The “inflaton” could shed light on the mystery of the early Universe
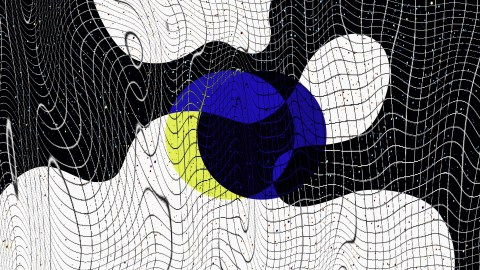
- When physicists don’t have data, they extrapolate from current models. This helps us explore new possibilities and their consequences. But it must be done with care.
- The most popular extrapolation about the very early Universe uses a field called the inflaton to change the way the Universe expanded for a short period of time.
- This approach could solve some problems in our current understanding of cosmology — but it generates new ones.
As the Universe expands, galaxies move away from each other. This movement is not in the way of shrapnel flying off from an exploding point — that is not what the Big Bang was. It happens because the galaxies are being carried along by cosmic expansion. They are like corks floating down a stream, and their receding motion is called the cosmic flow. The expansion of the Universe is an expansion of space itself, which can be loosely considered as a sort of elastic medium completely intermingled with the matter and energy in it. As the great American physicist John Archibald Wheeler wrote, “Matter tells space how to bend and space tells matter how to move.”
If we look back in time, we see matter squeezed into smaller and smaller volumes. As this happens, temperature and pressure rise, and the bonds that hold stuff together into molecules, atoms, and atomic nuclei are progressively broken. Reach back far enough in time, to about one-trillionth of a second after the Big Bang, and the Universe is filled with a primordial soup of elementary particles, all zooming around and colliding furiously with each other.
Twelve particles to bind them all
Countless experiments have verified this extraordinary picture of the early Universe. In the process, we reached an understanding summarized in the standard model of particle physics: There are 12 elementary particles of matter — six quarks and six leptons. The most famous of these are the up quarks and down quarks that constitute protons and neutrons, along with the electron and its neutrino, which are two of the leptons.
It is remarkable that all the atoms of the periodic table are made of just three particles — the up and down quarks and the electrons — and that the hundreds of other particles we find in particle collisions can be constructed out of the 12 quarks and leptons. Then we consider the Higgs boson, which gives the elementary particles their mass. In the early Universe, the ingredients of the primordial soup come from these known particles. (Perhaps they included some particles still unknown, however. This would be the case if dark matter is, as we believe, made of other kinds of particles — particles that may be present in dark stars.)
If we translate the energies at which these particles collide to the physics of the early Universe, we reach close to understanding the beginning of the Universe — all the way back to that time one-trillionth of a second after the Big Bang. This sounds small to us, but for particles it is quite a long time. Still, we can state with some reservation that we understand the basics of what was going on in the Universe at this early stage.
Mapping the unknown
Of course, we want to know what happened even earlier. We want to reach as close as possible to the Big Bang, t = 0. How do we do that when our experiments cannot reach the high energies present at the beginning? Well, we extrapolate. We take the theories we know to work, as exemplified in the standard model, and we push them to higher and higher energies. This might sound like pure guesswork, but it is not. The theories that describe how particles interact, called quantum field theories, allow us to scale the strength of the interactions to higher and higher energies. Within the limitations of our models, we can predict how the particles would interact if we probed them at higher energies. We can then take these high-energy models and transplant them to the early Universe to explore what could happen as we approach the Big Bang.
In doing this, we are of course drawing maps of an unknown territory. We extend our current knowledge beyond what we know to be true. For example, new forces of nature could become relevant at much higher energies. Maybe new particles emerge and play an important role. Many of the extrapolations used to populate the physics of the early Universe do exactly this — they make up possible scenarios based on new forces and new particles to explore what could have happened. If we are charting the unknown, we might as well be adventurous and use our imaginations as far as our current knowledge allows.
It is a peculiar feature of knowledge that we only know what we know, but we must use what we know to learn more than we do. Sometimes we get lucky, and new discoveries and novel experiments guide us forward. Unfortunately, this is not what is happening now. Quite the opposite — our extensive searches for physics beyond the standard model have not given us even a small taste of what may lie beyond. Our current extrapolations, then, must be taken with a very large grain of salt.
Answering new questions about the Universe
Take as an example the most popular scenario currently for the very early Universe. In this formulation, a field very much like the Higgs dominated physics and dictated how the Universe behaved, even if only for a fraction of a second. This field, which we sometimes call the inflaton, promoted an ultrafast expansion of the Universe.
Why is this good? In principle, this rapid expansion would solve a few problems with our current understanding of cosmology. Here are my favorite three:
1. The flatness problem: Why is the Universe’s geometry so flat?
2. The horizon problem: Why is the temperature of the cosmic microwave background radiation so incredibly homogeneous across the whole sky?
3. What caused the initial clustering of matter that evolved to become stars and galaxies in our Universe?
Next week we will explore these problems and how the inflaton just might solve them. As we will learn, such solutions come with problems of their own.