Could dark matter not exist at all?

It’s said to be the majority of matter in the Universe, but how sure are we of that?
“For the moment we might very well call them DUNNOS (for Dark Unknown Nonreflective Nondetectable Objects Somewhere).” –Bill Bryson
Dark matter is described as making up the majority of matter in the Universe, and yet is very different from our every day experience. While our Sun is certainly the most massive thing in our Solar System, and is made of normal matter (i.e., protons, neutrons and electrons), there are plenty of other sources of matter that we know exist, including planets, gas, dust, plasma, and stellar remnants. But dark matter not only can’t be any of those things, it can’t even be made out of any of the particles in the Standard Model. Yet dark matter isn’t the only proposal for explaining the observed gravitational phenomena in the Universe. Another idea is to modify the theory of gravity itself, which many people have attempted to do. The idea of MOdified Newtonian Dynamics (MOND), as well as the other theories which have grown out of it, are by far the most popular alternatives to dark matter.
To understand what the big deal is, I want to go way back to the 1800s, and talk to you about a problem that existed long before the “missing mass” (or “missing light”) problem that dark matter and MOND attempt to solve: the problems of Uranus and Mercury. The law of gravity, put forth by Newton back in the 1600s, was spectacularly successful at describing everything — as far as we could tell — that it was applied to. From the motion of projectiles to rolling objects; from the weight of objects to the ticking of a pendulum clock; from the buoyancy of a boat to the orbit of the Moon around Earth, Newton’s gravity never failed.
In fact, Kepler’s three laws, a special case of Newton’s gravitational formula, applied to all the known planets equally:
- Planets moved in closed ellipses with the Sun at one focus.
- The area swept out by each planet as it orbited the Sun was the same in any given time interval at all points along the orbit.
- And the period of a planet’s orbit, squared, was proportional to the cube of its semimajor axis.
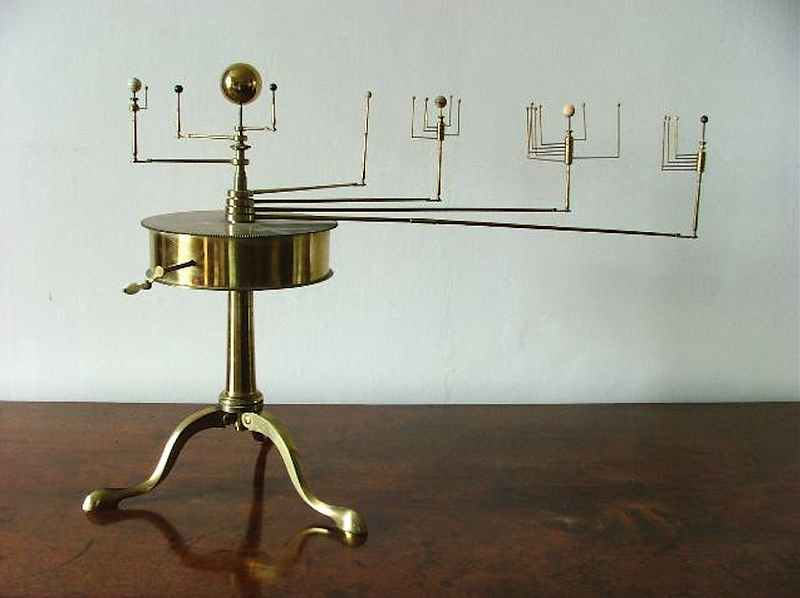
The known inner and outer worlds all obeyed these laws spectacularly, so much so that no deviations had been detected for hundreds of years. But with the discovery of Uranus in 1781, something changed. While the newest planet appeared to move in an ellipse around the Sun, it seemed to move at the wrong speed compared to the predictions of the laws of gravity.
For the first 20 years or so since its discovery, it moved faster, from night-to-night and year-to-year, than the laws appeared to indicate. For the next 20–25 years, however, the planet appeared to move right at the rate expected from those laws. But then it slowed down further, and its speed dipped below gravity’s predictions.
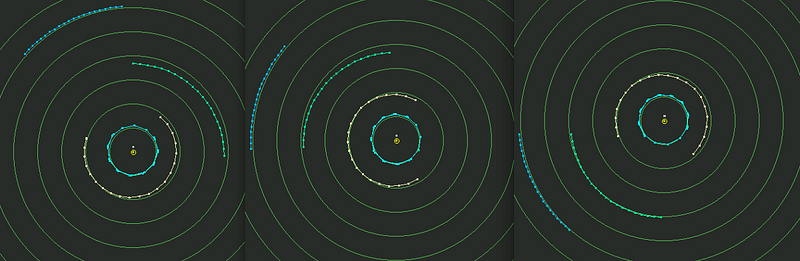
Was the law of gravity wrong? Perhaps. But perhaps there simply more matter out there — some type of unseen, or dark matter — that was pulling on Uranus, causing these orbital deviations. Indeed, that turned out to be the case. After a theoretical war between Urbain Le Verrier and John Couch Adams, both working independently and making predictions about where this new planet ought to be, Le Verrier’s predictions were confirmed by Johann Galle and his assistant, Heinrich d’Arrest, on September 23, 1846. The planet Neptune had been discovered, the first such object to have its existence predicted on account of the effects of its mass: of its gravitational influence.
On the other hand, the innermost planet, Mercury — thanks to increased observational precision coupled with centuries of data — had begun to show an even stranger violation of the laws of gravity. While Kepler’s laws predicted that planets should move in perfect ellipses with the Sun at one focus, that assumes there are no other masses disturbing or influencing that system. But there are other masses around, and Mercury doesn’t move in a perfect, closed ellipse. Rather, that ellipse precesses over time.
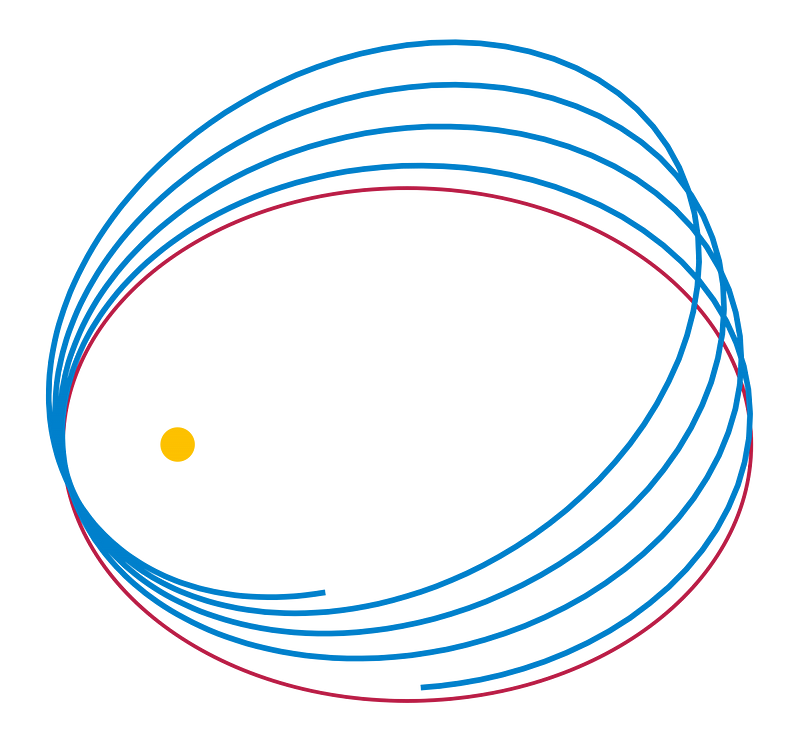
With Newton’s laws of gravitation, we could account for the effects of all the other known planets (including Neptune), as well as for the precession of Earth’s equinoxes. After doing all of this, we found that there was just a slightdiscrepancy left over between what was predicted and observed: a precession of just 43″-per-century, or just 0.012°-per-century. But that was no fluke.
So what was the explanation, this time? Was it a new, unseen mass, perhaps interior to Mercury? Or was it a real problem with the law of gravity? Exhaustive searches were undertaken for a new theoretical planet, Vulcan, closer to the Sun than had ever been observed. But there was (and is) no Vulcan. The solution came in 1915, when Einstein set forth his general theory of relativity.
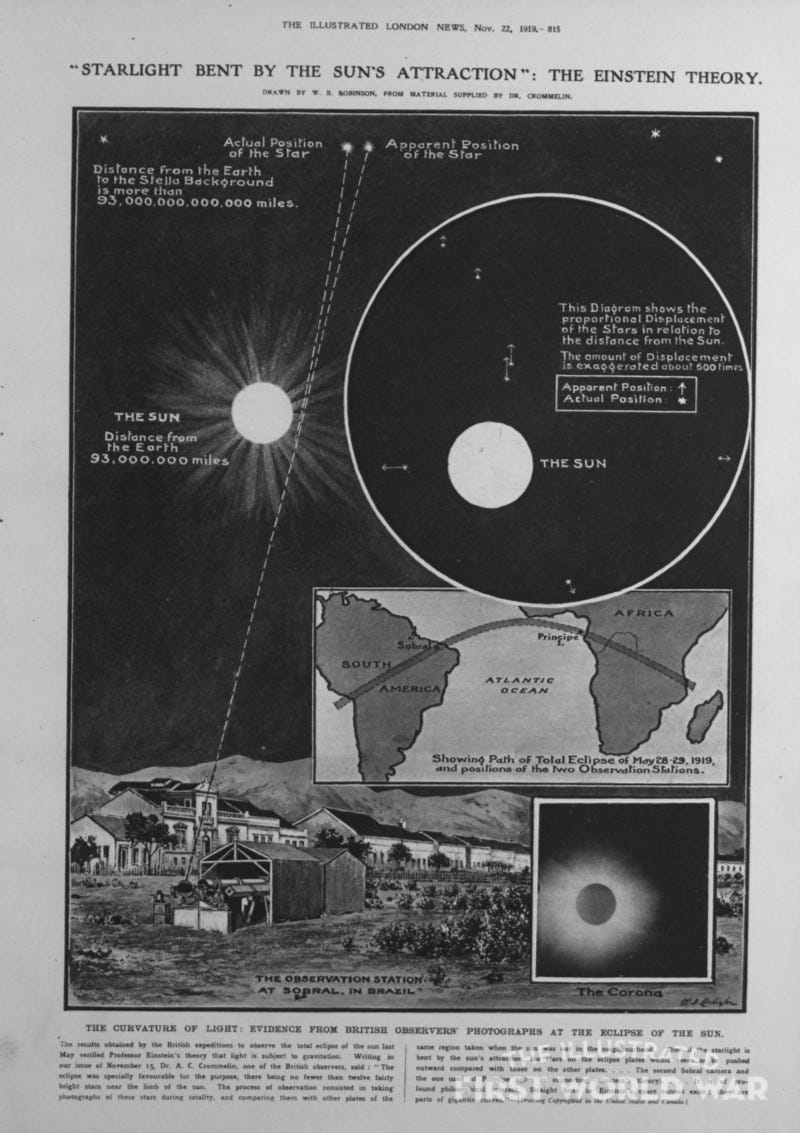
Now, come forward in time to the 1970s, and a scientific set of observations pioneered by Vera Rubin. We’re observing individual galaxies — particularly edge-on galaxies — and measuring their velocity profiles. We look at one side of the galaxy and see that it’s moving towards us (blueshifted), while we look at the other and see it’s moving away from us (redshifted), a consequence of galactic rotation. What we’d expect to find, similar to our Solar System, is that the interior stars should be rotating faster, with speeds falling off as we get farther and farther from the center. But that’s not what we find.
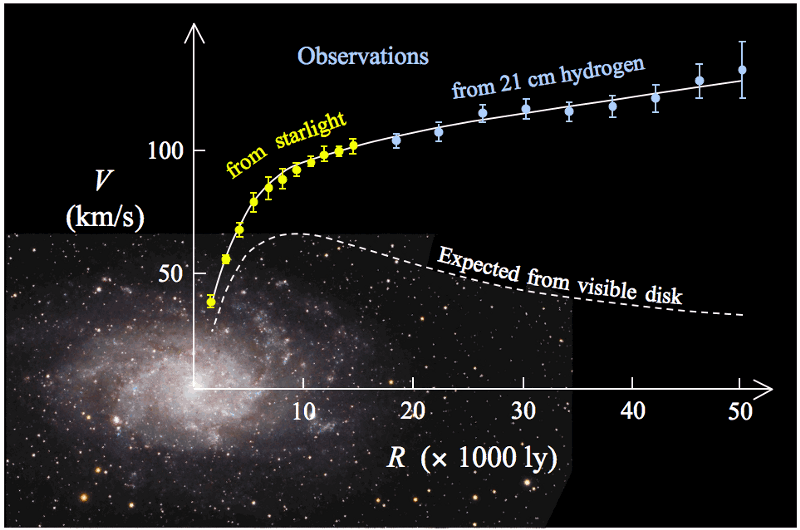
Instead, the rotational speeds of each individual galaxy remains constant as we go out to larger and larger distances. What could cause this? Again, the same two possibilities: either the laws of gravity need modification, or we need to hypothesize the existence of extra, unseen, invisible mass.
The MOND (MOdified Newtonian Dynamics) phenomenon was first noted in 1981 by Moti Milgrom, who observed that if we changed Newton’s law of gravitation at very small accelerations — something like fractions of a nanometer-per-second-squared — we could account for these rotation curves. Moreover, the same modification, a single, consistent one, could explain the rotations of all galaxies, from the smallest ones to the largest. MOND still does this today, and it does it very well.
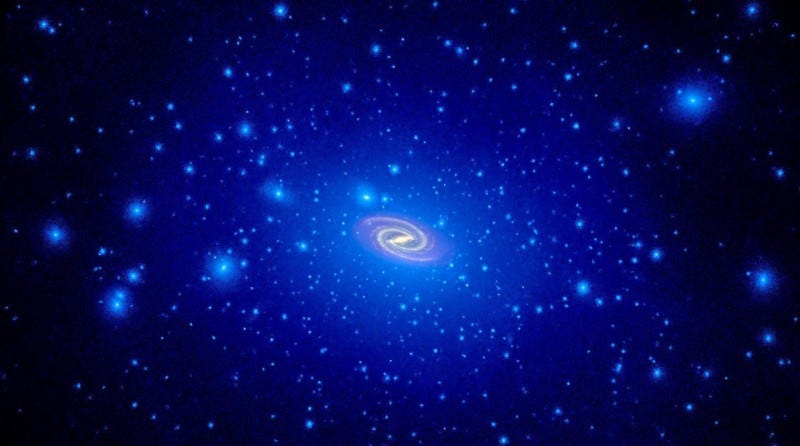
Dark matter, on the other hand, hypothesized that in addition to the normal particles of the Standard Model, and the normal matter of “protons, neutrons and electrons” that make up almost all of what we know, there was a new type of matter out there. To explain this rotational phenomenon, a large halo of matter that didn’t interact with light, that didn’t stick to itself, and that didn’t stick to normal matter was proposed. This was the idea of dark matter.
Dark matter can explain these rotation curves, but it doesn’t do it as well as MOND. The numerical simulations for the halos that the simplest dark matter models produce do not quite match up with the observations; the halos are too “cuspy” in the center and too “fluffy” at the outskirts. (In technical terms, they appear to be more isothermal than we expect.) If these rotation curves were all we had to go on, MOND would be the clear frontrunner.
But there’s a whole Universe out there. When you propose a new theory to replace an old one — as General Relativity replaced Newton’s laws — your theory has three burdens to meet:
- It must reproduce all the successes of the previous leading theory.
- It must successfully explain the new phenomenon (or phenomena) it was designed to explain.
- And it must make new predictions that can be experimentally or observationally tested, and confirmed or refuted, that are unique to this new theory.
We talk about all the successes of the previous leading theory, there are plenty.
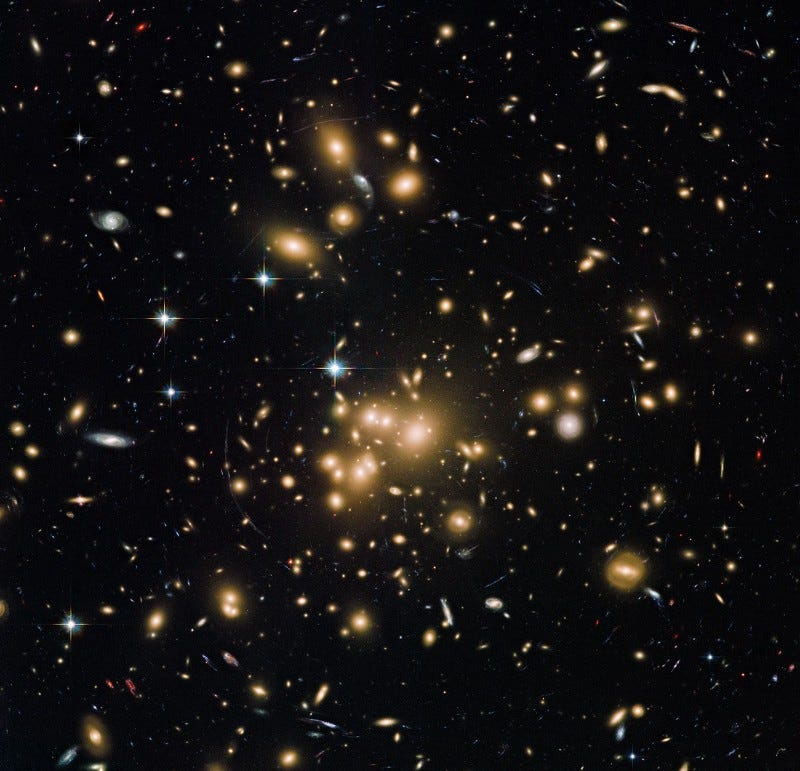
There’s the gravitational bending of starlight by mass, including strong and weak gravitational lensing. There’s the Shapiro time delay. There’s gravitational time dilation and gravitational redshift. There’s the framework of the Big Bang and the concept of the expanding Universe. There are the motions of galaxies within clusters and of the clustering of galaxies themselves on the largest scales.
For all of these — all of them — MOND fails spectacularly, either offering no predictions or predictions that woefully conflict with the available data. Perhaps if you argue that MOND was never intended to be a full theory, but rather a description of one phenomenon that might lead to a fuller theory, you can keep your hopes alive. There are many people working on extensions of MOND that could explain these observations, but there are no good successes so far, including TeVeS (Tensor-Vector-Scalar gravity by Bekenstein), MoG (Modified Gravity by John Moffatt), and others.
But if you keep Einstein’s law of gravity and simply add in a new ingredient, this collisionless, cold dark matter, you can explain it all, including some spectacular, novel nuances.
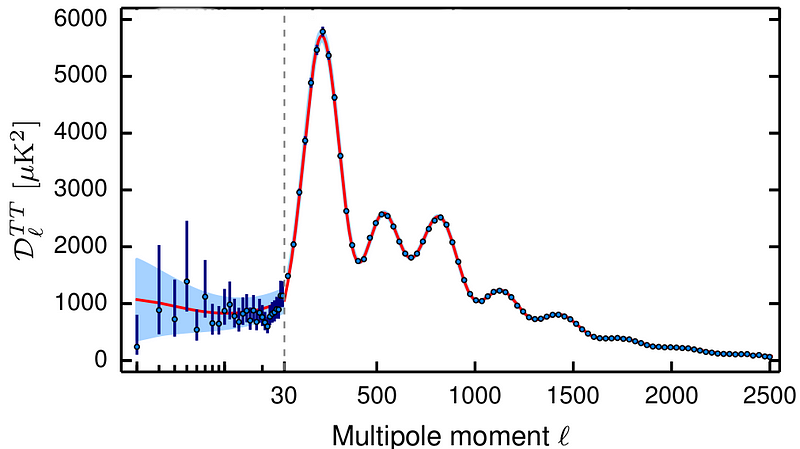
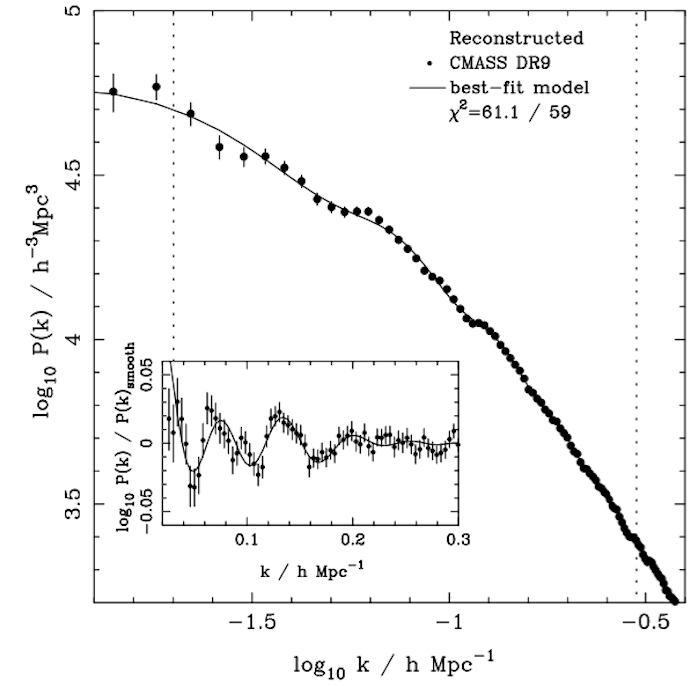
You can explain the clustering pattern seen in the large-scale structure of the Universe, including the large “curve” shape, above, and the “wiggles” in the curve, by having about five times as much dark matter as you do normal matter.
And most spectacularly, you get an entirely new prediction: that when you get two clusters of galaxies colliding, the gas inside should heat up, slow down and emit X-rays (in pink, above), while the mass that we can see through gravitational lensing (in blue, above) should follow the dark matter, and bedisplaced from the X-rays. This new prediction has been borne out observationally and held up over the past decade, a spectacular indirect confirmation of dark matter.
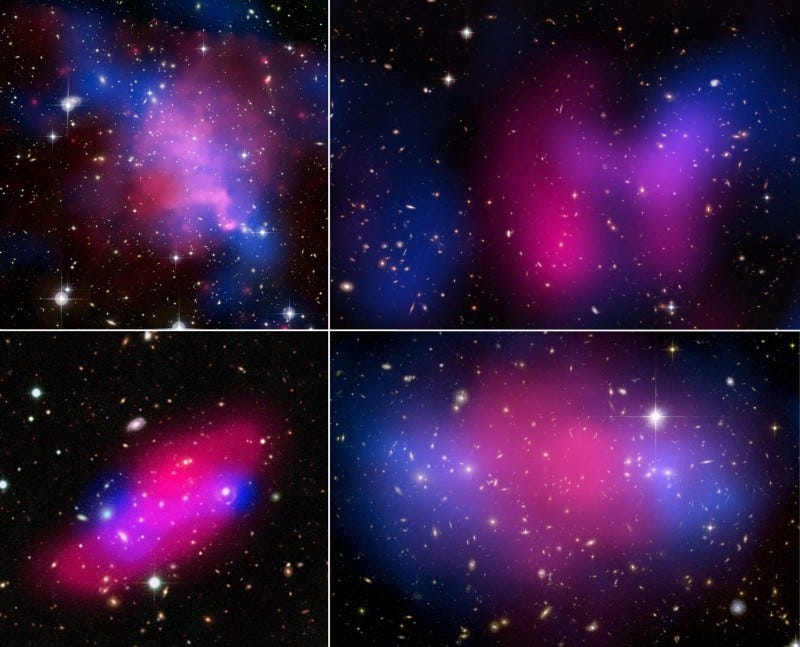
MOND does have a big victory over dark matter: it explains the rotation curves of galaxies better than dark matter ever has, including all the way up to the present day. But it is not yet a physical theory, and it is not consistent with the full suite of observations we have at our disposal. The reason you hear about dark matter is because it can give us the entire Universe, consistently, with the same single modification. MOND may yet turn out to be a clue to a fuller theory of gravity, and there are many who hope to someday derive the phenomenology of MOND from dark matter itself, a very ambitious project indeed!
But at present, MOND’s failures, cosmologically, make it far disfavored when compared to dark matter. It has its adherents and deserves to be considered and worked on, but it is not yet a viable alternative. Make a version that meets those three criteria, though:
- Reproduces all of GR’s successes,
- Explains the suite of new, known phenomena,
- And makes new predictions that can be tested and verified,
and I’ll change my tune, just as any good scientist should.
This post first appeared at Forbes. Leave your comments on our forum, check out our first book: Beyond The Galaxy, and support our Patreon campaign!