Throwback Thursday: How Do Black Holes Get So Big, So Fast?
The Universe contains black holes billions of times as massive as our Sun.
“It is by going down into the abyss that we recover the treasures of life. Where you stumble, there lies your treasure.” –Joseph Campbell
As we look out into the Universe, farther and farther, we see galaxies as they were further back in time. In the most extreme cases, we can see back to when the Universe was just a few percent its current age: hundreds of millions of years old, rather than more than 13 billion.
Yet when we look out at these most distant objects, we find that some of them have supermassive black holes at their cores that must be billions of times the mass of our Sun! You’d be reasonable to worry how they came to be so big in such a short amount of time. But as it turns out, the problem is even worse than you imagined, and it all goes back to the astrophysics of stars.
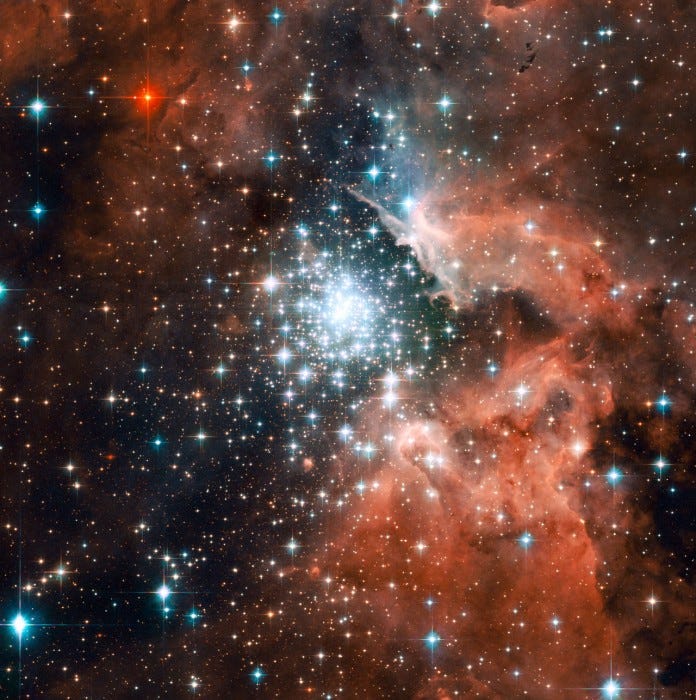
You’re very likely used to the idea that stars come in a huge variety of sizes, colors, lifetimes and masses, and that these properties are all related to each other. The more massive a star is, the larger its fuel-burning core — operating under the principles of nuclear fusion — is, too. This means that more massive stars burn more luminously, have hotter temperatures, tend to be larger in radius, and also burn through their fuel more quickly.
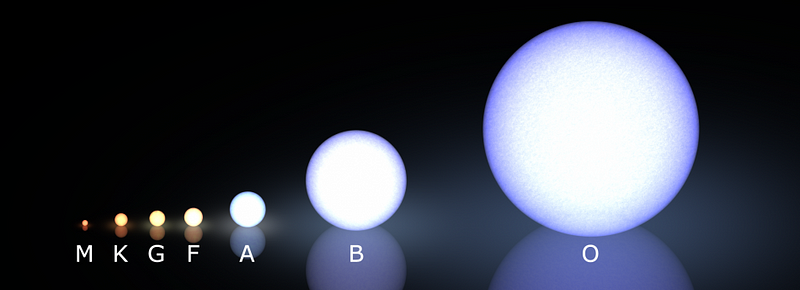
While a star like our Sun might take more than 10 billion years to burn through all the hydrogen fuel in its core, stars can be tens or even hundreds of times more massive than our Sun is. Instead of billions of years, they can fuse all the hydrogen in their cores into helium in only a few millions — or in extreme cases, possibly only hundreds of thousands — of years.
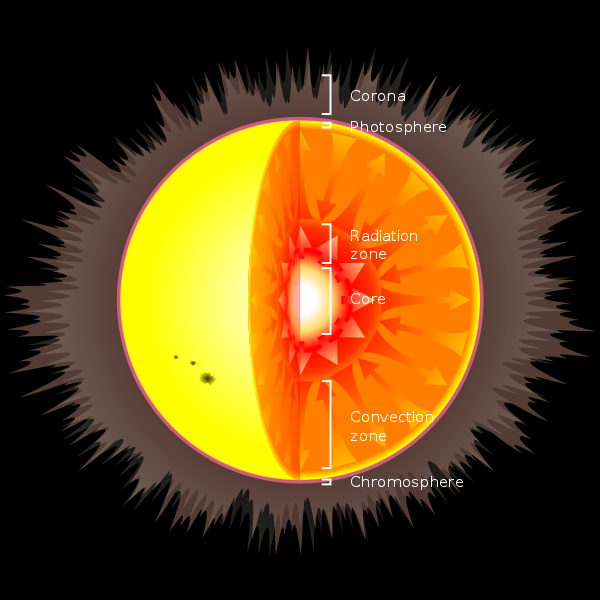
What happens to those cores when they use up their fuel? You’ve got to realize that the energy released from those fusion reactions — where light elements become heavier ones, releasing energy through Einstein’s famous E = mc^2 — was the only thing holding the cores of these stars up against the tremendous force of gravity.
Gravity, remember, is consistently working to contract all the matter in this star down into as tiny a volume as possible. When those fusion reactions cease because you run out of fuel, the core contracts quickly. The speed is important, because if you compress something slowly, its temperature tends to stay constant but its entropy rises, while if you compress it quickly, its entropy stays constant but the temperature goes up!
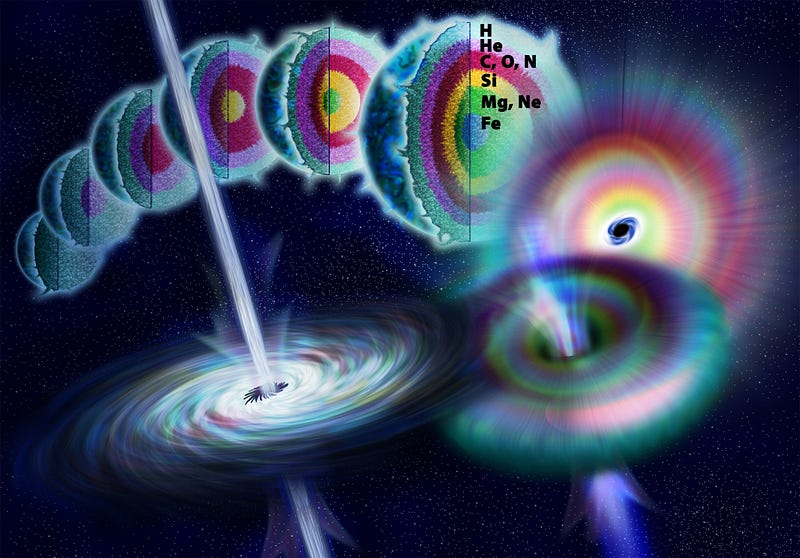
In the case of an extremely massive star’s core, that increased temperature means it can start fusing heavier and heavier elements, going from helium to carbon-nitrogen-and-oxygen to neon, magnesium, silicon, sulphur and eventually up to iron-nickel-and-cobalt in short order. (Note that these are mostly formed in increments of two, element-wise, due to helium nuclei fusing with the existing elements.)
When you reach iron-nickel-and-cobalt in the core — the most stable elements (on a per-nucleon basis) — there is no more fusion that can occur, since you’d actually lose energy by making heavier elements. So what happens then, when you’re out of material to fuse but you still have gravitation trying to pull everything together?
You get runaway core-collapse, resulting in a Type II supernova!
In a less-massive star that does this, you’ll get a neutron star at the core, while an even more massive star — with an even more massive core — won’t be able to stand up to gravity, creating a central black hole! A star about 15–20 times the mass of our Sun ought to produce a black hole at the center when it dies, and progressively more-and-more massive ones will produce even more massive black holes!
You could imagine huge numbers of massive enough stars producing black holes via this mechanism in a concentrated space, and then these black holes merging together over time. Or, perhaps, a combination of mergers to build up a substantial black hole, followed by feeding on stellar and interstellar matter, which we observe happening as well.
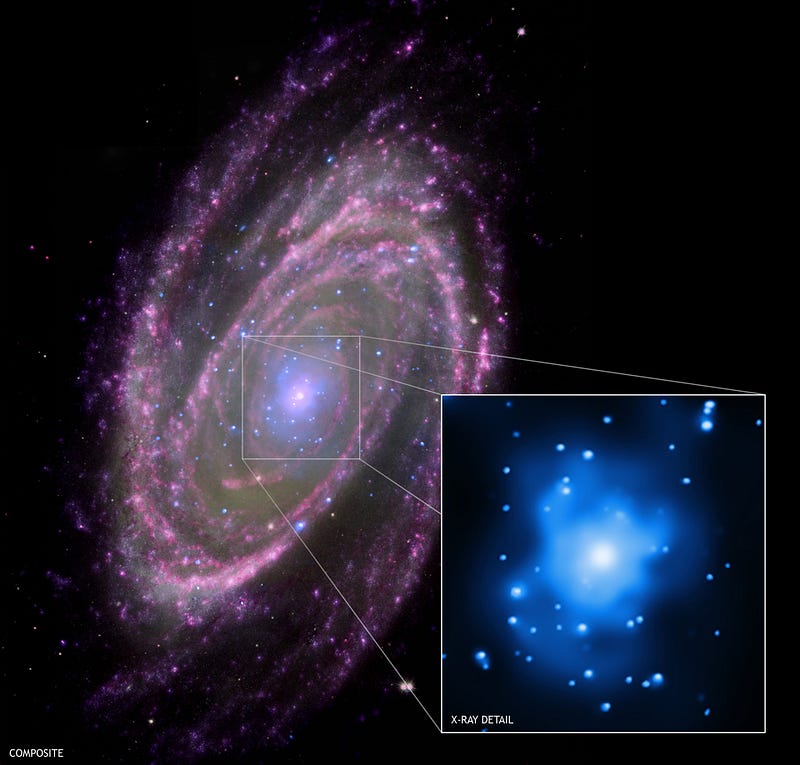
Unfortunately, that wouldn’t get you to the masses required quickly enough to be consistent with our observations.
You see, if a star gets too massive, it won’t produce a black hole at its center! If you start looking at stars over about 130 solar masses, the interior of your star becomes so hot and energetic that the highest-energy radiation particles you create can form matter-antimatter pairs, in the form of positrons and electrons. This might not seem like a big deal, but remember what was happening inside the cores of these stars: the only thing holding them up against core collapse was the pressure created by the radiation resulting from nuclear fusion! When you start producing electron-positron pairs, you’re producing them out of the radiation present in the star’s core, which means you reduce the pressure in the core. This starts to happen in stars of about 100 solar masses, but once you get up to about 130 solar masses, this reduces the pressure enough that the core starts to collapse, and it does so quickly!
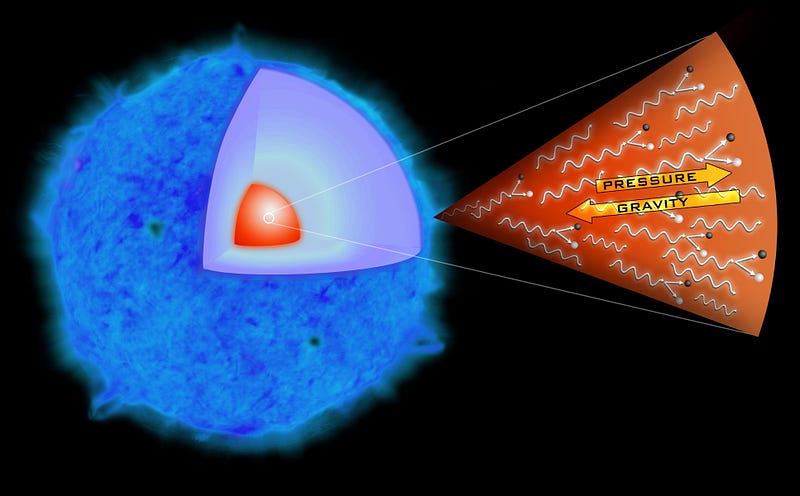
So it heats up, and it also contains a huge number of positrons, which annihilate with normal matter, producing gamma-rays which also heat up the core even further! Eventually, you create something so energetic in the core that the entire star is blown apart in the most spectacular type of supernova we’ve ever observed: a pair-instability supernova! This not only destroys the outer layers of the star, but the core as well, leaving absolutely nothing behind!
Without sufficiently large black holes formed in very short order in the Universe, we still might get supermassive black holes like the ones we find at the center of our own galaxy, which — from the gravitational orbits of stars around it — weighs in at a few million solar masses.
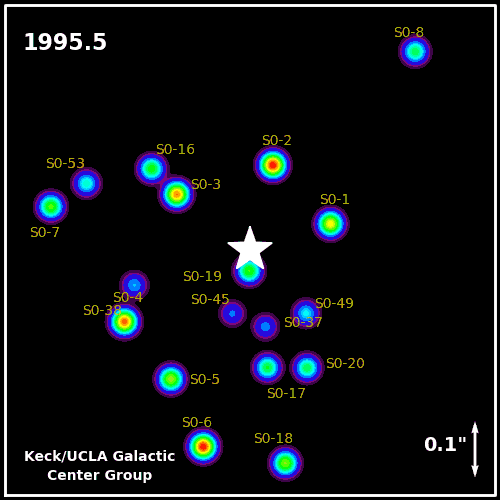
But that wouldn’t get you up to the billions of solar masses found in, for example, this relatively nearby galaxy (as you can see from its ultrarelativistic jet, below): Messier 87.
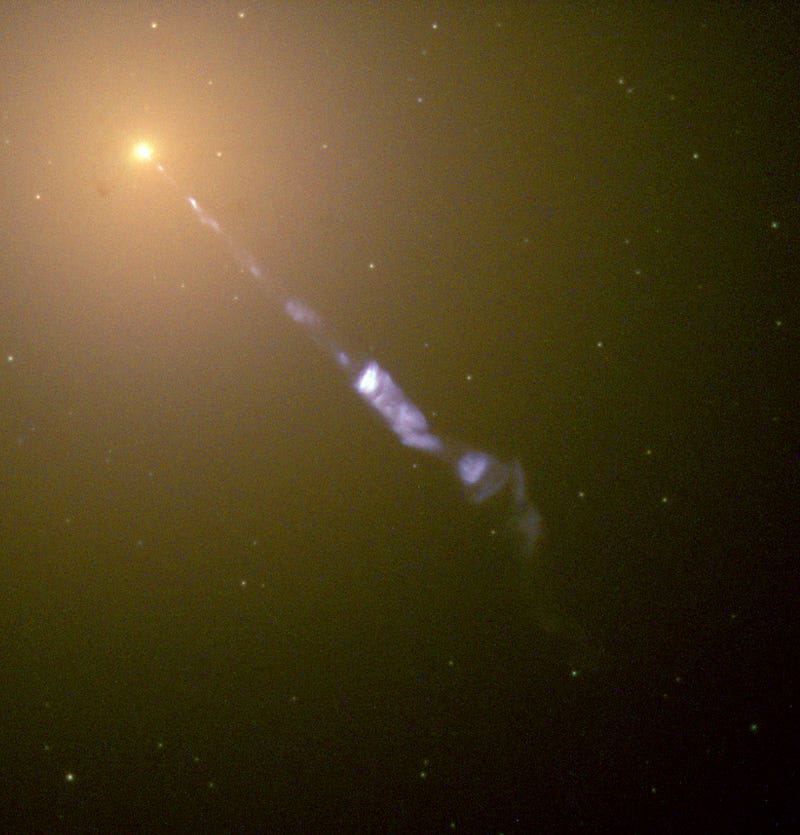
Supermassive black holes on this order — with many billions of solar masses — are not only found nearby, but also at very high redshifts, which means they’ve been around, and they’ve been very big, in the Universe for a long time!
You might think that we could have just started off the Universe with black holes of this magnitude, but that’s simply inconsistent with our picture of the young Universe, from both the matter power spectrum and the fluctuations in the cosmic microwave background. Wherever these supermassive black holes came from, it’s unlikely that they were primordial in nature, but they’re certainly present in even very young galaxies!
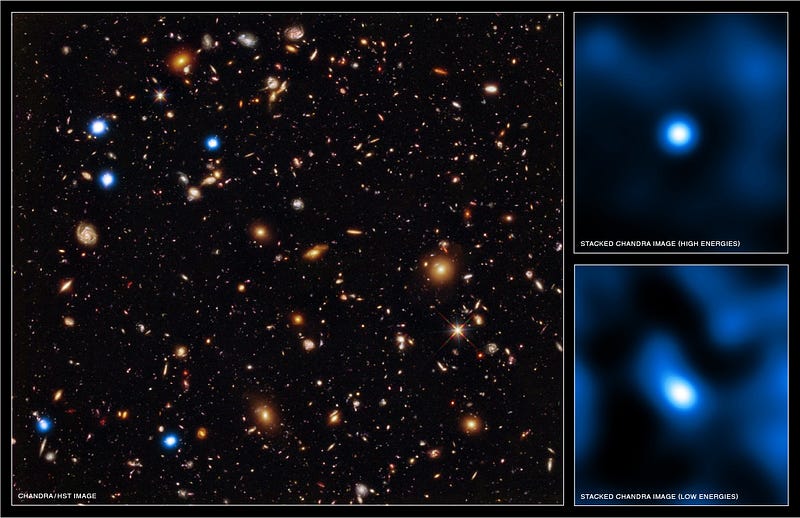
So if normal stars can’t make them, and the Universe wasn’t born with them, where do these young, supermassive black holes come from?
It turns out that stars can get even more massive than the ones we’ve talked about, and when they do, there’s a new hope. Let’s go back to the first stars that formed in the Universe — out of the primordial hydrogen and helium gas that existed back then — just a few million years after the Big Bang.
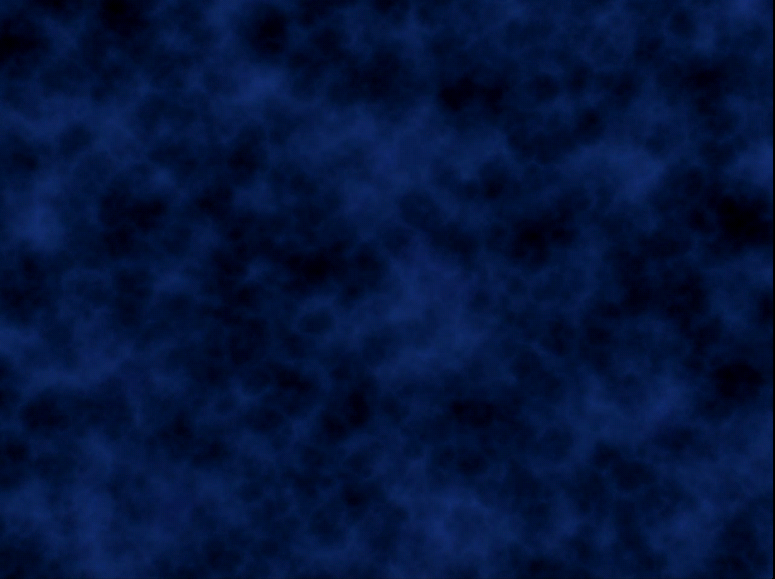
There’s plenty of evidence suggesting that very early on, the stars that formed were formed in huge regions, not like the star clusters containing a few hundred-or-thousand stars in our galaxy, but containing millions (or even hundreds of millions) of stars when they’re born. And if we look to the largest star forming region we have locally — the Tarantula Nebula located in the Large Magellanic Cloud — we can get a clue as to what we think is going on.
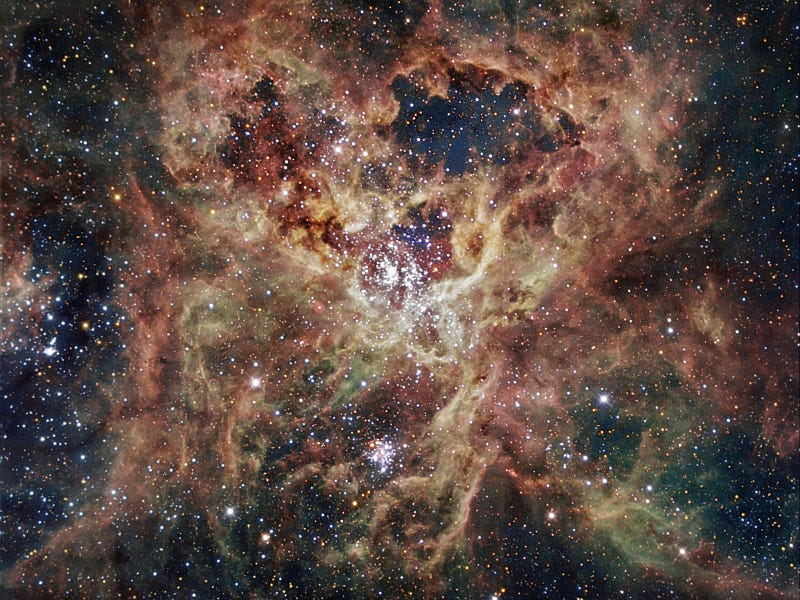
This region of space is nearly 1000 light years across, with the massive star-forming region in the center — R136 — containing about 450,000 solar masses worth of new stars. This entire complex is active, forming new, massive stars. But at the center of this central region, you can find something truly remarkable: the most massive star known (so far) in the entire Universe!
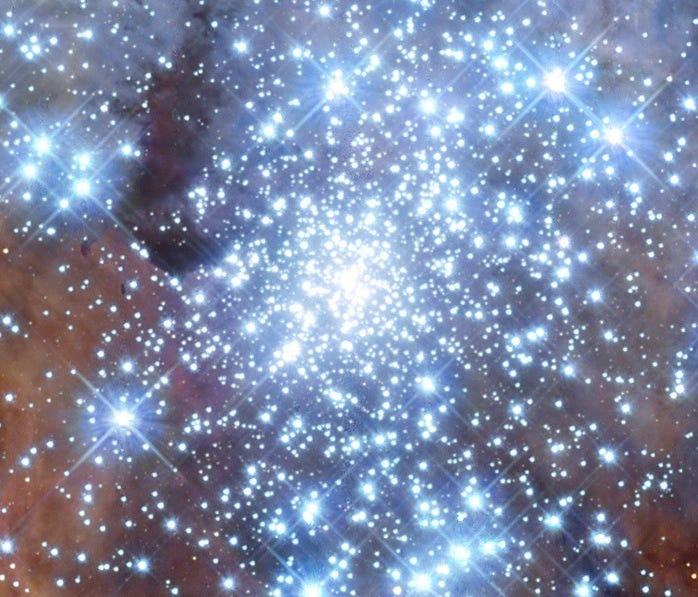
The largest star in here is 256 times the mass of our Sun, and that’s a very remarkable place to be. You see, remember what I told you about pair-instability supernovae, and how they destroy stars over 130 solar masses, leaving no black hole behind? That’s true, but it’s only true up to a point; that story is only true for stars with masses above 130 solar masses and below 250 solar masses. If we get even more massive than that, we begin to create gamma rays that are so energetic that they cause photodisintegration, where these gamma rays cool down the interior of the star by blowing the heavy nuclei back apart into light (helium and hydrogen) elements.
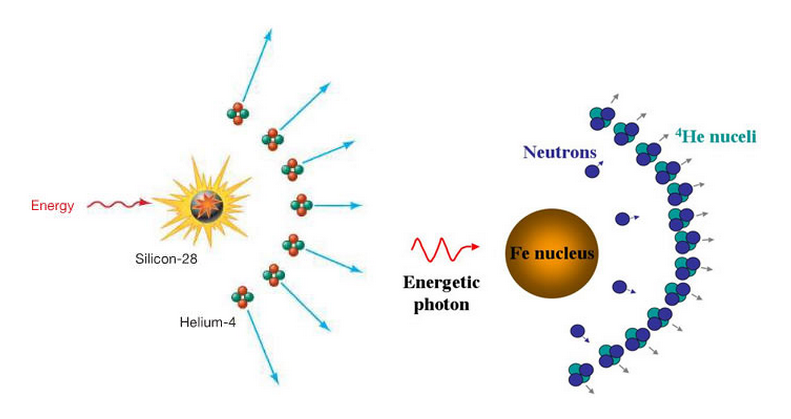
In a star with more than 250 Solar Masses, it simply collapses entirely into a black hole. A 260 solar mass star would create a 260 solar mass black hole, a 1000 solar mass star would make a 1000 solar mass black hole, etc. And so if we can make a star that exceeds that limit here, in our own isolated little corner of space, then we certainly made these objects when the Universe was very young, and we probably made a good-sized number of them. And over time, they’ll merge!
And if you can get an initial region kicked off with a massive black hole of a few thousand solar masses after just a few million (or few tens of millions of) years, the rapid merger and accretion of these collapsed, star-forming regions make it unthinkable that these early, large black holes wouldn’t merge with one another and grow. In short order, they’d be forming increasingly larger and larger black holes at the centers of these objects: the Universe’s first large galaxies!
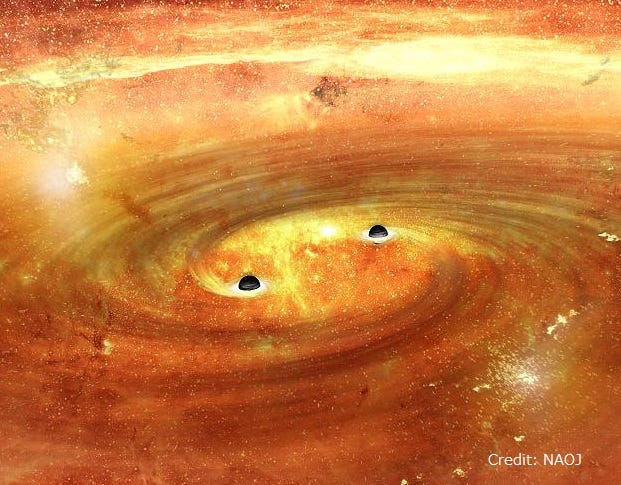
And that continued growth-over-time could easily result from some naïve estimates into a black hole of many hundreds of millions of solar masses for a Milky Way-sized galaxy. It’s not hard to imagine that more massive galaxies — or nonlinear effects — could ramp that up into the billions of solar masses without a problem. And although we don’t know for sure, that’s where we think, to the best of our knowledge, the most massive black holes in the Universe come from!
Leave your comments at the Starts With A Bang forum on Scienceblogs!