctuations. The input optics does all of those things. It’s not one of the sexiest subsystems in terms of the interferometer, but it’s the most complicated part of the interferometer in that it interfaces with all the other parts of it. And that’s what University of Florida has contributed, and it works remarkably well.
ES: There are many things that can make gravitational waves at the high frequencies LIGO is sensitive to: black hole-black hole mergers, neutron star-black hole mergers, neutron star-neutron star mergers, supernovae and gamma ray bursts. But do any, other than black hole-black hole mergers, have a chance of being seen with their anticipated amplitudes?
DR: Certainly the black hole-neutron star source is one that we really do hope to see. There’s no observational support for it so far, even though that is supposed to be a candidate source for gamma-ray bursts, as are the binary neutron star mergers. The rate for those is highly non-constrained, which means until we see one or two, we really don’t know. The supernovae are a really interesting case. When LIGO was first conceived in the late 1970s and 1980s, supernovae were thought to be one of the really good sources of gravitational waves. But as people began to model supernovae better and understand core collapse and the subsequent shockwave and blow-off of the outer layers, they turned out to be rather poor radiators. So Advanced LIGO and even with the next generation, we might be unlikely to detect supernovae outside of our own galaxy.
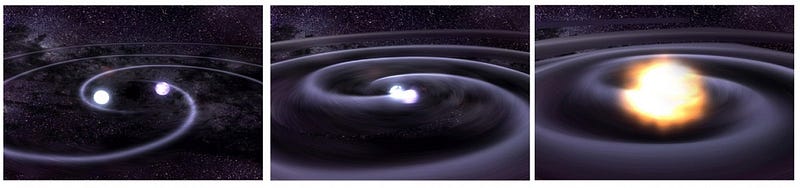
ES: Are there any unexpected surprises LIGO might find, or would we not see anything we don’t have a template for?
DR: The other interesting source — and if we saw it, it’d be really cool, but it’s a tougher source to see — we search for gravitational waves from isolated neutron stars, from pulsars. If there’s a mechanism that breaks the sphericity, that puts a time-dependent quadrupole mass moment (e.g., a crustal deformation, an elliptical shape to the neutron star, etc.), it will spin in such a way that there’s a wobble as its rotating around its axis. These gravitational waves will be very weak, but they’ll have the advantage that they’re very monochromatic, since neutron stars are very precisely clocked. We search for those over days, months and years, and we just keep integrating over time. If there is a signal that pops up above the background, eventually, if you integrate long enough, we’ll see it. Seeing something like that would be really exciting, because then you could say that gravitational waves contribute to the spinning down, to the slowing down of an isolated neutron star, of a pulsar.
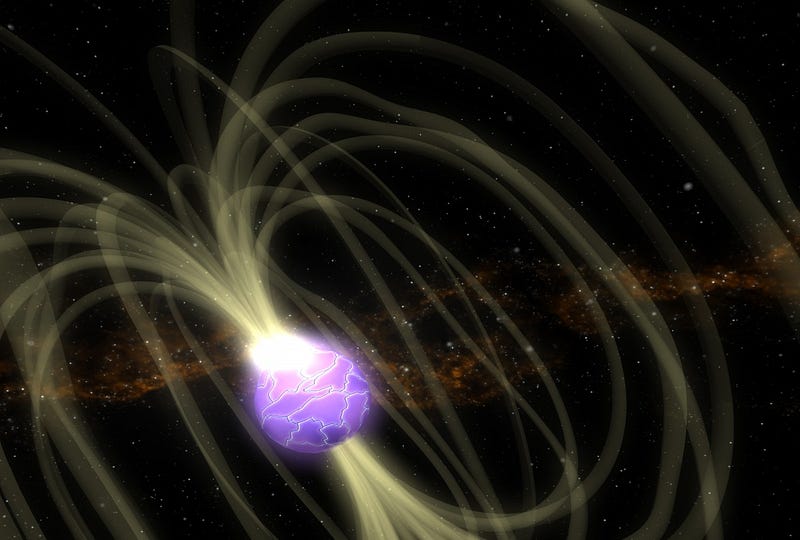
ES: So if we had a pulsar glitch within our galaxy, would LIGO have a shot?
DR: We absolutely could! It would have to be close, and it would have to be a pretty big glitch, but we search for those, actually. A glitch would be a burst-type event, where all the energy would be emitted at once, rather than a small signal that you integrated over a long time as in the above example. The pulsars are expected to spin down over perhaps billions of years, seeing a slow rate of change, and those searches are hard. The nice thing about a pulsar is that we have the radio information from pulsar timing: we know what the spin frequency is and what the gravitational wave frequency is and where they are in the sky. We have a much narrower parameter space, so we know what we’re looking for. I think the odds are long for Advanced LIGO, but you never know and that’s why we look.
ES: Steve Detweiler, our friend and colleague, just passed away suddenly of a heart attack last month. Is there anything you’d like to share about his role or impact on numerical relativity and on LIGO in particular?
DR: That was a shame; it was very sudden. Steve wrote one of the seminal papers for another type of gravitational wave detection on pulsar timing. He was always a little bit skeptical of LIGO; I’d see him in the hallway and he’d go, “Oh, so how’s LIGO going?” I’d say, “Oh, it’s going great!” He’d say, “When are you going to detect gravitational waves?” I’d say, “Oh, about five years,” and then he’d say, “yeah, everyone’s been saying that for 20–30 years!” The last time I saw him was five years ago, and I said, “this time it isgoing to be five years, it’s not going to be any longer than that.”
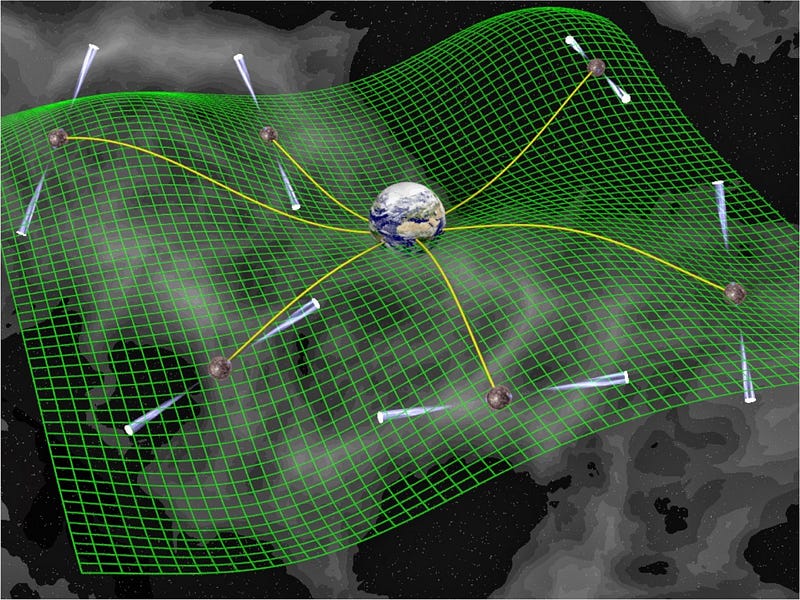
But he theorized that you could detect gravitational waves from pulsar timing using radio astronomy. You’d have to look not for days or weeks but years, and even 5–10 years. If you had enough pulsars located over points in the sky, you should be able to look at a difference in timing from those pulsars. From that difference in timing, you could infer the existence of a gravitational wave background at extremely low frequency gravitational waves: in the nanoHertz range. This is an experiment going on right now. There are a number of these experiments working together, the NANOGrav collaboration in the United States, one in Europe called the European Pulsar Timing Array and one in Australia called the Parkes Pulsar Timing Array, and they all share data and work together. They are potentially on the verge of making a discovery of these low-frequency waves using a method that was first proposed by Steve Detweiler, so in some sense I think Steve was a real pioneer there. Steve made really a seminal contribution to the field.
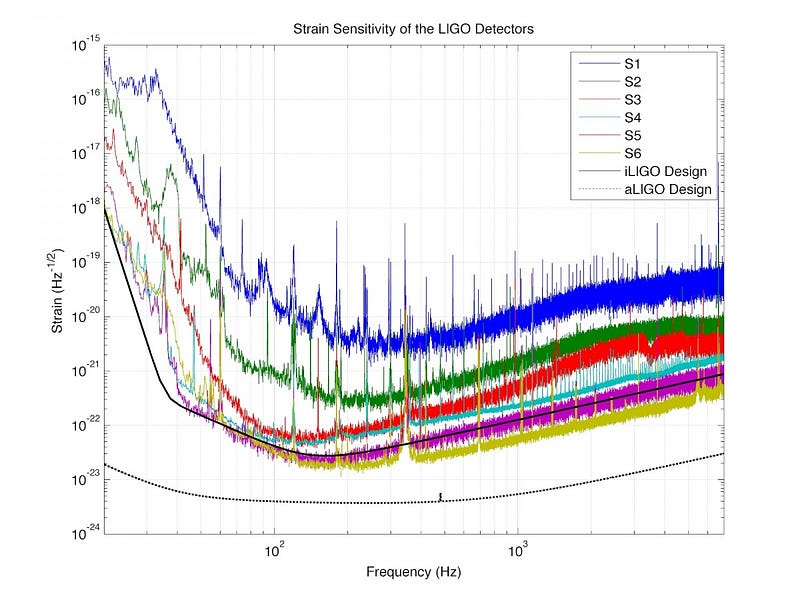
ES: Other than going into space, what are the prospects for increasing our sensitivity to gravitational waves via experiment?
DR: A lot of what we’re thinking about for making a new ground-based gravitational wave detector goes into thinking about how you suppress low-frequency noise: the noise that comes from the Earth. It’s really hard to envision how to build an Earth-based detector that goes below 1 Hz with any degree of precision. The Earth’s motion gets to you, but there’s also gravity gradient noise, which is also called Newtonian noise. Any time you have an object that’s moving, it’s changing the local gravitational field. The atmosphere is moving, the Earth is moving as there are surface waves going through it, people are driving cars and things like that. The problem with gravity is there’s no way to shield it; gravity goes through everything. In order to try and beat this Newtonian noise, you have to actually measure the stuff that’s moving around using seismometers and things like that, and then you have to account for it. I think we’re at a position where we can consider what type of monitoring network you’d need to weed out that noise, and… it’s a challenge. If you want to go below 1 Hz, you really do want to think about going into space.
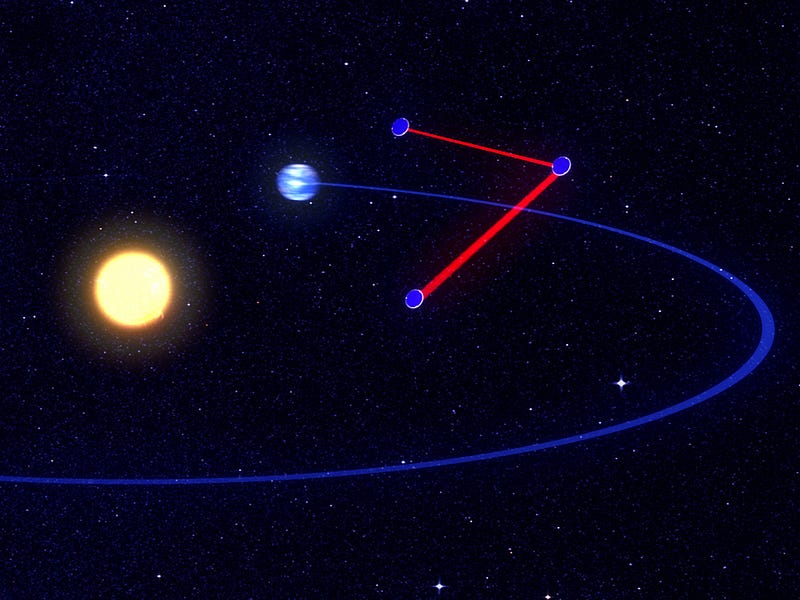
ES: What is your great hope for the future of gravitational wave astronomy, given the successes of LIGO so far?
DR: Oh! I think it’s all about cosmology. I think you want to get back to a bigger, better version of LISA. I think if there’s some path for NASA and ESA to join back together with some really significant contributions from NASA, you could envision a mission to do cosmology with some sort of distance ladder with gravitational waves. Gravitational waves have this property that they scale with the baseline of your detector — if you make your detector 10 times larger, you make it 10 times more sensitive — then if you make a ground-based detector with 40 km arms rather than [LIGO’s] 4 km arms, you can start to do experiments where you can start to see out far enough in the Universe then you can start to maybe measure cosmological parameters like w, the dark energy equation of state. I think ultimately, you’d like to see the cosmological gravitational wave background. I think there are a number of experiments that are thinking about how you could look in different frequency bands, and get a glimpse of the primordial gravitational wave background. I think that would be really revolutionary, because that would be your first glimpse at the very first instant of our Universe.
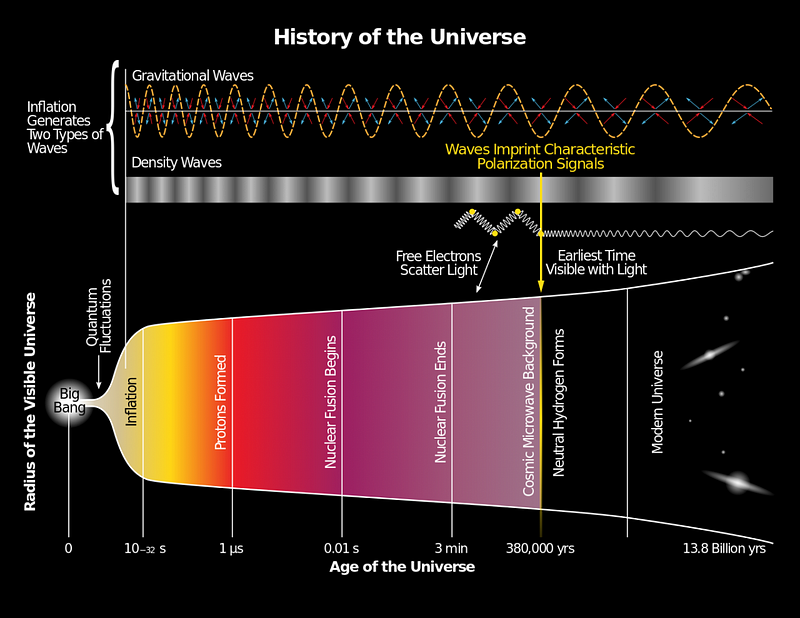
ES: And if we could see that, because gravitational waves from inflation are generated by an inherently quantum process, that would be a “smoking gun” signal that gravitation is an inherently quantum force, and that there must be a truly quantum theory of gravity out there.
DR: Right! Exactly! You’ve put it perfectly, that’s a perfect way to say that.
ES: What’s on the horizon for you, personally, now that LIGO’s finally detected its first gravitational wave event?
DR: Continue making our detectors better and seeing a lot more of them. I think that’s really the name of the game now: to show that LIGO can deliver on its promise of viewing the Universe with this new kind of tool, this new kind of detector, and start to see not only things we expect to see, but things we don’t expect to see. I think for me, it’s clear: I’m going to do my job to get the gravitational wave detectors working better, even beyond their current sensitivity states, and to start working more closely with astronomers to do this multi-messenger type of astronomy.
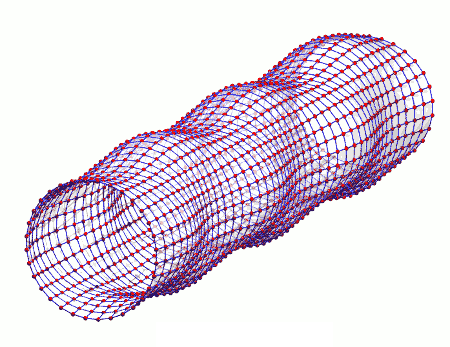
Another way to say this is that people who’ve been in this field have been wandering in the desert for 40 years — and I’ve been in it for 20 — and we’ve just entered the promised land. I’m sure there are going to be things we knew we were going to see but also things that we don’t, so let’s continue what I’m doing and get more excited as we see more things.
ES: And finally, what message would you most like to share with the general public who might be interested in gravitational wave physics, but doesn’t necessarily have expertise in it?
DR: There are a couple of messages. One message is the beauty of fundamental science and understanding our Universe. Even though gravitational waves are a very esoteric feature of a very complicated mathematical theory called General Relativity, which happens to work extraordinary well at explaining the way gravity works, even if you don’t understand the details, I think people can understand the wonder that comes with using these gravitational waves as messengers of understanding some of the most interesting phenomena in the Universe. Looking at two black holes colliding, you don’t expect to be able to observe them, in a general sense, in any other way. So I think there’s an exciting aspect to this, that we’re going to learn more about the Universe and how awe-inspiring it is, using gravitational waves.
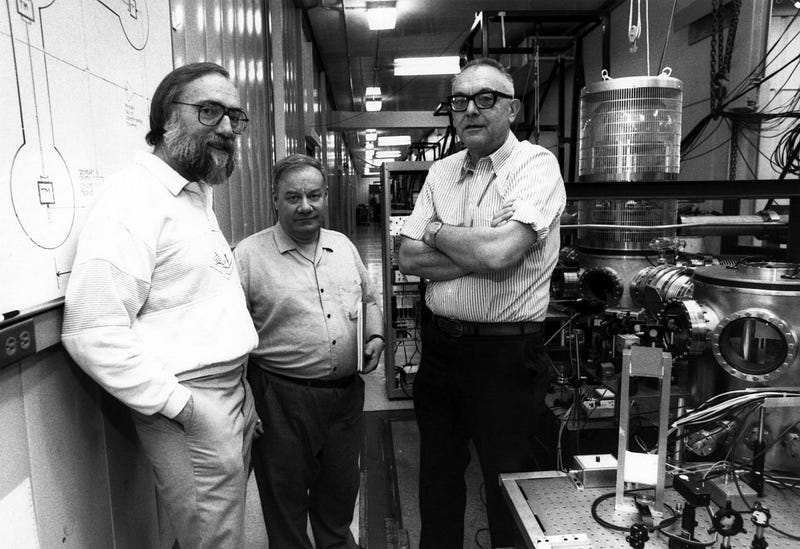
I think the other message is that the tool we’ve developed, and I want to point out that there are a couple of people who deserve credit for this — Rainier (Rai) Weiss at MIT, one of the first people who conceived of using interferometers to detect gravitational waves; Kip Thorne, who had the vision to realize this could be a new field of astronomy and hunted for people who were interested in building these sorts of detectors; Ron Drever who also made a lot of seminal contributions in terms of ideas for making interferometers — they came up with a tool that is really, really amazing technologically. It’s gotten to the point where we’re capable of making these mind-bogglingly tiny measurements of displacement, and from that inferring something about the nature of the distant Universe and black holes. When you look at it from the perspective of making a measurement that’s highly precise to measure a displacement of a fraction of an atom’s nucleus, from the standpoint that that’s what you need to do see these things like black holes, and the technology you need to develop, that’s awe-inspiring too. For me, as a scientist, that’s the kind of thing that gets me jazzed, that gets me excited.
This post first appeared at Forbes. Leave your comments on our forum, check out our first book: Beyond The Galaxy, and support our Patreon campaign!